Lung Cancer and Its Microenvironment
Marie Wislez
Jacques Cadranel
Jonathan M. Kurie
The association between inflammation and cancer was identified in the 19th century. Initially, it was believed that leukocyte infiltrates in tumors represented an attempt by the host to eradicate malignant cells. It was later demonstrated that several chronic inflammatory conditions, such as inflammatory bowel disease, Helicobacter pylori infection, hepatitis B or C infection, and prostatitis, predisposed people to cancer of the colon, stomach, liver, and prostate, respectively. In addition, malignant tissues that contain inflammatory cells such as macrophages in breast cancer or neutrophils and mast cells in lung cancer are associated with an unfavorable outcome.
Lung cancer risk is clearly enhanced by cigarette smoking, and chronic inflammation associated with chronic obstructive pulmonary disease probably enhances this risk, although an increase is difficult to demonstrate. Some lung cancers that occur in association with scars could have no relationship with smoking habits. In patients with idiopathic fibrosis, lung cancer incidence is much higher than in the general population.1 Oncogene activation is often associated with inflammatory response. For example, scar-associated cancers seem to more often have KRAS (codon 12) mutations. Further, a transgenic mouse model of lung cancer generated by KRAS activation showed a robust inflammatory response compared to the wildtype mice.2,3 Lastly, in several mouse models, this inflammatory response has been demonstrated to be not only associated with but also required for tumor initiation or growth.4,5
The tumor microenvironment is composed of structural (extracellular matrix [ECM]), soluble (growth factors, chemokines, cytokines, proteases, and hormones, among others), and cellular components (tumor cells, fibroblasts, inflammatory cells, vascular and lymphatic endothelial cells, and vascular smooth muscle cells and pericytic cells, among others). Characterization of the inflammatory cells within tumors has revealed both the adaptive and innate arms of the immune response. For example, dendritic cells (DCs) present tumor antigens to T lymphocytes (CD4+, CD8+, and natural killer [NK]), promoting an antitumor cytotoxic T-cell response. This response is negated by a population of immature myeloid cells called myeloid-derived suppressor cells that promotes the development of FOXP3+ CD4+ T cells or Tregs, which suppress the antitumor cytotoxic T-cell response and induce polarized differentiation of monocytes into tumor-associated macrophages (TAMs or M2 macrophages). TAMs, vascular endothelial cells, and fibroblasts within the tumor stroma secrete a number of growth factors and chemokines that promote tumorigenesis. Thus, conflicting immunologic forces fight for supremacy in the tumor microenvironment. This chapter will deal with recent research on the tumorigenic and antitumorigenic effects of the immune system on the lung. The latter was discussed fully in a recent review.6
ANGIOGENESIS
Angiogenesis is the growth of the new blood vessels, necessary for cancerous tumors to keep growing and spreading (see Chapter 8). Many proteins and other smaller molecules have been identified as angiogenic, particularly vascular endothelial growth factor (VEGF), basic fibroblast growth factor (bFGF), and CXC chemokines, among others. The binding of these molecules to their appropriate receptor activates a series of relay proteins that transmits a signal into the nucleus of the endothelial cells. The nuclear signal ultimately prompts a group of genes to make products needed for new endothelial cell growth. First, the activated endothelial cells produce matrix metalloproteinases (MMPs), a class of degradative enzymes that break down the extracellular matrix, thus permitting the migration of endothelial cells that had been tethered to the matrix. As they migrate into the surrounding tissues, activated endothelial cells begin to divide. Soon they organize into hollow tubes that evolve gradually into a mature network of blood vessels. Cancer cells originating in a primary tumor can spread to another organ and form metastases that can remain dormant for years. The induction of this vasculature in primary tumor or in metastases, termed angiogenic switch, can occur at various stages of tumor progression, depending on the tumor
type and the environment (see Chapter 8).7 In many studies, angiogenic switch has been reported to be closely associated with malignant transition. Cancer cells themselves could release molecules to activate this process, as could cells from the tumor microenvironment. This review will describe effects of the cells from the tumor microenvironment on angiogenesis.
type and the environment (see Chapter 8).7 In many studies, angiogenic switch has been reported to be closely associated with malignant transition. Cancer cells themselves could release molecules to activate this process, as could cells from the tumor microenvironment. This review will describe effects of the cells from the tumor microenvironment on angiogenesis.
FIBROBLASTS
Normal stroma contains fibroblasts in association with physio logical extracellular matrix. Reactive stroma is associated with an increased number of fibroblasts, enhanced capillary density, and type I collagen and fibrin deposition. In chickens that are cancer-prone because they have been infected with Rous sarcoma virus, wounding leads to invasive carcinoma, demonstrating that reactive stroma provides oncogenic signals that facilitate tumorigenesis.8 Fibroblasts are associated with cancer cells (tumor-associated fibroblasts [TAFs], carcinoma-associated fibroblasts [CAFs]) at all stages of cancer progression. The growth factors, chemokines, and extracellular matrix—these fibroblasts produce facilitate angiogenic recruitment of endothelial cells and pericytes. They are phenotypically and functionally distinct from fibroblasts that are not in the tumor microenvironment. The modified phenotype they acquire is similar to that of fibroblasts associated with wound healing. Smooth muscle differentiation (myofibroblasts) is prominent in stromal cells of malignant breast tissue but rarely seen in normal breast tissue.9 The signals that mediate the transition of normal fibroblasts into TAF or CAF are not fully understood, but transfor ming growth factor-β (TGF-β), platelet-derived growth factor (PDGF), and fibroblast growth factor 2 (FGF2) are the main mediators to induce fibroblast activation. TGF-β induces the acquisition of activated phenotype of fibroblasts in culture10 and has been shown to be correlated with desmoplastic reaction and poor prognosis in breast cancer.11 PDGF induces the proliferation of fibroblasts and has been shown to be associated with cancer progression in breast cancer.12 FGF2 also stimulates proliferation of fibroblasts and is also recognized for its potential to induce angiogenesis.13
The fact that fibroblasts contribute to tumor initiation, growth, and metastasis have been demonstrated by both in vivo and in vitro study.14 Whereas normal fibroblasts are required to maintain epithelial homeostasis, CAFs probably initiate and promote tumorigenic alterations in epithelial cells. Fibroblasts cultured from malignant tumors have stimulatory effects on breast tumor cell lines, whereas fibroblasts cultured from normal tissue are inhibitory.15 If CAFs are coinoculated with prostate, breast, or bladder tumor cell lines into nude mice, tumor latency is shortened and tumor growth increased, 16 whereas normal fibroblasts do not have this effect. Increased cell proliferation and angiogenesis also result. Lastly, fibroblasts could promote metastasis by secreting growth factors that create a niche that promotes the growth of cancer cells at distant sites.17
CAFs could also modulate the immune response. CAFs isolated from primary non-small cell lung cancers (NSCLCs) were able to enhance or suppress tumor-associated T-cell function.18
The epithelial-to-mesenchymal transition (EMT) might be an additional source of fibroblast-like cells (with an altered genome). In EMT, epithelial cells lose cell-cell contacts and acquire mesenchymal properties. Cancer cells undergoing EMT develop invasive and migratory abilities and express EMT markers (E-Cadherin, Vimentin) that have been shown to be markers of tumor progression.19,20,21 This phenotype has also been shown to be associated with resistance to certain therapies such as epidermal growth factor receptor (EGFR) tyrosine kinase inhibitor (TKI) in NSCLC.21,22
MACROPHAGES
Most solid tumors are abundantly populated with TAMs. These cells can compromise clinical outcome. Clinical stu dies have shown a correlation between TAM density and poor prognosis for several types of cancer, an association that is particularly strong for breast, prostate, ovarian, and cervical cancers.23 For NSCLC, TAM density correlated significantly and negatively with overall survival or relapse-free survival in two of three published studies.24,25,26
Macrophages are recruited to tumors by a wide variety of growth factors—granulocyte colony-stimulating factor (G-CSF), granulocyte-monocyte colony-stimulating factor (GM-CSF), macrophage-stimulating protein (MSP), VEGF, TGF-β—and chemokines, which include CC chemokines, monocyte chemoattractant protein family, macrophage inflammatory protein-1 (MIP-1), and macrophage migration inhibitory factor (MIF).27
Tumor-derived molecules probably influence TAM phenotype. Exposure to IL-4 and IL-10 in tumors may induce TAMs to develop into M2 macrophages, which are characterized by poor antigen-presenting capacity and production of factors that suppress T-cell proliferation and activity and induce angiogenesis, whereas M1 macrophages are efficient immune cells.28
Macrophages induce angiogenesis. Correlations between number of macrophages and microvessel count have been observed for many tumor types including lung cancer.24,25,29 Macrophage infiltration into tumor is not homogeneous: studies using hypoxic markers have shown that TAMs accumulate in hypoxic and necrotic areas. Hypoxia induces synthesis of macrophage chemoattractants such as VEGF by upregulating hypoxia-inducible factor (HIF), which recruits and immobilizes macrophages in such areas.30 Here, these cells synthesize angiogenic regulators, which results in formation of new blood vessels. These regulators are angiogenic factors (VEGF, PDGF, IL-8) and angiogenesis-modulating enzymes (MMPs and cyclooxygenase-2 [COX-2]). In vitro studies, based on coculture experiences, showed that exposure of macrophage to tumor cells increases synthesis of angiogenic factors.25,31,32 In transgenic
mice susceptible to mammary cancer (PyMT mice), malignant transition was demonstrated to be regulated by infiltrated macrophages in primary mammary tumors. Inhibition of macrophage recruitment into the tumors delayed the angiogenic switch and malignant progression, while genetic restoration of the macrophage population rescued angiogenesis.33
mice susceptible to mammary cancer (PyMT mice), malignant transition was demonstrated to be regulated by infiltrated macrophages in primary mammary tumors. Inhibition of macrophage recruitment into the tumors delayed the angiogenic switch and malignant progression, while genetic restoration of the macrophage population rescued angiogenesis.33
Macrophages have been shown to stimulate proliferation of tumor cells. In K-rasLA1 mice, which develop lung adenocarcinoma through somatic activation of a K- ras allele, intraepithelial and airspace macrophage infiltration is observed beginning at the earliest stage of neoplasia and increasing with malignant progression.3 In this model, inhibition of malignant progression by a targeted treatment directed against the mTOR pathway was accompanied by macrophage loss. Conditioned media from primary cultures of macrophages stimulated the proliferation of lung tumor cells, which was consistent with previous reports demonstrating a stimulatory effect of alveolar macrophages on the proliferation of normal and distal airway epithelial cells in other animal models.34,35,36 In a transgenic mouse model of preneoplastic progression in the mammary gland, conditional depletion of macrophages inhibited epithelial cell proliferation and lateral budding.37
Macrophages are involved in invasion and metastasis. In the PyMT mouse model of breast cancer progression, leukocytic infiltrates were present in areas of basement membrane breakdown38 suggesting their involvement in tumor invasion; macrophage depletion resulted in reduced formation of lung metastases.4 In coculture experiments, interaction between macrophages and tumor cells facilitated invasion of tumor cells into a collagen matrix.39 In a chemotaxis-based in vivo invasion assay, a paracrine loop involving macrophages and tumor cells was essential for motility and invasion of tumor cells in mammary tumor.40 Colony-stimulating factor-1 (CSF-1) secreted by carcinoma cells leads to the activation of macrophages to secrete EGFR ligands, leading to stimulation of carcinoma cell movement.40
NEUTROPHILS
Neutrophil infiltration has been described in NSCLC, and particularly in the bronchioloalveolar subtype.41,42 Recent studies support the fact that this could be induced by Ras activation, one of the most common oncogenic events in pulmonary ade nocarcinoma.5 Neutrophils could be recruited to tumors by CXC chemokines with an N-terminal Glu-Leu-Arg (ELR) motif. These chemokines are also autocrine growth factors for certain types of cancer cells.43,44,45 Mutations in the proto-oncogene KRAS occur in 10% to 30% of lung adenocarcinomas, 46 and expression of mutant KRAS in the alveolar epithelium leads to the development of lung adenocarcinoma in mice.45,46,47,48,49,50 In addition to its role in the transformation of alveolar epithelial cells, the presence of KRAS mutations is a predictor of shorter survival in NSCLC patients51 and of resistance to therapy.52 Sparmann et al.5 demonstrated that the CXC chemokine CXCL8 (interleukin-8) is a transcriptional target of Ras signaling and is required for the initiation of tumor-associated inflammation and neovascularization in xenograft models. In this model, neutralization of CXCL8 in RasV12-expressing subcutaneous tumors attenuates neoplastic growth; CXCL8 inhibition does not affect tumor cell proliferation but leads to an increase in tumor cell death and an impairment of tumor vascularization coincident with an impairment of stromal infiltration of neutrophils. In the heterotopic and orthotopic Lewis lung cancer models, tumor growth is associated with enhanced neovascularization, neutrophil inflammation, and expression of CXC chemokines. Neutralization of CXC chemokine receptor decreases tumor size and increases tumor necrosis.53
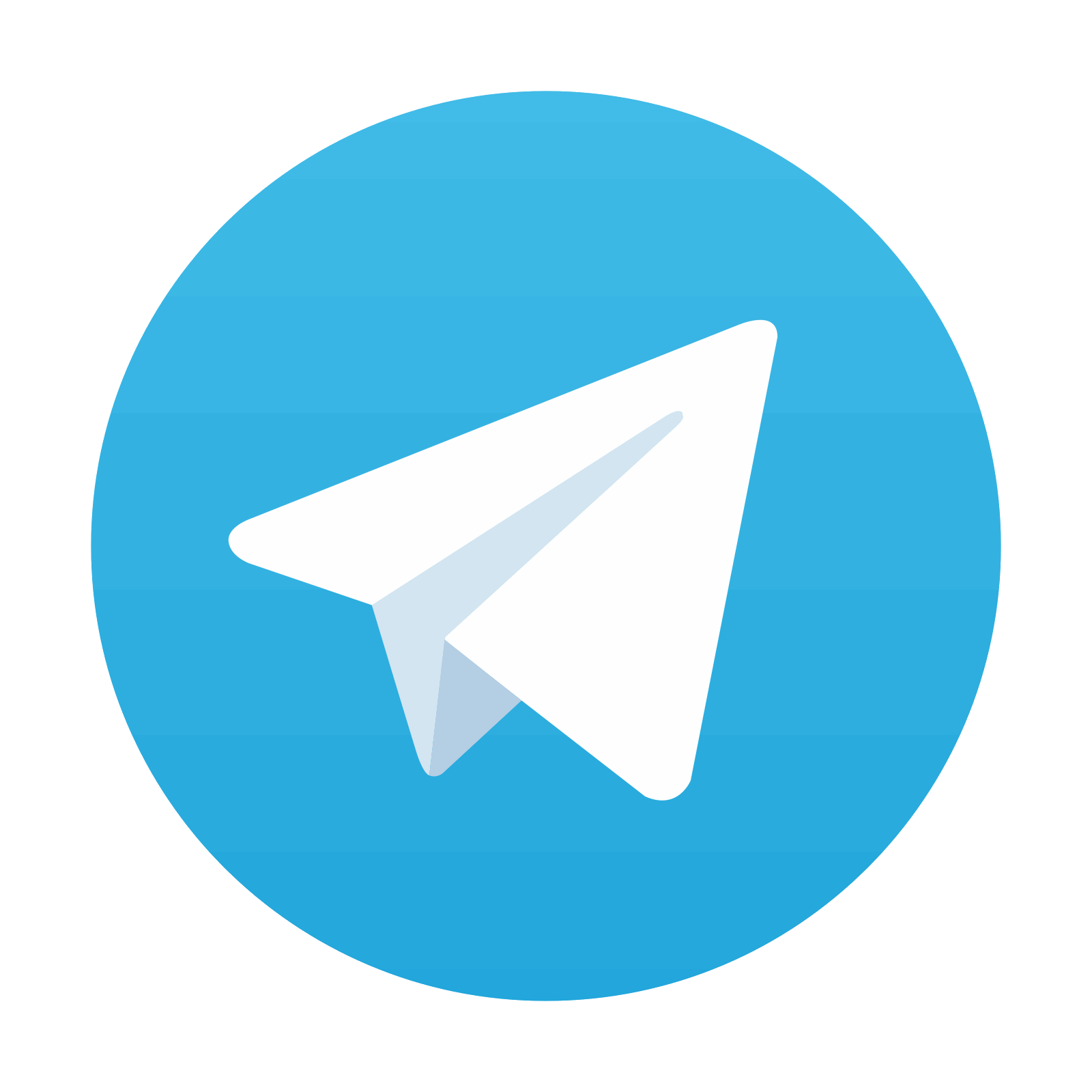
Stay updated, free articles. Join our Telegram channel

Full access? Get Clinical Tree
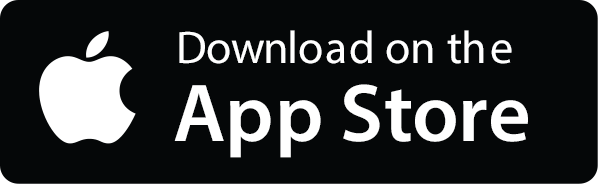
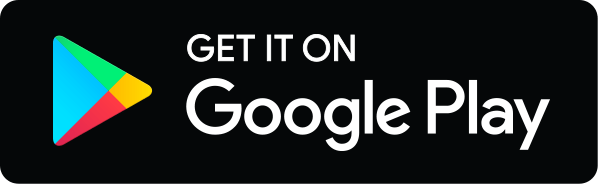