Abstract
This chapter discusses the transgenerational, antenatal, and early childhood factors that affect lung development across the life course. The consequences of these adverse influences may be direct, or secondary to an intermediate disease state such as prematurity and obesity. Many of these adverse effects cannot be remedied; we cannot stop atopic women bearing children, for example! There are two important take-home messages. The first is that we must identify and focus on what is preventable. This means legislation to protect vulnerable lungs against tobacco and nicotine (cigarettes and e-cigarettes) and traffic pollution; the institution of affordable and practical measures to minimize or eliminate exposure to biomass fuel; and being sure that due weight is given to the longer term consequences of Caesarean section by women and obstetricians when planning delivery. The second is to convince the community, especially adult thoracic physicians, that many lung diseases would disappear if our vulnerable fetuses, babies, and infants were better protected from the consequences of adult behavior.
Keywords
antenatal insults, prematurity, maternal factors, birth weight, gestational age, nutrition, asthma, atopy, stress, antibiotics, viral infections, tobacco, pollution, chronic obstructive pulmonary disease
Introduction
For too long, pediatricians and adult respiratory physicians have operated within developmental silos to the detriment of both and, more importantly, to the detriment of children. There are four reasons why better interactions between pediatricians and adult chest physicians are crucial for lung health. First, early life, pregnancy, and even preconception factors impact adult lung function and health; second, new chest diseases are coming to adult chest clinics as a result of advances in pediatric respiratory care, the classical disease being the survivors of prematurity; and third, children with diseases conventionally the province of the pediatrician (e.g., cystic fibrosis [CF], Duchenne muscular dystrophy) are now surviving into adult life, and pediatricians need to educate their adult colleagues about these new issues. Finally, adult physicians have very large cohorts from which we can learn in pediatrics; for example, the increased risk of respiratory infections in adults treated with inhaled corticosteroids (ICS) sounds a warning note in pediatric practice.
An illustration of the danger of working in silos is the first figure in the classical Fletcher and Peto paper showing the trajectories to chronic obstructive pulmonary disease (COPD), which could be called the “Star Trek” figure, the adult beamed down with a normal forced expired volume in the first second (FEV 1 ), with no concept that early life was of any relevance or even existed. In fairness, their second figure did acknowledge the possibility of failure to reach the full potential of lung development, but even today, we suspect that this is mere lip service for many.
Two recent papers have shown how interactions between pediatric and adult studies can build understanding of pathophysiology. The adult study finally nailed that COPD is a disease set up in childhood and that adult factors divorced from an understanding of pediatric risk factors are irrelevant; specifically, smoking is neither necessary nor sufficient for the development of COPD. A large study combining three adult cohorts defined two pathways to COPD, contributing equally to the burden of the disease: normal spirometry at age 40 years, with accelerated decline thereafter, and low FEV 1 at 40 years with a normal rate of decline. Of those not attaining the normal plateau (determined before adult life, see below) 26% developed COPD at follow-up, whereas if a normal plateau was reached, the risk was 7%. Neither from this nor any of the other four major studies reporting decline in lung function over time was there any consistent adult factor identified that was associated with accelerated decline. The pediatric paper was a follow-up of the Childhood Asthma Management Program (CAMP) asthma study participants, in whom annual prebronchodilator and postbronchodilator spirometry was performed into the third decade of life. Roughly equal proportions had a normal growth pattern and normal rate of decline, a normal growth pattern with accelerated decline, reduced growth with normal rate of decline, and both reduced growth and reduced rate of decline, interestingly a category not found in the COPD paper. Clearly, more follow-up data is needed to be sure this is a true decline rather than a transient dip in growth. This discrepancy may be related to the fact that the COPD study recruited all comers, and this last trajectory may have been missed because there were insufficient asthmatics; in other words, this last trajectory may only be seen in asthmatics, which hypothesis requires further confirmation.
Further underscoring the importance of pediatric factors in adult lung disease, a European study identified five markers of childhood disadvantage (maternal asthma and smoking, paternal asthma, and childhood asthma and severe respiratory infections), which in combination were associated with worse adult FEV 1 , a steeper rate of decline in FEV 1 , and increased COPD risk, at least as strong a signal as smoking. The conclusion is stark and unavoidable; if COPD is to be prevented, it is too late to start thinking about this in adult life.
Part of the difficulty of understanding the developmental perspective of adult diseases is that recall of childhood events by adults is notoriously unreliable; in one study, the incidence of pertussis and pneumonia (two fairly major pediatric respiratory illnesses!) was recorded prospectively. False positive and negative recall was very common ( Table 15.1 ), and the message is that retrospective recall may not be reliable. Similarly, so-called newly diagnosed asthma at age 22 years in 49 of 161 asthmatics diagnosed at all ages in the Tucson study was predicted by bronchial hyperresponsiveness and low lung function at age 6 years. Even the limited information that can be recalled is often not sought; in one survey, very few adult physicians asked about early life events.
Pneumonia | Pertussis | |
---|---|---|
Prospective parental report in first 7 years of life | N = 195 | N = 215 |
True retrospective recall in adulthood | 106/193, (55%) | 77/215 (36%) |
False retrospective recall in adulthood | 53/159 (33%) | 74/151 (49%) |
There are, however, important caveats when assessing the role of potential risk factors for long-term adverse outcomes; probable pathways are shown in Box 15.1 . Most often, it is not possible to untangle these possibilities, especially in the context of multiple interlinked exposures (e.g., increased risk of smoking, increased pollution exposure, living where there is a lot of neighborhood violence, reduced rate of breast-feeding all linked to low socioeconomic status [SES]). It is impossible to consider the long-term consequences of childhood disease without considering the long-term consequences of those factors underlying disease, some of which are discussed in more detail in earlier chapters in this book. However, the mantra that association does not prove causation should always be remembered. Causation can be demonstrated by intervention studies, or the likelihood bolstered by demonstration of biological plausibility by laboratory studies.
- ▪
The exposure is causative of the disease (disease would not happen without the exposure)
- ▪
An exposure may be causative of disease, but it is masked by other effects, for example, the interactions between cigarette smoke and glutathione transferase polymorphisms
- ▪
The underlying cause of being exposed is itself the reason for the association and not the exposure itself
- ▪
The exposure makes the disease manifestation worse but is not actually causative
- ▪
Treatment of the consequences of the exposure, but not the exposure itself, causes the disease
- ▪
An unknown underlying factor causes both the exposure and the disease; a good example of this is antibiotic prescription to infants, as discussed in more detail below
- ▪
The exposure is merely a marker for an unknown underlying exposure factor that causes the disease
- ▪
Berkson’s bias (a selection bias leading to hospital cases and controls in a case control study to be systematically different because the combination of risk exposure and occurrence of disease increases the likelihood of hospital admission). In other words, the harder you look in a selected population, the more you will find.
New diseases and the more prolonged survival of children with “childhood” diseases make cross-developmental interactions ever more important. Survivors of preterm birth have fixed and variable airflow obstruction, but no evidence of eosinophilic airway inflammation and are not helped by ICS. It is essential that adult physicians understand this is different from atopic asthma (and probably also COPD later in life) and needs different approaches. The increased longevity in CF has led to the importance of reduced bone mineral density and insulin deficiency being appreciated, which has in turn meant that pediatricians diagnose and treat these complications early. The next challenge will be the high prevalence of colonic malignancy and whether any childhood management strategies can reduce this risk.
How the Lung Develops in Health and Disease
Knowledge of normal lung development is essential if the long-term consequences of disease are to be understood. This is covered in detail elsewhere, so is only briefly summarized here. Interestingly, there is increasing evidence of adverse, preconception, transgenerational effects on the fetus. Thus, two studies have suggested that grandmaternal smoking increases the risk of her daughters’ children having asthma even if the daughters themselves never smoked. This has been attributed to epigenetic mechanisms, but it is not easy to reconcile this explanation with the mammalian germline reprograming that removes epigenetic signatures immediately after fertilization. The effect is not accounted for by the increased risk of asthma in the daughters of smoking mothers. Clearly, more work is needed to understand the mechanisms of these transgenerational effects and to determine if other adverse insults such as pollution may have the same effect.
Normal Antenatal Lung Development
This is described in more detail in Chapter 2 . The embryonic phase lasts from 0 to 7 weeks of gestation, and the primitive lung buds are outpouchings from the primordial gut. An anterior diverticulum appears at 4 weeks, the diverticulum bifurcates at 5 weeks, and lobes and segments become delineated thereafter. The main pulmonary arteries develop during this time, and blood vessel development follows the airways. From an early stage, there is phasic airway contraction and relaxation that are thought to be important in growth factor release. During the pseudoglandular phase, from 6 to 17 weeks, all generations of airways and their accompanying vessels are laid down, the latter by vasculogenesis ( de novo blood vessel formation); thereafter airways increase in size but not number. From 16 to 26 weeks is the canalicular phase, during which time, the acinar structures form, surfactant synthesis in type 2 cells becomes apparent, and the respiratory barrier thins. True alveoli and their accompanying blood vessels first appear in the saccular phase from 26 to 35 weeks; the vessels develop by angiogenesis (outsprouting from existing vessels) and the alveoli develop by secondary septation. The details of the molecular pathways and genetic control of these processes are beyond the scope of this chapter; the reader is referred to recent reviews and manuscripts. Important in this scheme is that adverse influences on airway branching are active in the first trimester and those active on airway caliber in the second and third trimester of pregnancy; and anything that interferes with the normal airway branching pattern will by definition adversely impact on the final alveolar numbers.
Adverse Effects Across the Life Course: Introduction
Although it is convenient to separate adverse influences into discrete developmental blocks such as antenatal, childhood, and adult life, this is to some extent artificial, since environment across the developmental stages is closely linked. A recent study attempted to take a more holistic look, spanning pregnancy and infancy. They reported that in adjusted analyses, there was a dose-dependent increased risk of subsequent asthma with maternal urinary tract infection and maternal and infant antibiotic use and a decreased risk if there were more older siblings at home. Delivery by Caesarean section increased the risk of subsequent asthma. The greater the number of risk factors, the greater the risk of asthma. However, these are all associations and are discussed in more detail below.
Adverse Effects Operating Antenatally
There are four main mechanisms whereby antenatal insults may impact on long-term outcomes, both disease in childhood and subsequent adverse effects in adult life. These are by leading to abnormal lung structure by causing abnormal immunological function and indirectly by causing either or both low birth weight and prematurity. The fourth mechanism, fetal programming, has been much less studied in humans, but there is evidence that early and discrete adverse exposures in animals prime adulthood responses to allergens and infections. A challenging human study showed that adult survivors of primary pulmonary hypertension of the newborn had much greater hypoxic pulmonary hypertensive responses than controls. It is of course impossible to be certain whether this relates to fetal programing or different developmental manifestations of some unknown underlying condition.
Tobacco and Nicotine Exposure
These are the most studied adverse influences. Structural changes have been most studied in animal models; of note, nicotine itself is damaging, calling into question the safety of e-cigarettes, nicotine patches, and nicotine chewing gum during pregnancy. Either may be safer than cigarettes, but this is unproven, and they certainly cannot be considered harmless.
Nicotine exposure in pregnancy has been shown to lead to airway remodeling, with increased deposition of type 1 and 3 collagen, airway lengthening and narrowing, airway wall thickening, and loss of alveolar tethering points leading to airway instability. The airway wall may be thickened postnatally. There is also increased MUC5AC expression in bronchial epithelial cells. The postnatal functional readout of these structural changes is airway hyperresponsiveness (AHR) even in the absence of allergen exposure. This is of particular importance, since three cohort studies have shown that AHR in the newborn period, long before there is any evidence of airway infection or inflammation, is associated with long-term adverse respiratory outcomes. Children of mothers who smoke are more likely to have asthma, and more likely to smoke themselves, thus perpetuating the cycles of avoidable damage to the lungs. Finally, tobacco exposure may lead to premature emphysema, with reduction of secondary septation and enlargement and simplification of alveoli; the relative contributions to this worrying effect of antenatal and postnatal smoke exposure have not been determined. Tobacco exposure of pregnant women is associated with a number of alterations in cord blood, including an increased responsiveness to allergens of cord blood mononuclear cells, abnormal Toll-like receptor function, and abnormal cytokine responses with a readout of increased viral wheeze in the first year of life. The effect of maternal smoking on infant atopy is controversial with some finding no effect at all. However, recently an association between maternal smoking and food allergy and also with atopic dermatitis has been reported, and a recent meta-analysis confirmed an association with atopy. The mechanism may be via reduction in cord blood regulatory T cells (Tregs). Finally, that active smoking is associated with prematurity and low birth weight has long been known; new data also shows that reduction of passive smoke exposure leads to reductions in preterm delivery.
Antenatal Effects of Pollution
There is increasingly compelling evidence that exposure of pregnant mothers to environmental pollution may adversely impact the fetus. In a study from Spain, the developmental residential exposure to benzene and nitrogen dioxide was related to spirometry at age 4.5 years in 624 children. There was a dose-dependent reduction in spirometry with increasing exposure levels in pregnancy but no other time point. This was worse for allergic children and those of low SES. In another study of 736 North American children, mostly ethnic minorities, but also mostly nonsmokers, there was an association between PM 2.5 exposure levels between 16 and 25 weeks of gestation and the development of early childhood asthma; however, this result only appeared in boys. It is likely that there will be adverse effects on the fetus of maternal biomass fuel exposure during pregnancy, but this has yet to be determined.
Maternal Malnutrition and Obesity
It is a shameful commentary on the human condition that maternal malnutrition is still an issue in the 21st century. The best-known long-term data are from the Dutch famine lasting 5 to 6 months at the end of the Nazi occupation in the Second World War. Famine exposure in the first and second trimester of pregnancy was associated with wheezing, but not airflow obstruction or increased atopic sensitization at follow-up in adult life; wheezing remained significant after adjusting for relevant confounders, and wheezing was independent of birth weight in this group (famine-born babies were on average 250 g lighter, a fairly small effect). An important follow-up paper demonstrated that not merely was there more wheezing illness at age 50, but also more coronary heart disease, hyperlipidemia, altered clotting, and obesity after early gestational exposure to famine. Microalbuminuria and decreased glucose tolerance were reported in those exposed to famine in late gestation.
Maternal obesity is likely to become ever more common. Prepregnancy obesity is associated with early wheeze and asthma in the offspring. There is no doubt that pregnancy obesity (which is of course closely linked with prepregnancy obesity) increases the risk of obstetric complications, but is also associated with wheeze in infancy and childhood. In a cohort study in which more than 38,000 mother-baby pairs were identified, maternal obesity in pregnancy was also associated with wheeze in the first 18 months of life in the baby ; this was confirmed by a pooled analysis of more than 85,000 mother-child pairs. Risk increased linearly with body mass index, and the risk was not mediated via obstetric complications, prematurity or low birth weight. In another study of more than 6000 mother child pairs, prescription of inhaled bronchodilators in the first 4 years of life was associated with prepregnancy obesity.
Antenatal Effects of Acetaminophen?
There is ongoing controversy about whether acetaminophen given to mothers in pregnancy or to infants postnatally is associated with the development of asthma. A recent murine model in which acetaminophen was given using various regimens to pregnant mice, lactating mice, and mouse pups, alone or in any combination, and no effect could be shown on the development of murine allergic airways disease. A recent birth cohort study showed an association between acetaminophen use in both the first and last trimesters of pregnancy and subsequent wheeze in the offspring. However, this disappeared when adjusted for confounding factors, and there was no association between acetaminophen use for noninfectious reasons and subsequent wheeze. However, given there are other available antipyretic and analgesic medicines such as ibuprofen (the safety of which has not been challenged), it would seem prudent to avoid acetaminophen in pregnancy and childhood.
Maternal Hypertension?
Maternal hypertension in pregnancy has also been associated with an increased risk of wheeze, although this has recently been challenged in the Avon Longitudinal Study of Parents and Children (ALSPAC) study. They found that maternal hypertension before pregnancy, but not gestational hypertension, was associated with subsequent childhood wheeze, but even this association needs replication.
Maternal Antibiotic Use?
Antibiotic prescription in pregnancy has been associated with subsequent wheeze, and certainly in a murine model, antibiotic use in pregnancy affects adaptive antiviral immune responses via alterations in the pup’s microbiome. However, a recent birth cohort study has clarified the association. Maternal use of antibiotics in the first trimester was not associated with wheeze; in the last trimester, there was an association with wheeze, and this remained, albeit weakly, when adjusted for confounding factors. Genitourinary infection in pregnancy increased the risk of subsequent wheeze in the offspring, irrespective of antibiotic treatment. No mechanisms for these possible associations were defined.
Maternal Stress in Asthma—A Developing Field
There is increasing evidence that maternal stress (in the very broadest sense) is important in disease outcomes, in particular, asthma. There are extra pitfalls in determining this relationship ( Box 15.2 ).
- ▪
Does stress per se cause asthma? This could be made more complicated if there is a time delay between the onset of stress and the onset of asthma.
- ▪
Does stress lead to overinterpretation of symptoms? Or lead to symptoms as a shield, or symptoms as a manifestation of stress?
- ▪
Does stress lead to symptoms that are misdiagnosed as asthma; the obvious examples are dysfunctional breathing and vocal cord dysfunction?
- ▪
Do stress-associated behaviors (e.g., smoking) cause symptoms?
- ▪
Does the source of stress (poverty, neighborhood violence, pollution) also lead to asthma symptoms?
- ▪
Are stress and asthma just fellow travelers?
- ▪
Does maternal stress affect how mothers and professionals react to asthma (admission more likely at a given level of symptoms, for example)?
- ▪
Since antenatal and postpartum maternal stress are likely closely linked, it may be difficult to determine which is important.
- ▪
Does stress lead to Berkson’s bias (above)?
The interaction between maternal atopy and wheeze was studied in 653 families. Stress was defined as negative life events during pregnancy and in the first and second years postpartum. Mothers were asked about the presence of wheeze in the babies quarterly. Maternal stress was a risk factor for wheeze, but only in nonatopic mothers. This was taken forward in a second, more mechanistic study in 557 families. Prenatal maternal stress was defined as any of financial hardship, difficult life circumstances, community violence, and poor neighborhood housing. Cord blood mononuclear cells were incubated with several stimuli of the innate and adaptive responses. There were greater interleukin (IL)-8 and tumour necrosis factor (TNF)-α responses to infection-based, and a higher IL-13 and reduced IFN-γ to house dust mite (HDM) stimuli (T H 2 polarization), but there was no explanation of the detailed mechanisms. However, there is at least one possible way in which altered responses to infection may impact longer-term outcomes, namely via upper airway bacterial colonization. The first Copenhagen Prospective Studies on Asthma in Childhood (COPSAC) Study reported on 321 infants who had hypopharyngeal cultures performed at 1 month of age, which these were infants of atopic mothers. Twenty-one percent colonized with one or more of Staph aureus, Moraxella catarrhalis, Strep pneumonia, Haemophilus influenzae, and when followed up, they had worse wheeze outcomes, both in terms of percentage with any wheeze, and percentage with persistent wheeze. However, it should be emphasized that maternal stress was not measured in the COPSAC study.
A further study reported conflicting interactions between atopy and maternal stress. The investigators approached 989 women, of whom 488 (<50%) were actually studied. They used the Crisis in Family Systems-Revised (CRISYS-R) questionnaire (which has 11 domains that were summarized to account for stress in multiple domains). They performed prenatal environmental HDM sampling and measured cord blood immunoglobulin E (IgE). They reported that maternal stress was associated with higher cord blood IgE; confusingly, for atopic mothers, the strongest association was in the high HDM exposure group, whereas in the nonatopic mothers, the reverse was found, the strongest association being in the low HDM group. These findings are difficult to explain, although supportive of an association between maternal stress and atopic outcomes.
Even more intriguing was a study looking at early life events in the mother herself long before pregnancy. The investigators defined the mother’s SES in childhood, age up to 10 years, by whether she lived in a home owned by her family. They recorded potential pregnancy effects, including sociodemographics, exposure to violence or trauma, financial status, and exposure to allergens and traffic pollution. Postnatally, they recorded reported wheeze every 3 months. They reported that lower maternal SES was associated with higher cord blood IgE and more infant wheeze. They also reported direct effects, namely cumulative maternal adversity was independently associated with cord blood IgE; and indirect effects, operating through adult SES, cumulative stress, and pollution. They proposed a complex model integrating all these findings, but it has to be said that mechanistically there are no answers and the model must be said at this time to be hypothesis generating not definitive.
In summary, maternal stress is clearly associated with altered cytokine responses (T H 2 and innate immune responses to infection) in the baby. There is also a readout with increased reports of wheeze in the offspring, but the weaknesses of relying on parental reports of wheeze have been highlighted elsewhere. The pathways are clearly complex and ill-understood, although the interactions between stress and atopy are important. However, we are a long way from proving causation, since so many factors cosegregate with stress.
Long-Term Effects of Events During Delivery
Caesarean section delivery is associated with an increased risk of atopic disorders in the baby probably mediated via an effect on the fetal microbiome. Especially if at least one parent is allergic, children delivered by Caesarean section are more likely to have asthma at 8 years of age, and children of nonallergic parents are more likely to be sensitized. Since there are no randomized controlled trials of Caesarean section delivery, it is not clear if the reported asthma prevalence changes are related to Caesarean delivery itself or to the reason Caesarean delivery was undertaken.
A further factor to be considered is changes in the way newborns (including term newborns) are resuscitated. Many babies require a brief period of resuscitation immediately after birth and thereafter remain well and are not reevaluated. Resuscitation policies have become less aggressive, with intubation and hyperoxic gas mixtures given after a delay, and there is evidence in animals that this may be less damaging to the newborn lung. The long-term sequelae of newborn resuscitation have not been considered outside the setting of prematurity, but the long-term effects of these changes are worth at least considering.
The Canadian CHILD (Canadian Healthy Infant Longitudinal Development national population-based birth cohort [www.canadianchildstudy.ca]) study has also reported on the interactions between mode of delivery, the use of intrapartum antibiotics, and subsequent breast-feeding on the fecal microbiome in infancy. Intrapartum antibiotics for whatever indication (Group B streptococcal prophylaxis, prolonged rupture of membranes, and elective or emergency Caesarean section) led to alterations in the fecal microbiome at 3 months of age and these persisted in babies delivered by Caesarean section to a year of age, particularly if the Caesarean section was an emergency and the infant was not breast-fed. The development and importance of the early life microbiome is discussed in more detail below.
Birth Weight and Gestational Age
The long-term consequences of extreme prematurity are considered later; here we discuss the effects of modest decrements in birth weight and gestational age, and some of which have hitherto been thought to be in the “normal” range. A meta-analysis has demonstrated that low birth weight of whatever underlying cause is associated with subsequent asthma across the life course. There is a linear relationship between birth weight (adjusted for all known relevant maternal factors) and spirometry both at the peak in the early twenties and at age 45 to 50 years. Tobacco smoking causes preterm delivery and importantly the risk is reduced by tobacco legislation ; it is obviously of the first importance to focus particularly on factors that are remediable, such as smoking and pollution, rather than those that are not, such as maternal atopy. Modest degrees of prematurity (up to 37 weeks gestation) are associated with impaired spirometry in the late teenage years and increased prescription of asthma medications in childhood. Low birth weight of itself is a risk factor for subsequent asthma. There may be a differential effect of low birth weight in babies who are small (SGA), as opposed to appropriate (AGA), weight for gestation age; at age 20 to 22, spirometry was strongly predicted by birth weight in the SGA, but not the AGA, group.
Normal Postnatal Lung Growth
Most is known about airway growth and the Global Lung Initiative has provided the best all-age spirometry data. These show a steady rise in lung function from the preschool years to a plateau between ages 20 and 25 years and then a steady decline. If these figures are back-extrapolated to birth cohort studies that have used either the rapid thoraco-abdominal compression technique (RTC) or the raised volume RTC (RVRTC), which is more closely relates to spirometry in children and adults, it is clear that there are three key issues that are needed to ensure lifelong airway health ( Box 15.3 )
- ▪
Normal lung function as shown by RVRTC/RTC at birth (genes and antenatal factors)
- ▪
Normal lung growth in the first two decades of life (genes, postnatal and antenatal factors)
- ▪
Normal rate of decline, from the fourth decade of life (genes, antenatal and postnatal factors; minimal contribution from adult life effects)
RTC, Rapid thoraco-abdominal compression technique; RVRTC, raised volume rapid thoraco-abdominal squeeze technique.
We have learned important lessons from birth cohort studies. There is no study that has extended over the entire life course, and so we rely on a series of overlapping cohorts, reviewed in. Although there are some discrepancies, the general message is that lung function at age 4 to 6 years is determined by lung function and AHR soon after birth, and thereafter tracks; hence decrements at age 4 to 6 are reflected in adult lung function, at least to age 50. There may be further deterioration over the life course, but no improvements. This has obvious implications for adult disease.
Understanding AHR and its long-term consequences requires a developmental perspective. Three groups have measured neonatal AHR and all showed significant relationships with long-term respiratory outcomes, albeit slightly different. COPSAC showed that 40% of airway obstruction at age 7 was determined antenatally and 60% postnatally and that AHR in the newborn period was the strongest predictor of asthma at age 7. Neonatal AHR is clearly not related to airway inflammation or previous infection, and must be determined by anatomical factors; thus, in small airways, resistance increases more for a given proportionate radius change. Although AHR and lung function at birth are said to be independent risk factors for childhood lung function, abnormal anatomy is likely to be the root cause of both. Finally, a study of AHR from birth to adult life showed that the relationship in adulthood between AHR and asthma was established before 6 years of age.
There is much less known regarding developmental changes in lung volumes. They probably increase through childhood, with at least one study suggesting a phase of accelerated growth during puberty, and more marked in boys than girls, reflecting changes in the dimensions of the thoracic cavity.
Alveolar development is a controversial subject. Conventionally, this has been described as being a largely postnatal phenomenon with a rapid phase in the first 2 years of life, while there is some neo-alveolarization until about age 8 years, at which point, alveoli increase only in size and not number. This has been challenged by some controversial studies in which hyperpolarized Helium (He 3 ) has been used to infer alveolar size from observation of path lengths after inhalation. These studies have suggested that neo-alveolarization continues throughout somatic growth, which is supported by direct morphometric measurements in primates. If this is the case, the implications are two-fold; first, there is greater scope for recovery from adverse neonatal events than we thought; and second, the period of vulnerability of the growing alveoli extends further than we previously considered. These are both particularly important in the context of preterm delivery and adverse environmental exposures.
Adverse Impacts on Lung Growth and Relationship to Disease Outcomes
As with neonatal adverse influences, the postnatal interactions between adverse effects and their consequences are complex. Included in this are the ways whereby an adverse influence causes long-term respiratory morbidity by leading to obesity, which obviously of itself causes substantial respiratory morbidity. Another example is wheezing and asthma, which are associated with adverse long-term effects, but have magnified effects by acute exacerbations of wheeze or “lung attacks” (below).
Effects of Nutrition on the Life Course of Lung Development
The single most easily modifiable trait that may impact on long-term outcomes is breast-feeding. The general health merits of breast-feeding are too well known to recapitulate here. Whether breast-feeding protects against asthma and wheeze is controversial; a meta-analysis showed benefit in the first 2 years of life, with diminishing effects thereafter ; however, a recent birth cohort study did not confirm any protective effect at age 7 years. Breast-feeding is also associated with a reduced risk of snoring and sleep-disordered breathing, and likely, but not certainly, protects against obesity. Early and rapid weight gain may be associated with poorer lung growth in addition to the effects of low birth weight and SGA. However, paradoxically, greater weight gain (but almost certainly not obesity) in later childhood may be associated with better spirometry. The multiple respiratory adverse effects of childhood obesity including a number of phenotypes of wheeze, deconditioning, and obstructive sleep apnea are well known. There is mounting evidence that the origins of later obesity are antenatal and in the first 2 years of life. In a systematic review, 282 publications were identified, from which high maternal prepregnancy body mass index, prenatal tobacco exposure, excessive maternal weight gain in pregnancy, high birth weight, and greater weight gain were implicated as risk factors for childhood obesity. There was less strong evidence (fewer studies) implicating gestational diabetes, child care attendance, poor maternal-infant bonding, low SES, reduction in infant sleep time, inappropriate formula feeding, introduction of solid foods before 4 months of age, and infant antibiotic exposure. Clearly many of these risk factors are interlinked, such as low SES, smoking, and low rates of breast-feeding.
Passive and Active Exposure to Tobacco Smoke
The effects of passive smoking were described in a series of meta-analyses, which have been recently updated. Passive smoke exposure is associated with an increased risk of sudden infant death syndrome, more and more severe childhood upper and lower respiratory infections, and more childhood wheeze. Finally, maternal smoking and the child taking up smoking (which is more likely if the parents smoke) have additive effects on the child’s lung function.
Environmental Pollution
Globally, the most significant antenatal exposure is indoor exposure to biomass fuels. In a study from Guatemala, a subset of children whose families had been randomized to receive a chimney stove to reduce indoor pollution either at birth or at 18 months of age underwent spirometry at around 5 years of age. Later stove introduction was associated with a statistically significant reduction in peak flow growth and a large but not statistically significant decrement in FEV 1 .
There is a large amount of literature on the adverse respiratory effects of ambient pollution. Lung growth over time is adversely affected by pollution, meaning that the normal plateau of lung development will not be reached, putting the child at risk of later COPD. Furthermore, pollution interacts with respiratory infection to increase the prevalence and worsen attacks of preschool wheeze and asthma with the adverse long-term results described below. Encouragingly, legislation to reduce air pollution is also temporally associated with improved lung growth in children, identifying outdoor as well as indoor pollution as something that can and should be dealt with to preserve long-term lung health.
Asthma, Atopy, and Acute Attacks of Wheeze
Three factors reflecting long-term consequences of childhood asthma and wheeze emerge from the literature: (1) the importance of different temporal patterns of wheeze ; (2) the significance of different patterns of atopic sensitization ; and (3) the crucial importance of attacks of wheeze, or better “lung attacks.” These last have been the subject of attention in other contexts (as follows) including CF and primary ciliary dyskinesia. It has been suggested that these are so important that the rather feeble word “exacerbation,” implying a mere temporary and reversible inconvenience, should be replaced by the more forceful “lung attack” to reflect the long-term adverse effects of these episodes in many airway diseases.
In terms of wheeze patterns, the birth cohort studies have confirmed the shrewd observations of our forebears that all wheeze is not the same, and 4 to 6 patterns or phenotypes can be discerned, which are broadly reproducible across the cohorts, and associated with particular gene expression patterns. Broadly, there are early wheezing periods in the first 0 to 4 years of life and persistent and late onset wheeze phenotypes leading to symptoms into mid-childhood. The cohorts with more sophisticated epidemiological classifications have yet to reach late adulthood, so the long-term consequences can only be inferred. An alternative, and more clinically useful, time-honored classification, recently endorsed by the European Respiratory Society, is into “wheezy bronchitis”/episodic viral wheeze (symptoms only during viral upper respiratory tract infections) and asthma/multiple trigger wheeze, in which additionally there are symptoms between infections, with typical asthma triggers such as exercise and allergen exposure. The Aberdeen cohort, which used just this classification, has been followed into the seventh decade and showed that both groups were associated with COPD, but neither were associated with an accelerated decline in spirometry ; hence, failure of normal lung growth in childhood was the cause of COPD in adult life. In this context, it is worrying that one-third of children with relatively mild asthma in the CAMP study had impaired growth in spirometry irrespective of whether they were prescribed ICS. This is one more indication of the disconnect between airway inflammation and airway remodeling, which was presumably the cause of impaired growth.
We have also come to realize that all atopies are not equal, and approaches such as the quantification of atopy and determining the temporal patterns of sensitization lead to delineation of subgroups of atopy of different significance. The Manchester cohort used a machine learning approach to define patterns of atopy, and this approach identified early multiple atopic sensitization and persistent wheeze as being the only combination of wheeze and atopic phenotypes that predicted progressive airflow obstruction measured by specific airway resistance. Additionally, attacks of wheeze worsened the progression of airflow obstruction.
Lung attacks have been specified as important in the evolution of lung function in two contexts: the first is the Manchester birth cohort study ; and the second is in a post hoc analysis of the Inhaled Steroid Treatment as Regular Therapy in Early Asthma (START) trial in which asthma lung attacks were shown to be associated with a long-term effect on airway obstruction; in this case, the effect was abrogated by treatment with ICS.
Finally, the Melbourne study has shown that people with asthma that remits and normal subjects have the same spirometry in the sixth decade of life, whereas asthma that does not remit (even if not severe at recruitment) is associated with decrements in lung function across the life course. Risk factors identified for persistence were severe childhood asthma, female gender, and childhood hay fever.
Stress and Its Outcomes
A number of manuscripts link childhood psychological stress with asthma attacks; but as in other areas, associations are described, and there are no intervention trials to prove that psychological intervention is beneficial. In the most significant prospective longitudinal follow-up study, 113 children were approached and 90 participated. They filled in the Psychological Assessment of Childhood Experiences (PACE) questionnaire at baseline, 9, and 18 months and undertook home peak flow monitoring. Acute, severe stresses were defined as a death or divorce in the family, while chronic stresses were chronic physical or mental illness in family, substance abuse, family discord, school-related stress (bullying), and poor living conditions. They found that female gender, higher baseline severity, frequent previous attacks, and (unsurprisingly) the autumn to winter season were all associated with more attacks. Although this is expected, these positive controls add value to the study by demonstrating adequate power to show real associations. They reported that social class and chronic stress were not associated with acute attacks. Severe acute events, with or without high chronic stress, however, did increase the risk of new asthma attacks. There were quite complex time-relationships between stress and asthma attacks. If there was no background chronic stress, there was a time lag of around 2 weeks before the increased risk of acute attacks, which was manifested in the subsequent 4 weeks. If the child suffered from acute on chronic stress, there was an almost immediate increased risk of asthma attacks. In other work, detailed analysis revealed a biphasic risk pattern with early and late periods of vulnerability.
The effects of positive life events have also been studied. “Acute” positive events were defined as receiving valued presents, joining clubs (new activities and friends), and winning prizes. “Long-term” positive events included being a member of a sports team and having a hobby. The group had low to medium chronic stress levels, but not high chronic stress. They found that positives could reverse the risk of an acute stressful event, but the presence or absence of long-term positives did not affect attack risk.
Finally, a horrifying paper from Brazil studied the effects of violence on asthma attacks. A total of 1232 parents/guardians were surveyed, and, appallingly, more than three-quarters had been exposed to violence. Children exposed to violence were more likely to report asthma (International Study of Asthma and Allergies in Childhood [ISAAC]; 28.4% vs. 16.4%), and if violence was maximal, the odds ratio for asthma was 1.94, with 95% confidence intervals of 1.12 to 3.36. Of course, one cannot exclude that what was being reported by the children was hyperventilation or panic attacks, triggered in part by traumatic events.
Another approach is to look at new cases (incident) of asthma rather than exacerbations of a preexisting disease. One group conducted a postal survey of 16,681 males and females, aged 20 to 54, to determine the presence of stressful life events and determined the onset of asthma from national registries. There were 192 cases of incident asthma, which were associated with acute stress; the worst were illness of family member, marital problems, divorce or separation, and conflict with a supervisor. Again, whether they were reporting true asthma or dysfunctional breathing could not be determined.
Antibiotics?
This literature clearly shows the importance of not confounding causation and association. Many papers show an association; a recent study in more than 900 babies followed to age 11 years obtained data on prescription of antibiotics, wheeze prevalence, and asthma attacks. Peripheral blood mononuclear cell responses to rhinovirus and respiratory syncytial virus, and H. influenzae and Streptococcus pneumoniae were assessed at 11 years, and genotyping for polymorphisms at the 17q21 locus was performed. The expected association of wheeze and asthma with antibiotic prescription was noted, but the children who were prescribed antibiotics were in fact significantly different from those who were not both in terms of immunological responses to viruses but not bacteria and 17q21 polymorphisms. Hence, the association between wheeze and antibiotic prescription is likely related to confounding factors and not causative.
Early Viral Infections
Acute viral infections are important causes of early respiratory morbidity due to bronchiolitis and wheeze, but whether viruses cause asthma to develop in an infant who would otherwise not go on to the disease or are a marker for an asthma propensity is controversial. It should be noted that these are not mutually exclusive. Cord blood studies (above) show that the host immune system may be programmed before birth such that the baby has a propensity to viral wheeze. Another study demonstrated impairment of respiratory function prior to the development of bronchiolitis, and this decrement tracked into mid-childhood. Respiratory syncytial virus has been most studied; infection with this virus in infancy is universal, but whether severe infection causes asthma is controversial. Recently, rhinovirus infection has been shown to be more strongly associated with subsequent asthma than respiratory syncytial virus, although association and causation are not the same. Probably, allergic sensitization precedes viral wheezing, and it is likely that sensitization rather than viral infections is important in pushing the trajectory of the child into school-age asthma, although this is by no means certain.
Microbiome
The lower airway is not sterile as was once believed based on conventional culture techniques. Molecular techniques, which detect a hundred-fold more bacteria, have shown that the airway has abundant flora. Furthermore, alteration in the normal lower airway flora may skew immune development, and interactions between allergen and the microbiome influence the body’s immune responses. Early nasopharyngeal bacterial colonization of human infants is associated with altered early immune responses, and a greater prevalence of subsequent wheezing and worse respiratory infections. It is likely that early upper airway colonization with pathogens is a sign of an underlying mucosal immune defect, but this is still unproven. By contrast, environmental bacterial and fungal diversity is associated with a reduced risk of asthma. The long-term effects of an abnormal early microbiome on lung aging are currently unknown, but the influences on asthma risk mean that the early microbiome will at least have an indirect effect on outcomes.
The Aging Lung
There are a large number of studies of lung aging, including population based and in those with established COPD. As discussed above, no single factor operative in adult life emerges as associated with accelerated decline in lung function; either no factors are identified, or they are not replicated. The Evaluation of COPD Longitudinally to Predict Surrogate Endpoints (ECLIPSE) study showed there was accelerated decline in current smokers, patients with a bronchodilator response, and patients with emphysema ; the Body Mass Index, Airflow Obstruction, Dyspnea and Exercise Capacity Index (BODE) study by contrast reported higher rates of decline in those with a higher basal metabolic index and a higher starting FEV 1 . A study in adults with severe asthma showed that an elevation in exhaled nitric oxide, especially in those with FEV 1 ≥ 80%, was predictive of accelerated decline in lung function. The likely pathological basis of this is an abnormal airway inflammatory response, elevation of CD8 and CD4 T cells in baseline bronchial mucosal biopsies and CD8, CD3, and granzyme B at follow-up.
Lung Development: More Than Just Airways
Much less is known about alveolar as opposed to airway growth, and it is mostly in the context of prematurity and its treatment. Neonatal hyperoxia, systemic steroids, and nicotine all impair neoalveolarization via effects on secondary septation in animals. Human He 3 data suggest that maternal smoking in pregnancy, as well as affecting the airway, may increase alveolar size and reduce their numbers leading to premature “emphysema.” Since it is nicotine that is implicated in both processes, the safety of e-cigarettes is called into question. There may be the alveolar equivalent of catch-up growth based on cross-sectional studies; there are no longitudinal neonatal data with extension into childhood and adult life. Carbon monoxide transfer (DL CO ) is a surrogate for the size of the alveolar-capillary membrane, and one group demonstrated that this was normal at rest and on exercise in adult life. He 3 data in preterm survivors in adolescence showed that alveolar size was normal, implying catch-up growth, but there were no measurements in the newborn period. Nitrogen washout can be used to partition gas mixing abnormalities into airway (S cond ) and alveolar (S acin ) components. Preterm survivors in childhood had as expected an abnormal S cond compared with controls, but S acin was normal, implying alveolarization had normalized (but again, there were no neonatal data). However, if in fact many alveoli had been completely destroyed, S acin would still be normal. However, taken together, there is suggestive evidence that alveolarization continues for longer and with more potential for catch-up growth than previously thought. Certainly, lungs apparently destroyed by necrotizing pneumonia usually recover completely, so there is certainly potential for catch-up. Intriguingly, being reared in hypoxic conditions at altitude appears to stimulate alveolarization but has no effect on airway function ; this raises the hitherto unexplored question as to whether keeping preterm survivors very well oxygenated is as beneficial as we currently think.
Summary: What Have We Learned About the Importance of Antenatal and Childhood Factors?
We have summarized factors across the developmental spectrum that clearly either lead to either or both of impaired lung function at birth and impaired lung growth in childhood leading to a failure to reach the normal adult plateau. There are clearly important genetic effects and gene by environment interactions discussed elsewhere in this book (see Chapters 3 and 4 ).
It is very clear that asthma and many if not most childhood wheezing syndromes, including atopic asthma, are not mere childhood inconveniences to be outgrown but have long-term consequences in terms of premature airflow obstruction and thus early onset of respiratory disability. The exact importance of multiple factors operative in an individual may not be possible to dissect, but the importance of a focus on antenatal and early childhood factors is indisputable. The combined effect of these adverse influences and their childhood consequences is to lead to an increased risk of adult COPD (below). However, what is also clear is that the rate of lung aging must be largely dependent on genetic and childhood factors. The inescapable conclusion is that if adult COPD is to be prevented, then efforts to do this must be focused in childhood.
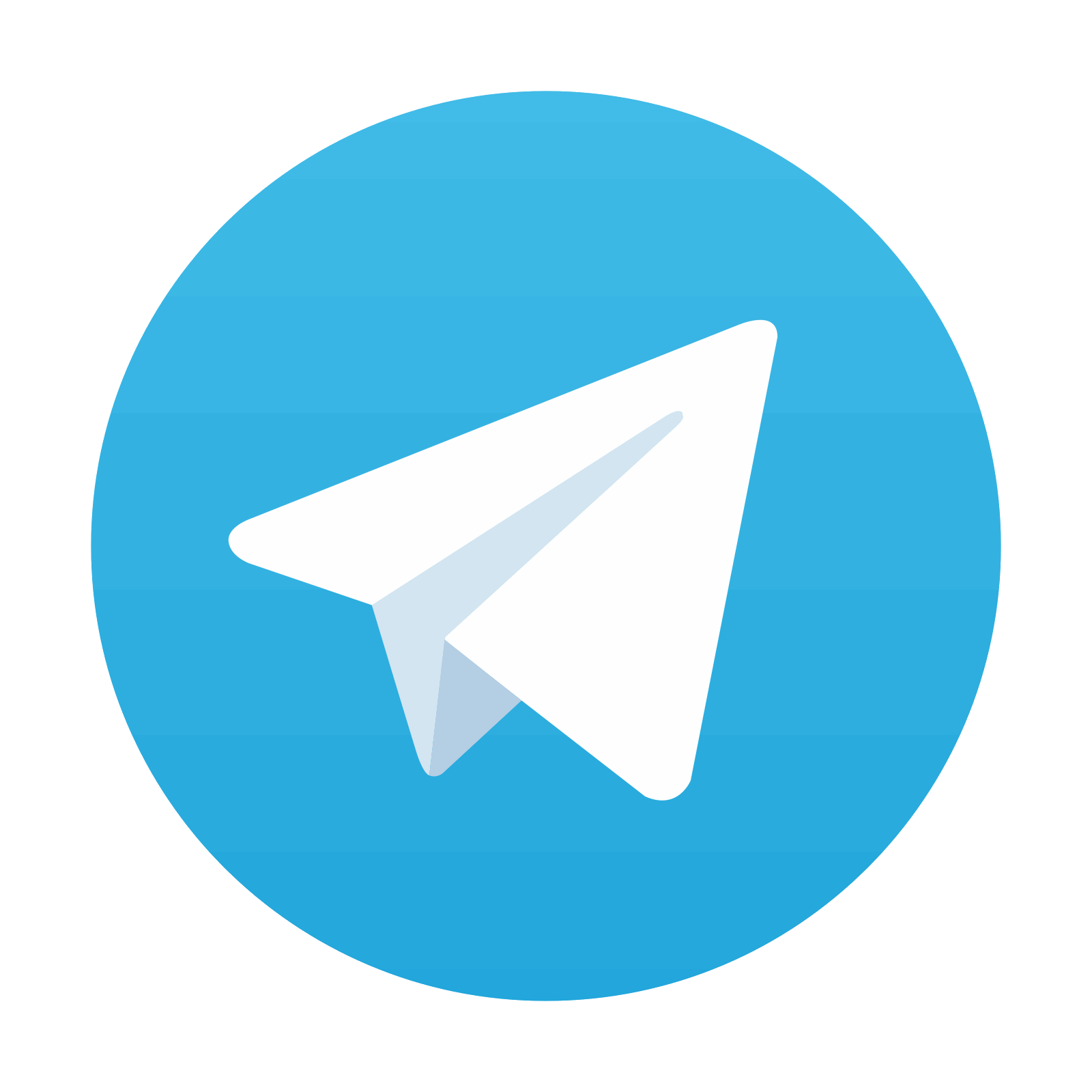
Stay updated, free articles. Join our Telegram channel

Full access? Get Clinical Tree
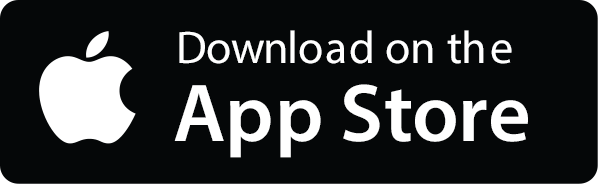
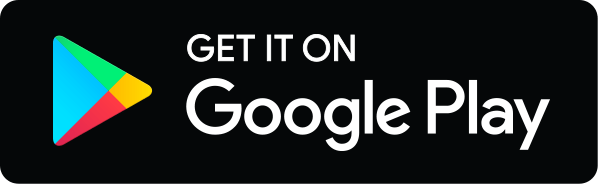