Abstract
Pediatric pulmonologists are frequently consulted on the child with “too many” respiratory infections. Considering the volume of air, particulates, chemicals, and potential airborne pathogens inspired with each breath, the question should actually be “Why don’t we get more infections?” The large surface area of the conducting airways and alveolar surfaces (>70 m 2 in the adult) that is available for these particles to deposit poses a great challenge for the lung defenses. The anatomy of the lung, lung products, cell receptors (and signaling mechanisms), and host cellular responses all contribute to the normal lung defense and repair. A defect in any of these defenses can result in an increased susceptibility to infection in the absence of “classic” immunodeficiency. In this chapter, we will review defenses that have evolved in the lung. In this chapter, we discuss three major categories of lung defense: intrinsic defenses (airway anatomy, physiology, secretory products that trap inhaled particles), innate defenses (various pattern recognition receptors [PRRs] and secreted proteins that provide first line antimicrobial and proinflammatory responses), and adaptive responses (cellular and antibody immunity). Finally, we will discuss cellular processes that help control inflammatory responses and allow repair.
Keywords
innate immunity, adaptive immunity, intrinsic immunity, cough, mucus, collectins, defensins, Toll-like receptors, alveolar macrophages, dendritic cells, mast cells, neutrophils, eosinophils, lymphocytes, T cells, B cells, apoptosis, efferocytosis, autophagy, interferons
Intrinsic Lung Defenses
Intrinsic defenses arise from the very structure of the conducting airways and the lung surfaces. The anatomy of the airways and airflow properties of the lung remove particles due to impaction or settling. Once they interact with the airway surface, these particles can elicit reflexes to remove them from the airway (sneezing or cough).
Aerodynamic Filtering
The anatomy of the upper and lower airways contributes to the defense of the lungs against infection. The nose provides the initial filtration and humidification of inspired air because of its structure and surface area. Very large particles are filtered by the nasal hairs, and particles larger than 10 µm impact on the surfaces of the turbinates and septum. Impaction is facilitated by the inertia of large particles that have a high linear velocity and do not easily change direction to follow air flow. The tonsils and adenoids are strategically located to deal with larger soluble particles by specific local defenses. If the tonsils and adenoids are markedly enlarged, nasal resistance increases. This potentially results in mouth breathing, bypassing the nasal anatomic defenses. Edema of the turbinates from viral infections or allergies may produce similar effects. Hydrophilic particles are enlarged by humidification of the inspired air, and this facilitates their impaction in the upper airways.
Particles between 2 and 10 µm are removed from the air flow by impaction on the walls of the branching airways beyond the nose, and by sedimentation. Sedimentation occurs because the increasing cross-sectional area of the conducting airways leads to a decrease in the linear air flow velocity such that gravitational forces may act on the particles. Smaller particles (as small as 0.2 µm) may not sediment at all and are exhaled. Particles in the size range of 0.2 to 2 µm generally penetrate the airways and can be deposited in the alveoli. Aerosol devices and metered-dose inhalers use particle size to direct deposition of the medication to different levels of the airway. Deposition of particles can trigger local inflammatory responses in the lung and airway reflexes.
Humidification
Warming and humidification begins at the nose and continues distally. Ultimately, inspired air is warmed to body temperature (37°C) and 100% relative humidity. Adequate hydration of airway mucus is essential to its proper functioning. The upper airway receives some humidity from convection of warm, humidified alveolar gas. During exhalation, the temperature drops and condensation forms on the upper airways, keeping their surfaces wet. At the isothermal saturation boundary (the point where air becomes body temperature) air is at 100% relative humidity that remains constant as it continues to move distally. This point typically occurs below the carina and will shift, depending on ambient temperature, humidity, and volume and rate of air exchange. It will also shift distally when the upper airway is bypassed, such as by tracheostomy. It never drops to the level of the respiratory bronchioles, so that at functional residual capacity (FRC), inspired gas is at body temperature and 100% humidity in the normal airway.
Airway Reflexes
Sneezing, bronchoconstriction, and coughing are airway reflexes that act as nonspecific host defenses. Sneezing is a forceful expulsion of air that is triggered by receptors in the nose and nasopharynx and is effective in clearing the upper pharynx and nose. The mechanisms are similar to coughing ( http://thekidshouldseethis.com/post/85595081892 ). Bronchoconstriction may prevent entry of particles into the distal airways by decreasing airway caliber and redirecting air flow away from the irritated airways. However, it also leads to increased pulmonary resistance, decreasing air velocity in the peripheral airways, and thus, increasing the likelihood of sedimentation. Coughing is a forceful expulsion of air from the lungs that is under both voluntary and involuntary control ( https://curiosity.com/paths/cough-grosser-than-sneeze-curiosity-worlds-dirtiest-man-discovery/#cough-grosser-than-sneeze-curiosity-worlds-dirtiest-man-discovery ). There are at least nine sensory receptors in the bronchopulmonary system located within the epithelium of the pharynx, larynx, trachea, and the bifurcations of the major bronchi. At least five of these are involved in the cough reflex, including the unmyelinated C fibers and the myelinated irritant receptors containing substance P and calcitonin-gene–related peptide (CGRP). These receptors can be stimulated by inflammatory mediators, chemical irritants, osmotic stimuli, and mechanical stimulation. Stimulation of respiratory afferents results in cognitive awareness of breathing and the urge to cough. The transient receptor potential (TRP) class of ion channels expressed on sensory neurons have recently been implicated in perception of noxious stimuli that lead to cough. The reflex of cough is initiated by stimulation of afferent fibers leading to the vagus nerve that connect to the cough center in the medulla oblongata and conscious awareness in the suprapontine brain. Efferent fibers transmit stimuli along the vagus nerve and spinal cord to the larynx, diaphragm, chest wall, and abdominal muscles to produce cough. After the cough, a feedback loop occurs to determine if the cough relieved the stimulation to cough. The cognitive urge to cough is an integration of respiratory afferents, respiratory motor system, affective state, attention, learning, and experience.
The Mechanics of Cough and Abnormalities in the Cough Reflex
Six phases of cough create high air flow velocities that produce the shear forces required to clear mucus from the airway walls and to promote its expulsion through the larynx.
- 1.
Irritation phase —cough triggered by stimulation of the irritant receptors in the tracheobronchial tree
- 2.
Inspiratory phase —initiated by a deep breath, which is usually 1.5 to 2 times the tidal volume. Air enters the airway distal to secretions. The length-tension relationships of the respiratory muscles are increased and optimized for contraction, elastic recoil potential increases, and the bronchi dilate.
- 3.
Glottic closure —necessary to build pressure for subsequent phases of cough
- 4.
Compression phase —begins with closure of the larynx and is followed by contraction of the intercostal muscles and abdominal musculature, which rapidly leads to increased intrathoracic pressure (up to 300 cm H 2 O or more in normal adults). This phase is fast, lasting approximately 200 msec.
- 5.
Expulsive phase —initiated when the glottis opens and high air flows are achieved. Following the glottic opening, the airways may collapse by as much as 80% in tracheal cross-sectional area, which further increases the velocity of exhaled gas (approaching 25,000 cm/sec the speed of sound) shearing mucus from the airway walls. The cough may be interrupted by a series of glottic closures, each with its own compressive and expulsive phases. These “spikes” in flow (flow transients) can improve cough effectiveness by increasing the shear forces. In patients with significant tracheomalacia, the equal pressure point may be quite proximal and limit cough effectiveness. Efforts to move this point distally (e.g., positive expiratory pressure device at the mouth) improve cough effectiveness.
- 6.
Relaxation phase —characterized by a decrease in intrathoracic pressure associated with relaxation of the intercostal and abdominal muscles.
Abnormalities of the cough mechanism can result in an ineffective cough. Cough receptors are not present in the alveoli or lung parenchyma, and therefore coughing can be absent in children with extensive alveolar disease or consolidation. A decrease in the sensitivity of the cough center occurs in obtunded patients and individuals under the influence of opiates. The efferent nerves can be affected by poliomyelitis or infantile botulism. The muscular force needed to generate the cough can be decreased by neuromuscular diseases such as spinal muscular atrophy or muscular dystrophy. Laryngeal disorders such as vocal cord paralysis or the presence of a tracheostomy tube prevent laryngeal closure so that the cough can lose its explosive quality. The use of cough-assist devices may be useful in enhancing airway clearance.
Innate Lung Defenses
Innate immunity provides immediate defense against a wide variety of pathogens or noxious substances without previous exposure to them. Innate immunity can remove infectious agents from the airway by binding and trapping them for removal (mucus and mucociliary clearance). Several PRRs recognize conserved patterns of molecular structures on pathogens (pathogen associated molecular patterns, PAMPs). Engagement of PRRs can activate cascades of intracellular signals producing inflammatory cytokines and chemokines that recruit and activate effector cells. Innate PRRs and receptor proteins facilitate cellular adhesion, and activation of soluble bactericidal factors such as complement. Secreted proteins contribute to innate lung defenses (e.g., the collectins and antibiotic proteins). There are resident innate immune cells that function as “first responders” to infection (dendritic cells [DC], macrophages, and mast cells). These “first responders” not only fight infection, but also produce mediators that recruit “early responders” from the circulation. These defenses protect the lung before adaptive immune processes can develop.
Mucus and Airway Surface Liquid
Mucus and mucociliary clearance are essential components of innate mechanical defenses in the lungs. Airway mucus is a complex network of mucins, electrolytes, enzymes, and protein defenses that immobilize, destroy, and remove noxious particles, foreign bodies, and invading microorganisms from the airway. It is the first line of innate defense in the lung.
The airways between the larynx and the respiratory bronchioles are lined by ciliated columnar epithelium and covered by an airway surface liquid (ASL) layer that is 5 to 100 µm thick. Mucus provides several important airway defense functions: It (1) provides a covering sheet that entraps particulate matter and microorganisms; (2) is a movable medium that can be propelled by cilia (the tips of cilia drive the mucus gel layer toward the oropharynx); (3) provides a waterproofing layer that acts to reduce fluid loss through the airways; (4) detoxifies noxious inhaled irritants; and (5) transports essential secreted substances such as enzymes, defensins, collectins, antiproteases, and immunoglobulins (discussed later).
ASL consists of two distinct layers: the low-viscosity periciliary gel layer (PCL) adjacent to the epithelial surface, and the mucus layer that floats on the PCL. The viscoelastic mucus layer is composed of secreted mucus glycoproteins (termed mucins) in addition to several secreted products. These mucins have high molecular weight, are long (0.5 to 20 µm), and are highly glycosylated and branched. MUC5AC and MUC5B are the major mucins in the mucus layer. Studies in gene knockout mice demonstrate that MUC5AC and MUC5B play distinct and important roles in airway homeostasis. The PCL is made up of the tethered surface mucins (MUC1 [MUCIN 1, cell surface associated], MUC4 [MUCIN 4, cell surface associated], MUC16 [MUCIN 16, cell surface associated], MUC20 [MUCIN 20, cell surface associated]) and is approximately the height of the cilia (7 µm). These tethered mucins provide a brushlike network that prevents penetration of inhaled particles and organisms to the epithelial surface. The PCL layer is crucial because it provides a low-viscosity fluid in which the cilia can beat rapidly (8 to 20 Hz), moving the more viscous mucus layer over it and shielding the epithelial surface from the substances trapped in the overlying mucus layer. The diversity of the carbohydrate side chains of the mucin macromolecules provides a library of carbohydrate sequences that can bind to an enormous repertoire of particles that land on the mucus layer.
The properties of this mucin gel are the product of the mucin and water contents, concentrations of monovalent and divalent ions, and the pH of the ASL. The water content of ASL is controlled by regulating levels of ion transport in the PCL layer via chloride channels (cystic fibrosis transmembrane conductance regulator [CFTR] and a calcium-activated [alternative] chloride channel), and the epithelial sodium channel (ENaC). These channels control Na + reabsorption and Cl − secretion by the respiratory epithelial cell via energy dependent mechanisms, with passive movement of water across the epithelial membrane in response to ion transport. There is active sensing of the mucus depth and viscosity by the underlying epithelium through cilia interaction with the overlying mucus. Increase in the “strain” on ciliary movement is transmitted through the cilial shaft, resulting in release of adenosine triphosphate (ATP) from the epithelial cell. The extracellular ATP activates purinoreceptors that inhibit sodium reabsorption through ENAC and accelerate Cl − secretion through CFTR and the calcium activated channel, resulting in a net fluid secretion onto the airway surface. The mucin concentration in the PCL is greater than that of the mucus layer, resulting in a higher osmotic pressure in the PCL (~500 Pa) compared to that of the mucus layer (~100 Pa). This difference in osmotic pressures makes the overlying mucus layer a “reservoir” for fluid in the airway and helps maintain the depth of the PCL layer. Inflammation can result in the deposition of large macromolecules such as DNA and polymerized actin from white cells, proteoglycans, biofilms, and other combinations of bacteria and inflammatory cells that can significantly increase the viscosity of the mucus. This increased viscosity of the overlying mucus layer increases its osmotic pressure, resulting in the equilibration of the osmotic pressures of the mucus and PCL layers and potential depletion of the PCL, eventually resulting in in the “collapse” of the ASL and loss of normal ciliary function.
Ciliary beat frequency and the effectiveness of the ciliary beat are the primary determinants of the mucus clearance rate. Ciliary motion is broken down into two steps: a unidirectional power stroke that sweeps forward and a recovery phase in which the cilia bend backward and extend to their starting position. Ciliary movement occurs within the same plane so that the movement is forward and backward without any lateral movement. Cilia also impart a vertical motion within the mucus layer, mixing particles, bacteria, and other pathogens into the mucus and facilitating their clearance. The lubricating effect of the mucous layer on the surface of the PCL allows the cilia to propel the overlying mucus layer with ease.
Disorders of the Mucociliary System
Primary ciliary dyskinesia (PCD) is a genetic disease associated with defective ciliary structure and function, with resultant chronic oto-sino-pulmonary disease, male infertility, and (in about half of patients) situs inversus. Cilia are complex structures composed of a highly organized array of microtubules and accessory elements, including inner and outer dynein arms, radial spokes, and nexin links (see Chapter 71 ). Disruption of this structural organization has been associated with ciliary immotility or dysmotility. Measurements of mucociliary clearance in patients with PCD have revealed no basal, cilia-dependent mucus clearance, although cough-dependent clearance is nearly normal. These patients maintain a nearly normal mucus clearance rate by increasing the dependence on cough. As a result, these patients exhibit milder airway disease than seen in cystic fibrosis (CF) and typically live into middle age and beyond. However, bronchiectasis and obstructive airway disease may occur in preschool children.
CF results from mutations in the respiratory epithelial inducible chloride channel (CFTR; see Chapter 49 , Chapter 50 , Chapter 51 , Chapter 52 , Chapter 53 ). In people with CF, mucus transport appears to be significantly altered by depletion of ASL and pH and electrolyte alterations that result from mutations in CFTR. Resulting defects in Na + and Cl − transport result in volume depletion on the airway surfaces which, in turn, depletes the PCL, disrupts normal ciliary activity, and inhibits mucociliary clearance.
Adhesion Proteins
Adhesion and migration of circulating inflammatory cells (or their progenitors) are integral to cell recruitment and activation response to injury ( Fig. 8.1 ). Three major families of adhesion molecules participate in these processes in the lung: the immunoglobulin superfamily, the integrins, and the selectins.

The immunoglobulin superfamily is a group of polypeptide genes characterized by the presence of one or more regions homologous to the basic structural unit of the immunoglobulin gene. Immunoglobulins and the T cell receptors (TCRs) are the only members of this family that undergo somatic diversification for antigen recognition (adaptive immunity). Several members of this family are required for cell-cell adhesion and migration of leukocytes from the vascular space into the airway. Moreover, they are required for adaptive immune responses (antigen presentation to T cells by DC and macrophages).
The integrins are a diverse family of molecules that are involved in cell-substrate or cell-cell interactions. The integrins are composed of two noncovalently associated polypeptide chains (α and β). In humans, there are 18 α and 8 β subunits that form 24 different heterodimers. Subgroups of this family are defined by common, shared β chains. β1 integrins function primarily by interacting with matrix components (collagen, laminin, fibronectin). Very Late Antigen 4 (VLA4, α4β1) is expressed by lymphocytes and monocytes. The β2 integrins (heterodimers of CD11 and CD18) are expressed almost exclusively on leukocytes and mediate cell-cell adhesion. Upon binding their extracellular ligands, integrins transmit signals that regulate various cellular functions (see Fig. 8.1 ). Integrins are critical for maintaining endothelial integrity, repair of damaged cells, and regulation of cell differentiation and proliferation. The importance of the β2 integrins in leukocyte recruitment is demonstrated in patients with leukocyte adhesion deficiency type 1 (LAD1), as characterized by recurrent bacterial skin and soft-tissue infections, diminished pus formation, and impaired wound healing.
Selectins are glycoproteins rich in O-linked and N-linked carbohydrates that mediate adhesion between leukocytes and vascular endothelium (see Fig. 8.1 ). The N-terminal ends of these molecules function as calcium-dependent carbohydrate lectin molecules. Three selectins have been identified: E-selectin (ELAM-1 [endothelial leukocyte adhesion molecule-1]), P-selectin, and L-selectin (LECAM-1 [leukocyte-endothelial cell adhesion molecule]). E-selectin is expressed on endothelial cells following stimulation by cytokines (such as IL-1 or tumor necrosis factor-α) and supports neutrophil adhesion. L-selectin is expressed on all leukocytes and is shed following adhesion to endothelial cells. P-selectin mediates cellular adhesion of neutrophils and monocytes to activated platelets and endothelial cells.
Pattern-Recognition Receptors in Lung Innate Immunity
The innate immune system utilizes a wide repertoire of conserved membrane-associated or soluble PRRs able to identify pathogens or cellular injury and initiate host defenses. These include the Toll-like receptors (TLR), nucleotide-binding leucine-rich repeat (LRR) containing receptors (NLR), retinoic acid-inducible gene 1 (RIG-1)-like receptors (RLR), C-type lectins (CTLs), and absent-in-melanoma (AIM)-like receptors (ALR). This pattern recognition receptor (PRR) recognizes patterns of sugars, ribonucleic acid (RNA), and deoxyribonucleic acid (DNA) as PAMPs that function as danger-associated molecular patterns (DAMPs). Through interactions with various cellular signaling cascades, they initiate and modulate inflammation, detect metabolic changes in the cell or cell death, and develop adaptive immune responses.
Toll-Like Receptors
The TLRs are a highly conserved family of membrane-associated PRR that have been identified in plants, insects (drosophila), and animals (mouse, human). They have two functional regions. The extracellular domain recognizes an array of microbial components: sugars, proteins, lipids, DNA motifs, and double-stranded RNA. The intracellular region contains a Toll/IL-1 receptor (TIR) domain that provides an intracellular scaffold interacting with a number of “adapter” proteins to initiate and integrate signaling cascades that result in cellular activation and the production of several cytokines and chemokines ( Fig. 8.2 ). Ten TLRs have been described in humans, and they are expressed by cell types involved in both innate immune defenses (such as macrophages, neutrophils, and airway epithelial cells) and adaptive immunity (macrophages, DC and lymphocytes). Ligands have been identified for all but TLR10 (see Fig. 8.2 ). The TLRs can be located in the plasma membrane (TLR1, TLR2, TLR4, TLR 5, TLR6, and TLR10) or intracellularly (TLR3, TLR7, TLR8, TLR9; see Fig. 8.2 ). The TLR proteins can associate as homodimers or as heterodimers of different TLR proteins thereby extending the target repertoire. The TLRs can also utilize a variety of coreceptor molecules. For example, TLR2 and TLR4 interact with MD-2 (soluble extracellular protein) and CD14 in binding to LPS. Several other PRR can cooperate with TLR in response to pathogens.

The TIR domains of the TLR interact with intracellular adaptor proteins (MYD88 or TIR-domain-containing adapter-inducing interferon-β [TRIF]+TRAM), resulting in activation of transcription factors that lead to the expression of cytokines or interferons (see Fig. 8.2 ). In addition, activation of TLR has been implicated in the upregulation of microbial killing mechanisms such as production of nitric oxide (NO). TLR signaling in DC drive T cell differentiation into T-helper type 1 (Th1) or T-helper type 2 (Th2) phenotypes. Thus, TLR activation interfaces both innate and adaptive immune responses.
C-Type Lectin Receptors
CTL receptors are membrane associated PRR that can bind either carbohydrate and noncarbohydrate (lipid or protein) ligands through a conserved CTL-like domain. They are expressed primarily on myeloid cells where they function in antifungal immunity.
Nodlike Receptors
The nodlike receptors (NLR) are a family of cytosolic PRR that participates in cellular inflammatory responses, apoptosis (programmed cell death), formation of phagosomes, and transcriptional activation of major histocompatibility complex proteins (MHC). These proteins are expressed in many cell types, including immune cells and epithelial cells. There are 22 members of the NLR family in humans. Their general structure includes a central nucleotide-binding oligomerization (NOD) domain and a C-terminal LRR domain. The N-terminal effector region varies between the different proteins, resulting in activation of diverse downstream signaling pathways. The NLR receptors participate in signal transduction, autophagy, transcriptional activation, and inflammasome assembly (see Fig. 8.2 ). NOD1 and NOD2 activate Rip2 kinase, leading to activation of NFκB. The NOD-like receptor pyrin domain (NLRP) family can lead to the assembly of inflammasomes, which consist of one or two NLRs, an adapter molecule ASC (inflammasome adaptor protein apoptosis-associated speck-like protein containing CARD), and caspase 1, that participate in caspase-1-mediated cell death and post translational production of mature IL-1β and related cytokines (including IL-18; see Fig. 8.2 ). NOD2 interacts with bacteria at the cell entry site and initiates autophagosome formation. CIITA (class II, MHC, transactivator) and NLRC5 (NLR family, CARD Domain Containing 5) are transactivators of the MHC in the nucleus.
RIG-Like Receptors
RIG-like receptors (RLRs) are cytoplasmic RNA helicases that recognize nonself RNA motifs. RIG-1 binds to 5′ tri and diphosphates present on short double stranded (ds) RNA, whereas MDA5 binds to long dsRNA molecules. Both helicases signal through the downstream adaptor MAVS (mitochondrial antiviral signaling) activating the IRF3/7-dependent production of type 1 interferons and NFκB-mediated production of proinflammatory cytokines.
Absent-in-Melanoma-Like Receptors
ALRs are a small group of PRR that can interact with ASC to form inflammasomes activating caspase 1 resulting in production of IL-1 and IL-18 (see Fig. 8.2 ). These PRR interact with both bacterial and viral derived dsDNA and are located in both the cytoplasm and nucleus.
Soluble Extracellular Pattern-Recognition Proteins
The soluble extracellular pattern-recognition proteins can be transfer from the bloodstream (complement, mannose binding lectin [MBL]) or secreted directly into the airway epithelium (surfactant proteins A and D) and provide innate defenses in the lung by carbohydrate recognition domains (CRDs). Several members of the collectins and ficolins activate complement.
Complement
Complement proteins play an important role in lung defenses. Complement components arrive in the lung both by extravasation due to vascular injury and leak and by local production by the respiratory epithelium and macrophages. Complement activation occurs by three pathways (classical, lectin or alternative) and contributes to host defense through several mechanisms:
- ▪
opsonization by deposition of complement components on the pathogen surface
- ▪
activation of phagocytic cells by the local release of chemotactic agents (e.g., C5a)
- ▪
lysis of pathogens through the activation of the late components C5, C6, C7, C8, and C9
- ▪
generation of anaphylatoxins (C3a and C5a) that cause the release of vasoactive mediators from mast cells
- ▪
generation of C3b and C4 on cell surfaces that bind to receptors on phagocytic cells.
Complement deficiency is associated with recurrent infections, glomerulonephritis, or collagen vascular diseases such as systemic lupus erythematosus.
Collectins
The collectins are a family of collagenous, calcium-dependent (C-type) lectins, including MBL (mannose-binding lectin) and surfactant-associated proteins A and D (SP-A and SP-D, respectively). They have a key role as a first line of innate defense against invading microorganisms in the airway. Both SP-A and SP-D are secreted into the air spaces by alveolar type II cells and nonciliated bronchiolar cells. Selective binding of collectins to specific complex carbohydrates is mediated by the CRD and requires calcium. Human SP-A is assembled as heterotrimers or homotrimers of two genetically different chain types and forms hexamers of these trimeric subunits (6 × 3 = 18 chains). SP-D is assembled as homotrimers that form tetramers (4 × 3 = 12 chains). This arrangement of subunits increases the binding affinity and specificity: multiple CRD domains can simultaneously bind to different ligands on a single polysaccharide chain, increasing the binding avidity of the complex. SP-A and SP-D interact with a variety of gram-negative and gram-positive organisms, fungi, and viruses including respiratory syncytial virus (RSV), Influenza A virus, and cytomegalovirus (CMV). Lung collectins can lead to opsonization through activation of complement and deposition of C3 (MBL) or can directly opsonize microorganisms (SP-A and SP-D), leading to phagocytosis and pathogen killing. SP-A binds to LPS and interacts with CD14 and TLR4, suggesting a role for SP-A in LPS-mediated cell responses. MBL is produced in the liver but is found in the lung at sites of inflammation. MBL is a serum protein, although small amounts of this protein have been found in lung secretions.
The ficolins are lectin recognition molecules that are structurally and functionally homologous to MBL. Three ficolins have been described. Ficolin-1 (M-ficolin) is expressed in the lung, monocytes, and spleen. Ficolin-2 (L-ficolin) is a serum protein expressed in the liver. Ficolin 3 (H-ficolin) is expressed in liver but also by bronchial and type II alveolar epithelial cells. They share the collagenous structure of MBL, but the CRD of MBL is replaced by a fibrinogen-like domain. They assemble in large multimeric structures of several hundred kilodaltons and recognize molecular patterns such as acetylated compounds and sugars. Similar to MBL, they activate complement through the lectin pathway.
Antimicrobial Peptides
Several intrinsic microbiocidal and microbiostatic proteins are found in human respiratory secretions, including lysozyme, lactoferrin, secretory leukoprotease inhibitor (SLPI), and neutrophil and epithelial defensins.
Lysozyme is the one of the most abundant antimicrobial proteins in the airways with concentrations estimated at 0.1 to 1 mg/mL, which is sufficient to kill important pulmonary pathogens such as S. aureus and P. aeruginosa. Lysozyme damages the walls of bacteria and fungi by hydrolyzing β1–4 glycosidic bonds between N-acetylmuramic acid and N-acetylglucosamine, structural components of bacterial peptidoglycan and fungal chitin. In humans, lysozyme is secreted predominantly by the serous cells of submucosal glands in the conducting airways, and, to a lesser extent, by airway epithelial cells and alveolar macrophages (AM). It is also a component of both phagocytic and secretory granules of neutrophils.
Lactoferrin is present in tears, saliva, and bronchial secretions. It is present in ASL at concentrations similar to lysozyme (0.1 to 1 mg/mL). It is produced by the submucosal glands and neutrophils and has activity against both gram-positive and gram-negative bacteria and Candida species. The antimicrobial actions of lactoferrin results from iron chelation (iron is required by many bacteria for optimal growth) or from destabilization of bacterial membranes.
SLPI and elafin were originally described as protease inhibitors. They have subsequently been shown to have a wider range of biologic activities. SLPI is an 11.9-kDA protein about 10-fold less abundant than lysozyme and lactoferrin in ASL. The N-terminal domain has modest activity against both gram-positive and gram-negative organisms. The C-terminal domain is an effective inhibitor of neutrophil elastase, cathepsin G, trypsin, chymotrypsin, tryptase, and chymase. Elafin/Trappin-2 is a 9.9-kDA protein that has a narrower spectrum of protease inhibition (only inhibiting neutrophil elastase and proteinase 3). In addition, the protease inhibitors exhibit antiinflammatory effects on NFκB activity and antimicrobial activity against a number of organisms, including Staphylococcus aureus and Pseudomonas aeruginosa. SLPI may also play a role in adaptive immunity through maintenance of mucosal tolerance.
Human defensins are antimicrobial peptides (3 to 5 kDa) found in BAL fluid from noninflamed lungs at a concentration of about 100 ng/mL, but at increased levels in inflamed airways. Four human alpha defensins, (human neutrophil defensins 1-4, human neutrophil peptide [HNP]-1, HNP-2, HNP-3, and HNP-4) are found in neutrophil azurophilic granules. Four beta-defensin molecules have been described (hBD-1, hBD-2, hBD-3, and hBD-4). hBD1 is expressed constitutively by epithelial cells, while hBD2 to hBD4 are inducible. In addition to their antimicrobial effects, defensins help recruit inflammatory cells and promote both innate and adaptive immune responses.
Cathelicidins are peptides produced by neutrophils and respiratory epithelial cells that have functions similar to those of defensins. The human cathelicidin (LL-37) displays LPS-binding activity and broad-spectrum microbiocidal activity.
Cellular Defenses: at the Crossroads of Innate and Adaptive Immunity
Our knowledge of the cellular constituents in the lung, their activation state, and their function has been considerably augmented by the flexible fiberoptic bronchoscopy and biopsy. Using bronchoalveolar lavage (BAL), the normal cellular constituents of the airway and alveolar space have been measured in both adults and children. In normal subjects, macrophages are the major cellular component of BAL, making up 81% to 95% of the cells; the remaining cells are lymphocytes, neutrophils, and eosinophils. The BAL cell number and percent composition vary greatly in disease states. Data obtained from BAL and biopsy studies provide direct evidence of the role of inflammatory cells, adhesion molecules, and cellular mediators in humans, confirming findings in cell culture and animal studies.
The Respiratory Epithelium
The respiratory epithelium is a functional interface between the pathogen and the innate or adaptive immune responses, making it a pivotal structure in respiratory physiology and pathology. The respiratory epithelium participates in lung immunity in many different ways. The epithelium provides physical barrier to infection, lining the respiratory tract from the nose to the alveoli with a wide range of cell types. Ciliated epithelial cells are important in propelling mucus up the airway, thereby removing particulate material. Ciliated cells line the respiratory tract down to the level of the respiratory bronchiole. Tracheobronchial glands and goblet cells are important sources of airway mucus, which serves to nonspecifically trap particulates. The respiratory epithelium also functions in the regulation of water and ion movement into the airway mucus. It secretes SP-A and SP-D, lysozyme, lactoferrin, and antimicrobial peptides (β-defensins and cathelicidins), and releases reactive oxygen and nitrogen species to kill invading pathogens. The respiratory epithelium can produce a number of bioactive cytokines that recruit and activate inflammatory cells in the lung (interferon [IFN]-α, IFN-γ, IL-1, TNF-α, IL-8, RANTES [Regulated on Activation, Normal T Cell Expressed and Secreted]). The respiratory epithelium influences adaptive immunity through cytokine release: fostering a Th2-like response through release of IL-25, IL-33, and TSLP (thymic stromal lymphopoietin). The epithelium promotes recruitment and activation of DC and lymphocytes (CCL20) and mast cells (stem cell factor [SCF], IL-33, TSLP release). The respiratory epithelium also acts as its own reservoir for injury repair. Finally, the epithelium promotes remodeling through release of transforming growth factor-beta (TGF-β). It is therefore a key modulator of lung defense, lung injury, and repair.
Resident Cell Defenses: At the Interface of Innate and Adaptive Immunity
Three major groups of inflammatory cells reside primarily within the lung parenchyma itself: DC, macrophages, and mast cells. The cells (particularly the macrophages and DC) are capable of migration; however, they reside primarily within the lung itself. Other inflammatory cells are recruited from the circulation in their mature form (neutrophils, eosinophils, and lymphocytes) and become activated in the lung. The pulmonary DC and macrophages participate in both innate and adaptive immune responses in the lung.
Dendritic Cells.
DC are the primary resident antigen-presenting cells in the lung and airway. Activation of DC in response to innate stimuli is essential to initiate the adaptive response to antigens. In these processes, the phenotype and function of DC play an important role in initiating adaptive immune responses (tolerance, memory, and polarized Th1 and Th2 differentiation). Antigen presentation to T cells is actively and tightly regulated in vivo by soluble factors produced by mature tissue macrophages. Therefore, the phenotype of the DC, the response to innate stimuli and cytokines produced by macrophages, and the lung microenvironment play important roles in determining host adaptive responses.
In humans, three different subsets of DC (2 “myeloid” and one “plasmacytoid”) have been described: mDC1 (expressing BDCA1/CD1c), mDC2 (BDCA3/CD141), and pDC (BDCA2/CD123). The origin of human DC is not completely understood, but they appear to arise from common granulocyte/macrophage and multilymphoid progenitors in the bone marrow. DCs have a rapid half-life in the conducting airways (about 2 days), whereas more distal lung DCs have a slower turnover rate. mDC1 cells have been associated with induction of CD8+ and CD4+ Th1 responses, whereas mDC2 cells are associated with CD4+Th2 and Th17 responses, although there is some overlap. pDC have been ascribed an antiviral role, although in the steady state, their main role appears to be induction of tolerance. mDC are associated with the airways and appear to “sample” the airway by extending dendrites through the airway epithelial layer. pDC locate mainly in the alveolar interstitium. However, they have the ability to migrate to inflammatory foci, where they can take up and process antigen, migrate through the lymphatics to the draining lymph nodes, and associate with T cell–rich areas to initiate adaptive immune responses.
Macrophages.
The pulmonary macrophages are central regulators of airway inflammation. They exhibit mobility, phagocytosis, receptor expression for signal recognition, and production and release of a number of bioactive mediators. In the lung, they are capable of scavenging particulates, removing macromolecules, killing microorganisms, recruiting and activating other inflammatory cells, maintaining and repairing injured tissue, removing apoptotic cells, and modulating normal lung physiology. Many of these functions are “turned off” in the resting state but are upregulated with macrophage activation. Therefore, regulation of macrophage activation and turnover is important in determining pulmonary health or disease. Macrophages can cooperate with other cell types (DC, neutrophils, lymphocytes, fibroblasts) by direct cell–cell interactions and by cytokine signals to orchestrate development of cell-mediated immunity and humoral immunity. ( https://www.youtube.com/watch?v=LwLYGTS_3EI ). Macrophages are more abundant in the distal respiratory tract, particularly the alveolus, than in the tracheobronchial tree. The macrophage population is usually constant in size, but this is the result of a dynamic steady state of cellular recruitment, cell division, and cell turnover. At least two types of macrophages reside in the lungs: interstitial macrophages (IM) and AM. The macrophages populating the airways above the alveolus are thought to arise from those in the alveolus. IM are in the interstitial space between alveolae where they interact with DC and interstitial lymphocytes. They promote tolerance and prevent Th2 mediated airway inflammation. The IM are thought to be derived from circulating precursor peripheral blood cells of the monocyte/macrophage lineage.
AM, along with the epithelium, are the first line of defense against infection. They are mobile, traveling between the alveoli and sampling the alveolar surface. They are phagocytic and express numerous PRR that trigger expression of cytokines, chemokines, and other reactive products (lipids, reactive oxygen, and nitrogen species) that are crucial in control of infection through the innate immune system and balance of proinflammatory and antiinflammatory pathways in the lung. The AM are classified according to their activation status as M1 or M2. M1 macrophages (also termed classically activated macrophages) respond to IFN-γ, LPS, and TNFα to produce proinflammatory cytokines leading to the destruction of intracellular pathogens and promoting a Th1 environment. M2 (alternatively activated macrophages) are characterized by their participation in Th2 responses. The switch to M1 or M2 phenotype is associated with the specific transcriptional response to the local microenvironment.
AM are thought to arise from yolk sac macrophages or fetal liver monocytes that enter the lung during embryonic development and colonize the alveoli in the first days after birth. AM mature under the influence of local production of CSF-2 (GM-CSF). This population of early progenitors populates the lung and self-maintains throughout the host’s lifetime. Under inflammatory conditions, some AM may arise from mononuclear cells, but these more resemble the IM in function. The turnover time for AM has been calculated to be 21 to 28 days in animal models. At the end of this time, they exit from the lungs up the mucociliary escalator, although the mechanisms for this efflux are still unknown.
AM produce a number of reactive oxygen metabolites and nitrogen metabolites and a large diverse array of enzymes (acid hydrolases, neutral hydrolases, and lysozymes) capable of killing bacteria. AM also produce cytokines and chemotactic factors that play a central role in phagocyte recruitment and activation, wound healing, fibrosis, and modulation of actions of other innate and adaptive immune responses in the lung.
Mast Cells.
Mast cells are the major effector cells of allergic reactions. They are distributed in tissues throughout the body in close proximity to blood vessels and do not circulate in the blood. It has been postulated that they play roles in host defense against parasites, wound healing, immunoregulation, and tumor angiogenesis. Mast cells originate in the bone marrow from granulocyte and monocyte pluripotent CD34+ and CD117+ stem cells. Homing to specific tissues is driven by active local recruitment. Growth and survival of mast cells requires stem cell factor (SCF) production by structural cells (epithelial cells, fibroblasts, endothelial cells, smooth muscle cells). SCF promotes proliferation and differentiation, chemotaxis, and suppression of apoptosis that promotes mast cell growth, recruitment, and survival. Mature differentiated mast cells are capable of proliferation. Therefore, tissue-specific increases in mast cell numbers can occur by either recruitment or local proliferation.
Mast cells can express a wide variety of receptors that allow them to detect intracellular and extracellular signals and interact with other inflammatory cells and with the extracellular matrix. Ligand binding and activation of the FcεRI results in release of a number of bioactive products responsible for allergic reactions.
Mast cells participate in antigen presentation and interactions with other immune cells via costimulatory molecules or secreted products that can enhance or suppress the development of innate or adaptive immune functions. There is strong evidence that mast cells play significant roles in atopic and nonatopic asthma, pulmonary fibrosis, pulmonary hypertension, and may contribute to acute respiratory distress syndrome (ARDS). Persistent activation of mast cells can also exacerbate Th2-dependent or autoimmune disorders.
Recruited Cellular Defenses
All recruited pulmonary inflammatory cells are initially derived from bone marrow precursors and arrive from the pulmonary circulation in their mature, active forms. In the process of recruitment, these cells move from a “quiescent” resting state to a state in which they are fully “activated” or “primed” for further cellular activities.
Neutrophils.
Neutrophils are characterized by their multilobed nucleus and distinctive cytoplasmic granules that contain an arsenal of enzymes and proteins. Neutrophils constitute about half the circulating white blood cell population, and their primary function is phagocytosis and killing of invading pathogens. In order for the neutrophil to accomplish this, it must respond to signals in the area of injury, adhere and transmigrate through the vascular endothelium, migrate to the area of infection, recognize the pathogen, phagocytose, and kill it. Interruption of any of these steps will leave the host susceptible to infections. Patients with leukocyte adherence deficiency (LAD) are unable to recruit neutrophils into sites of inflammation, and, as a result, they sustain recurrent, life-threatening infections. In chronic granulomatous disease (CGD), the neutrophils lack components of the nicotinamide adenine dinucleotide phosphate hydrogen (NADPH) oxidase system, and affected neutrophils that have engulfed organisms cannot efficiently kill them. The processes of neutrophil recruitment and activation, followed by neutrophil removal (cell death, or apoptosis) after the resolution of the infectious process or injury, are closely regulated at several levels. Disruption of these regulatory processes can lead to acute or ongoing lung injury.
Neutrophils are short-lived cells; their life span from stem cell differentiation to removal in the tissues is 12 to 14 days. CD34+ myeloid progenitor cells in the bone marrow produce myeloblasts, which then differentiate through several recognizable morphologic stages into mature, nondividing polymorphonuclear neutrophils. It normally takes about 14 days for the neutrophil precursor to mature and be released into the blood. Once in the circulation, neutrophil half-life is quite short (~6 to 7 hours). The pulmonary capillary bed is unique in its ability to “concentrate” neutrophils. The term margination has been proposed to describe this increased concentration of neutrophils in noninflamed lungs. Margination is proposed to result from discordance between the diameter of neutrophils (6 to 8 µm) and the capillary segments (2 to 15 µm), increasing the pulmonary capillary transit time. This is postulated to allow neutrophils time to sense and respond to the presence of inflammatory processes. In response to inflammatory stimuli, neutrophils accumulate in preparation for migration (see Fig. 8.1 ). In much of the systemic circulation, neutrophil sequestration occurs in postcapillary venules in the form of rolling and is mediated by selectins. In the pulmonary capillary, inflammatory mediators alter the mechanical properties of the neutrophils, resulting in changes in deformability and “stiffening” that reduces the neutrophil deformability, lengthens the capillary transit time, or stops the movement of the neutrophil altogether at the sites of inflammation. Once sequestration has occurred, the neutrophils can adhere via selectin or CD11/CD18-intercellular adhesion molecule-1 (ICAM-1) and migrate across the vascular endothelium (see Fig. 8.1 ). During migration into the lung, the neutrophils become activated, resulting in a change in surface receptors compared to blood neutrophils. The migration and activation of neutrophils requires a number of products of the endothelium, epithelium, and AM including IL-1β, TNF-α, IL-6, IL-8/KC, macrophage inflammatory protein (MIP)-1α, MIP-1β, MIP-2, CCL2, G-CSF, and LTB4. Once the neutrophil has migrated into the tissue, its primary purpose is to recognize, ingest, and destroy pathogens.
Similar to the resident innate cellular defenses, neutrophils express numerous PRR and respond by production of reactive oxygen species and secretion of azurophilic granule contents (myeloperoxidase, elastase, defensins), specific granule contents (lactoferrin, cathelicidins), gelatinase granule contents (lysozyme) and the formation of neutrophil extracellular traps (NETs). NETs are weblike structures composed of dsDNA, histones, antimicrobial peptides, and proteases ejected by neutrophils to ensnare microbes in a sticky matrix of extracellular chromatin and antimicrobial peptides, making the microbes more vulnerable to phagocytosis and clearance. ( https://www.youtube.com/watch?v=TIFmtnSdolM ). Over-exuberant neutrophil activity can contribute to ARDS and to tissue destruction in autoimmune diseases. There are data supporting a role for neutrophils in wound healing and homeostasis.
Eosinophils.
Eosinophils are usually the second largest group of granulocytic cells in the circulation. They can be morphologically distinguished from neutrophils by their ability to be stained by negatively charged dyes, such as eosin. They are 8 µm in diameter and typically have two nuclear lobes. Eosinophils arise from bone marrow precursor cells under the influence of a number of cytokines including IL-3, IL-4, IL-5, IL-14, and GM-CSF. IL-4 and IL-13 stimulate IgE production and promote eosinophil recruitment by increasing expression of eotaxin and endothelial cell vascular cell adhesion molecule 1 (VCAM1). IL-5 mediates eosinophil production and egress from the bone marrow, eosinophil activation, and survival. The migration of eosinophils from the bone marrow takes about 3.5 days after DNA synthesis has completed. In the blood, the half-time of an eosinophil is 18 hours. Similar to neutrophils, there appears to be a marginated pool of eosinophils, which are in dynamic equilibrium with the circulating pool. Eosinophils marginate and emigrate into the tissues through postcapillary venules. Eosinophils, but not neutrophils, adhere to the ligand vascular cell adhesion molecule (VCAM)-1 on the endothelial surface and once adherent, can be attracted by a specific array of chemotactic agents into the lung. Eosinophils express the receptor CCR3 that binds eosinophil specific chemokines including eotaxin (CCL11) eotaxin-2, monocyte chemoattractant protein (MCP)-3, MCP-4, and RANTES (CCL5). Eosinophil recruitment into the lungs occurs under the influence of Th2 mediated inflammation. Once present in the lungs, eosinophils degranulate and release a number of granular components including major basic protein (MBP), eosinophil peroxidase (EPO), eosinophil cationic protein (ECP), and eosinophil-derived neurotoxin (EDN); as well as cytokines and chemokines. Some of these cytokines promote eosinophil survival and activation. In addition, eosinophils can undergo extracellular trap cell death (ETosis) and release extracellular traps similar to those released by neutrophils. The eosinophil traps bind eosinophil granules as intact structures, unlike the traps formed by neutrophils. Collectively, these eosinophil products have potent activity that implicates the eosinophil in the control of parasitic diseases. However, these proteins also have activities that are associated with the pathogenesis of a number of pulmonary disorders. Asthma is by far and away the most common eosinophil-associated lung disease in children. The pathophysiology of asthma results from contributions from a number of inflammatory cells including eosinophils, mast cells, neutrophils, and T lymphocytes. In addition to asthma, eosinophils contribute to the development of a number of eosinophilic pneumonias (see Chapter 65 ). These can be roughly divided into primary eosinophilic pneumonias (eosinophilic granulomatosis with polyangiitis, idiopathic acute eosinophilic pneumonia, idiopathic chronic eosinophilic pneumonia) and secondary pneumonias (pneumonia in response to parasitic worms, allergic bronchopulmonary aspergillosis, drug reactions).
Innate Lymphocytes: Innate Lymphocyte Cells and Natural Killer Cells.
Over the past decade, there has been strong interest in newly described subsets of lymphocytes referred to as innate lymphocyte cells (ILCs). There are currently three groups of ILC (ILC1, 2, 3) characterized by cytokine products and transcription requirements for maturation. They display a wide variety of effector functions that contribute to defense against pathogens, to lymph node development, and to tissue remodeling. Given the ongoing research to further characterize ILCs, a full discussion is beyond the scope of this chapter, and the focus will be directed to NK cells, which comprise part of the ILC1 subset.
Natural killer (NK) cells are large granular lymphocytes that are key players in early immunity along with other cells involved in the innate immune system, including neutrophils, monocytes, and mast cells. At first, they were recognized in having cytotoxicity against tumor cells and virus-infected cells. Over the past four decades, their role has been well established in a variety of functions, including cytokine production and interaction with other immune cells to promote immune system homeostasis. Although they were traditionally considered part of the innate immunity, there is a significant amount of evidence to support NK cells having a role in adaptive immunity (below). NK cells have been shown to respond to their local environment by indirectly modulating T cell effector and antigen presenting cell function, and forming immunologic memory, which are hallmarks of adaptive immunity.
Derived from the bone marrow, NK cells are characterized by CD56. Peripheral NK cells can be further divided into two subsets; CD56 bright and CD56 dim . CD56 bright , which are more predominant in serum, are known to be more responsive to soluble factors, while CD56 dim , which are more abundant in tissue, respond better to cell surface ligands. NK cells lack a TCR/CD3 complex, and represent approximately 2% to 18% of the total lymphocyte population circulating in the blood. NK cells can initiate natural cytotoxicity via release of perforin and granzyme granules. Perforin results in pore formation on the target cell, which allows entrance of granzyme that induces apoptosis. In addition, their effector functions (enhancing cytotoxicity, inducing cytokine secretion, modulating immune homeostasis) are largely exerted by the wide and diverse number of activating and inhibitory receptors expressed on the NK cell surface. Binding of antibody to CD16 (FcγR III) on the NK cell surface induces antibody-dependent cell-mediated cytotoxicity. The natural killer cell receptors (NCR), can bind to a stressed cell, caused by infection or cellular damage. These stressed cells express ligands that the NK cell can bind to and subsequently induce cell lysis. There are several receptors that bind proinflammatory cytokines, which stimulate NK cells to respond to infection, proliferate, and promote further cytokine secretion.
Inhibitory receptors will downregulate NK cell function. Killer cell immunoglobulin like receptors (KIR) are MHC class I inhibitory receptors, and when bound to self MHC molecules, they ensure tolerance to self, preventing damage to healthy cells.
Recently, there has been a better understanding of the tissue bound NK cells, particularly in the mucosa, including uterus, and lung. These “nonconventional” NK cells are unique NK cells with varying phenotypic properties. With their proximity to the epithelia, they express unique receptors to enhance protection against the external environment as well as to provide homeostasis to prevent an exuberant inflammatory response.
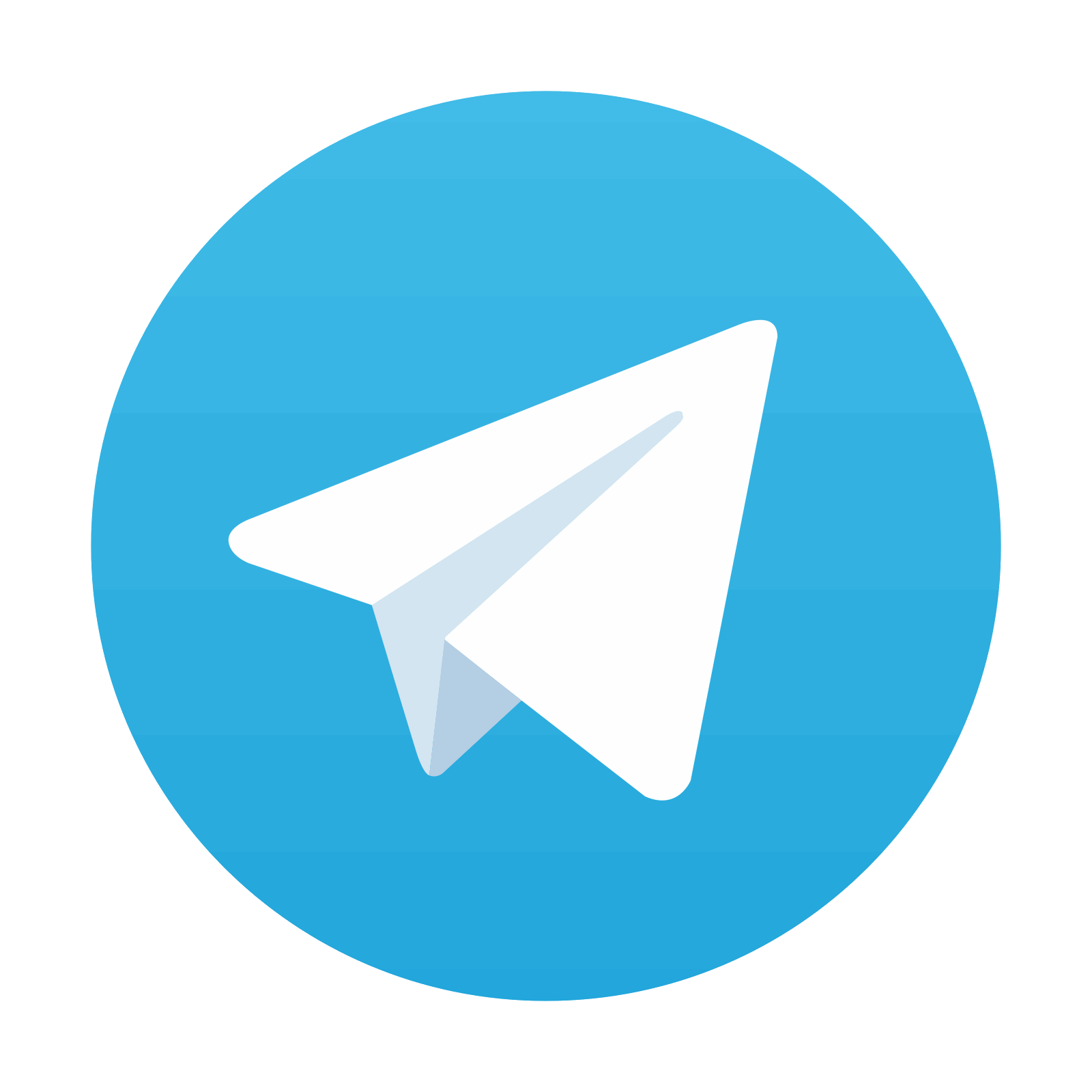
Stay updated, free articles. Join our Telegram channel

Full access? Get Clinical Tree
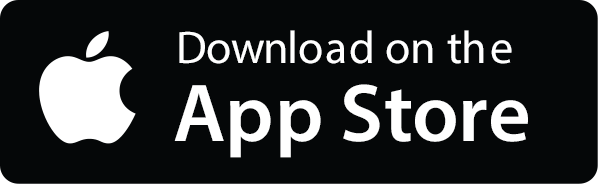
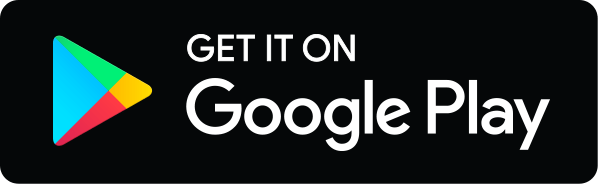