Background
Iron-mediated cardiomyopathy is the leading cause of death in patients with thalassemia major (TM). The identification of subclinical cardiac involvement in the early phases of the disease is important to optimize therapeutic strategies. The aim of this study was to identify early markers of cardiac dysfunction through new parameters of cardiac rotational dynamics and to look for a relationship with parameters of iron overload.
Methods
Twenty-seven asymptomatic patients with TM and 27 healthy control subjects were prospectively enrolled. All subjects underwent standard echocardiography and subsequent offline analysis to assess left ventricular (LV) rotation and longitudinal mechanics using speckle-tracking echocardiography. In all patients with TM, ferritin levels were measured, and a subgroup underwent cardiac magnetic resonance imaging.
Results
All subjects had normal parameters of cardiac function, although patients with TM showed significantly lower S′ values ( P = .030) and E′ values ( P = .025), with increased E/E′ ratio ( P = .003) and indexed left atrial volumes ( P = .022). Compared with controls, patients with TM had significantly reduced systolic apical rotation ( P = .006), LV twist ( P = .002), and LV torsion ( P = .001). Systolic and diastolic rotational peak velocities at the apical level were also significantly decreased in the TM group ( P = .003 and P = .011, respectively) with reductions of twisting and untwisting rates ( P = .003 and P = .001, respectively). Patients with TM also showed a significant reduction of longitudinal displacement from the two-chamber apical view ( P = .042) but preserved longitudinal strain and strain rate. Patients with T2* values > 20 msec had preserved rotational function, while those with T2* values < 20 msec showed significantly lower mean values of LV peak basal systolic rotation (−3.1 ± 1.4° vs −6.2 ± 2.6°, P = .016), LV peak apical systolic rotation (3.4 ± 1.3° vs 6.4 ± 3.1°, P = .045), LV twist (4.8 ± 2.5° vs 10.9 ± 4.9°, P = .012), and LV torsion (0.6 ± 0.2°/cm vs 1.4 ± 1.6°/cm, P = .010). LV torsion was negatively related to ferritin levels ( r = −0.47, P = .013) and directly to T2* values ( r = 0.64, P = .007).
Conclusions
LV rotational dynamics in asymptomatic patients with TM are negatively related to iron overload. Rotational function of the left ventricle is preserved in patients with normal T2* values. These new parameters are useful for an early diagnosis of cardiac involvement.
Beta-thalassemia major (TM) is an inherited blood disorder characterized by impaired β-chain output. This leads to severe anemia secondary to inefficient erythropoiesis and hemolysis. For this reason, patients receive periodic blood transfusions to keep hemoglobin levels compatible with life. Blood transfusions, together with increased intestinal iron absorption and chronic hemolysis, determinate progressive iron overload in different tissues with secondary hemochromatosis. The heart is a target organ in the pathologic process, and it has been previously shown that iron-mediated cardiomyopathy is the leading cause of death and morbidity in patients with TM.
Recent advances in echocardiography, especially two-dimensional speckle-tracking imaging, have created the possibility for a better understanding of cardiac pathophysiology with the assessment of new parameters of cardiac function such as left ventricular (LV) torsional dynamics. Torsional change of the left ventricle is defined as the “wringing motion” of the heart. Myocardial fiber orientation changes progressively from the endocardial to the epicardial layer in a spiral manner. Fibers that are subendocardial show a right-handed helical disposition, which gradually tends to become left-handed in the subepicardial layer. In particular, the subepicardial region mainly determines the direction of twist and contributes to ventricular rotation, whereas the subendocardium makes a prevalent contribution to the longitudinal component of LV deformation. This particular anatomic disposition creates two opposite isochronal rotational directions during systole. In fact, when seen from the apex, the active contraction of basal fibers determines a clockwise rotation, while a counterclockwise rotation is predominant at the apex. Torsional dynamics create matrix deformation, which results in potential energy storage. Subsequently, the passive relaxation or untwisting of these fibers, determined in large part by the release of previous stored energy, creates suction for optimal diastolic blood filling. In particular, the sudden detention of the apex creates an intraventricular pressure gradient that contributes to optimal diastolic function together with chamber compliance.
Different studies have previously focused on longitudinal deformation in thalassemic heart disease, identifying regional alterations, but few studies have been performed to asses LV torsion. Accordingly, the aim of this study was to evaluate LV torsion in a group of asymptomatic patients with TM and its relationship with iron overload.
Methods
Study Population
From November 2010 to March 2011, 27 patients affected by TM, receiving long-term blood transfusions every 3 weeks and undergoing iron chelation therapy with desferoxamine, were prospectively enrolled. Twenty-seven healthy control subjects, matched for age and sex, with normal ferritin levels were also studied. Exclusion criteria in both groups were hypertension, diabetes mellitus, heart failure symptoms, ejection fraction < 55%, mild or severe valvulopathy, presence of atrial fibrillation or flutter, and a history of ischemic heart disease.
In all patients with TM, ferritin levels were measured, and a subgroup underwent cardiac magnetic resonance (CMR) imaging to assess myocardial iron overload by means of T2* value. The flow diagram of the study is shown in Figure 1 .

Conventional Echocardiography
All patients underwent standard echocardiographic examinations in the supine position using a GE Vivid 7 ultrasound system (GE Vingmed Ultrasound AS, Horten, Norway) equipped with an S3 multifrequency probe. Parameters evaluated from M-mode echocardiography were: LV diastolic and systolic diameters, diastolic interventricular septal and posterior wall thicknesses, and LV mass. Left atrial volume was obtained using two-dimensional echocardiography from two-chamber and four-chamber views. All measures were performed according to the recommendations of the American Society of Echocardiography. LV end-systolic and diastolic volumes, LV stroke volume, and LV ejection fraction were analyzed offline using a semiautomatic measurement tool for global ejection fraction evaluation (see “Speckle-Tracking Analysis and Torsion” for further details). Left atrial volume and LV mass and volumes were indexed to body surface area.
Pulsed-wave Doppler imaging was performed by placing the sample volume at the tip level of the mitral leaflets in the apical four-chamber view, obtaining in this way peak mitral inflow early diastolic velocity (E), late diastolic atrial filling velocity (A), their ratio (E/A), and E-wave deceleration time. Doppler tissue imaging (DTI) analysis was performed by placing the sample volume of pulsed DTI at the septal and lateral mitral annulus, obtaining peak systolic myocardial velocity (S′), early (E′) and late (A′) diastolic myocardial velocities, LV isovolumetric relaxation time, LV ejection time, and LV isovolumetric contraction time. All DTI parameters were evaluated by averaging the respective values at the septal and lateral mitral annulus. The LV myocardial performance index was calculated as the sum of LV isovolumetric contraction time and LV isovolumetric relaxation time divided by LV ejection time, and the ratio between E and averaged E′ (E/E′) was then used as a surrogate for LV filling pressure. A level III–certified echocardiologist, blinded to clinical data, made all measurements.
Two-dimensional grayscale loops, from parasternal short-axis views (basal and apical level) and apical views (four and two chambers) were carefully acquired, for three consecutive cycles, at 80 frames/sec. The basal plane was identified by the mitral valve tips, while the apical segment at the underside of the papillary muscles, where the right ventricular cavity was not visible and proximal to LV luminal end-systolic obliteration. Images were digitally recorded for subsequent offline analysis.
Speckle-Tracking Analysis and Torsion
Offline analysis was performed using commercially available speckle-tracking software (EchoPAC version 110.0.0; GE Healthcare, Milwaukee, WI). An automatic ejection fraction tool, recently validated in clinical practice, was used for the evaluation of ventricular volumes and ejection fraction. The tool is derived from a speckle-tracking echocardiographic algorithm, which tracks and calculates myocardial tissue deformation on the basis of two-dimensional grayscale loops. Apical four-chamber and two-chamber views were analyzed in each subject. Three points were placed (two annular points at the base and one at the apex) at the endocardial border and automatically displayed by the system on a frame on which it was clearly visible. After that, the endocardial border was automatically traced, visually controlled, and approved for calculation. Subsequent to the validation process, the system calculated ventricular volumes and ejection fraction according to two-dimensional Simpson’s formula.
To assess LV rotation, the endocardial border was traced in end-diastole, and the software then automatically detected the region of interest (ROI) from the epicardial to the endocardial layer. Cycles with similar heart rates were selected for rotational measurement at the base and apex; the program did not allow speckle-tracking echocardiographic analysis if the heart rates at the two levels were significantly different. Attention was paid to ensure that the entire myocardium was inside the ROI for optimal tracking. The software then divided the ROI into six segments according to anatomic position and performed speckle-tracking analysis. Segments with poor tracking quality were excluded from the analysis. Rotation (angular displacement; see Figure 2 ) and rotation rate (the velocity of angular displacement; see Figure 3 ) were automatically calculated at the base and at the apex of the left ventricle. The average values of rotation and rotation rate among the six segments were considered as the global values for the respective sections. LV twist was automatically calculated as the net difference between the rotation of the apex and the rotation of the base in systole. LV torsion was then obtained as the ratio of LV twist for the end-diastolic longitudinal diameter of the ventricle. Time to peak rotation of the base and the apex was included in the analysis. Diastolic untwist and untwisting rate, at the base and the apex, were also evaluated. All rotational parameters were obtained according to previously published studies. Longitudinal strain, strain rate, and displacement from apical four-chamber and two-chamber views of the left ventricle were analyzed using the procedure described for rotational analysis and for the correct identification and positioning of the ROI.


Statistical Analysis
Parameters are expressed as mean ± SD. Continuous variables were compared between the two groups using Student’s t tests and dichotomous variables using χ 2 tests. All tests were two tailed. Intraobserver and interobserver variability is expressed using coefficients of variability and intraclass correlation coefficients with 95% confidence intervals. The coefficient of variability were calculated as the absolute difference of paired measurements as a percentage of their mean. Relationships between parameters of cardiac function, traditional and rotational, and previously described parameters of iron overload (ferritin levels and T2* values) were assessed using Pearson’s method. P values < .05 were considered statistically significant.
Results
Clinical data are presented in Table 1 . Patients with TM had significantly lower body surface areas (1.58 ± 0.19 vs 1.75 ± 0.21 m 2 , P = .003) and systolic blood pressures (102.0 ± 12.6 vs 109.4 ± 11.6 mm Hg, P = .029). Patients with TM had iron overload with elevated serum ferritin levels (1,797.04 ± 1,330.5 ng/mL), and hemoglobin levels before and after trasfusion were 9.79 ± 0.59 and 12.04 ± 0.63 g/dL, respectively. Subjects in the control group all had ferritin levels in the normal range (an inclusion criterion for this group). In the subgroup of patients evaluated by means of CMR imaging, the mean T2* value was 27.18 ± 12.34 msec, with five patients showing myocardial iron overload (T2* < 20 msec).
Variable | Patients with TM ( n = 27) | Healthy subjects ( n = 27) | P |
---|---|---|---|
Age (y) | 29.7 ± 8.4 | 30.3 ± 6.0 | .780 |
Men/women | 11/16 | 12/15 | .783 |
Body surface area (m 2 ) | 1.58 ± 0.19 | 1.75 ± 0.21 | .003 |
Body mass index (kg/m 2 ) | 22.9 ± 3.2 | 22.9 ± 3.3 | .947 |
Heart rate (beats/min) | 72.4 ± 8.4 | 71.3 ± 9.6 | .648 |
Systolic blood pressure (mm Hg) | 102.0 ± 12.6 | 109.4 ± 11.6 | .029 |
Diastolic blood pressure (mm Hg) | 65.9 ± 6.5 | 67.0 ± 6.1 | .520 |
All subjects had normal ejection fractions and other classic parameters of systolic and diastolic function. Although average values were within the normal range, patients with TM showed significantly lower S′ values and E′ values with increased E/E′ ratios and indexed left atrial volumes compared with healthy controls ( Table 2 ).
Variable | Patients with TM ( n = 27) | Healthy subjects ( n = 27) | Range | P |
---|---|---|---|---|
LV IVSd (mm) | 7.4 ± 1.3 | 7.6 ± 1.2 | 6–10 | .633 |
LV Dd (mm) | 49.2 ± 4.5 | 48.4 ± 4.5 | 41–57 | .536 |
LV Ds (mm) | 30.1 ± 3.8 | 29.4 ± 4.3 | 24–38 | .526 |
LV PWd (mm) | 7.7 ± 1.7 | 7.5 ± 1.3 | 5–12 | .622 |
RWT | 0.32 ± 0.06 | 0.31 ± 0.05 | 0.21–0.42 | .890 |
LV mass index (g/m 2 ) | 77 ± 17 | 70 ± 16 | 50–115 | .117 |
LV EDV index (mL/m 2 ) | 44 ± 10 | 41 ± 9 | 30–62 | .178 |
LV ESV index (mL/m 2 ) | 18 ± 4 | 17 ± 4 | 11–29 | .375 |
LV SV index (mL/m 2 ) | 27 ± 7 | 24 ± 6 | 17–37 | .138 |
LV EF (%) | 61 ± 6 | 59 ± 4 | 55–77 | .209 |
LAVi (mL/m 2 ) | 25 ± 7 | 21 ± 4 | 13–34 | .022 |
E/A ratio | 1.8 ± 0.4 | 1.7 ± 0.5 | 1.0–2.8 | .400 |
E-wave deceleration time (msec) | 169 ± 21 | 178 ± 25 | 127–217 | .159 |
S′ (cm/sec) | 7.6 ± 1.4 | 8.6 ± 1.7 | 8–13 | .030 |
E′ (cm/sec) | 12.8 ± 2.4 | 14.3 ± 2.4 | 9–19 | .025 |
A′ (cm/sec) | 6.7 ± 1.9 | 7.3 ± 2.6 | 4–15 | .350 |
E/E′ ratio | 7.3 ± 1.4 | 6.2 ± 1.1 | 4.7–10.1 | .003 |
LV MPI | 0.48 ± 0.11 | 0.47 ± 0.08 | 0.38–0.56 | .740 |
Longitudinal deformation analysis is shown in Table 3 . A significantly lower mean value for two-chamber displacement was identified in patients with TM ( P = .041).
Longitudinal | Patients with TM ( n = 27) | Healthy subjects ( n = 27) | P |
---|---|---|---|
Strain (%) | |||
Four chamber | −21.2 ± 3.0 | −21.4 ± 3.4 | .829 |
Two chamber | −21.5 ± 3.6 | −21.5 ± 3.5 | .532 |
Strain rate (1/sec) | |||
Four chamber | |||
S wave | −1.1 ± 0.2 | −1.1 ± 0.2 | .156 |
E wave | 1.8 ± 0.4 | 1.7 ± 0.5 | .454 |
A wave | 0.7 ± 0.2 | 0.7 ± 0.2 | .653 |
Two chamber | |||
S wave | −1.1 ± 0.3 | −1.0 ± 0.2 | .872 |
E wave | 1.5 ± 0.4 | 1.6 ± 0.4 | .551 |
A wave | 0.7 ± 0.2 | 0.6 ± 0.2 | .596 |
Displacement (cm) | |||
Four chamber | 3.5 ± 1.5 | 3.6 ± 1.4 | .773 |
Two chamber | 3.7 ± 1.8 | 4.6 ± 0.8 | .042 |
LV rotational parameters are shown in Table 4 . Compared with controls, patients had significantly reduced systolic apical rotation ( P = .006), LV twist ( P = .002), and LV torsion ( P = .001). Rotational velocity analysis showed significantly reduced velocity in systolic and diastolic rotation at the apical level ( P = .003 and P = .011, respectively) in the TM group. Twisting and untwisting rates were significantly decreased in patients ( P = .001 and P = .002, respectively). No difference emerged in time to peak rotation at the base and the apex of the left ventricle. Compared with healthy controls, patients with T2* values >20 msec had preserved rotational function, while those with T2* < 20 msec showed significantly lower mean values of LV peak basal systolic rotation (−3.1 ± 1.4° vs −6.2 ± 2.6°, P = .016), LV peak apical systolic rotation (3.4 ± 1.3° vs 6.4 ± 3.1°, P = .045), LV twist (4.8 ± 2.5 vs 10.9 ± 4.9 deg, P = .012), and LV torsion (0.6 ± 0.2°/cm vs 1.4 ± 1.6°/cm, P = .010). LV torsion was negatively related to ferritin levels ( r = −0.47, P = .013) and directly to T2* values ( r = 0.64, P = .007) ( Figures 4 A and 4 B). T2* values were also significantly related to LV twist ( r = 0.60, P = .014). LV twist rate was negatively related to ferritin levels ( r = −0.51, P = .009), and a significant relationship was also found between ferritin and peak basal systolic and diastolic rotation rate ( r = 0.49, P = .012, and r = −0.48, P = .014, respectively). A trend toward significance was identified between T2* values and ferritin with untwisting rate (see Figures 5 A and 5 B). Untwisting rate was significantly related to the E′ value in the overall population ( r = −0.30, P = .026). Traditional parameters of systolic and diastolic function were not correlated with measures of iron overload.
Parameter | Patients with TM ( n = 27) | Healthy subjects ( n = 27) | P |
---|---|---|---|
Peak basal systolic rotation (°) | −5.0 ± 3.0 | −6.2 ± 2.6 | .125 |
Peak basal diastolic rotation (°) | 2.8 ± 1.6 | 2.8 ± 1.6 | .966 |
Peak apical systolic rotation (°) | 4.3 ± 2.2 | 6.4 ± 3.1 | .006 |
Peak apical diastolic rotation (°) | −2.1 ± 1.4 | −2.4 ± 1.6 | .557 |
Peak basal systolic rotation rate (°/sec) | −52.6 ± 21.9 | −63.3 ± 20.8 | .071 |
Peak basal diastolic rotation rate (°/sec) | 53.8 ± 16.5 | 64.0 ± 26.0 | .092 |
Peak apical systolic rotation rate (°/sec) | 47.1 ± 14.8 | 63.2 ± 22.2 | .003 |
Peak apical diastolic rotation rate (°/sec) | −47.3 ± 14.9 | −61.8 ± 24.6 | .011 |
Peak LV twist (°) | 7.2 ± 3.0 | 10.9 ± 4.9 | .002 |
Peak LV torsion (°/cm) | 0.9 ± 0.4 | 1.4 ± 0.6 | .001 |
Peak LV Twist rate (degree/sec) | 59.2 ± 22.9 | 79.4 ± 24.7 | .003 |
Peak LV Untwisting rate (degree/sec) | −64.4 ± 20.7 | −88.1 ± 29.4 | .001 |
Time to basal rotation peak (msec) | 303 ± 91 | 352 ± 87 | .058 |
Time to apical rotation peak (msec) | 351 ± 96 | 390 ± 95 | .155 |
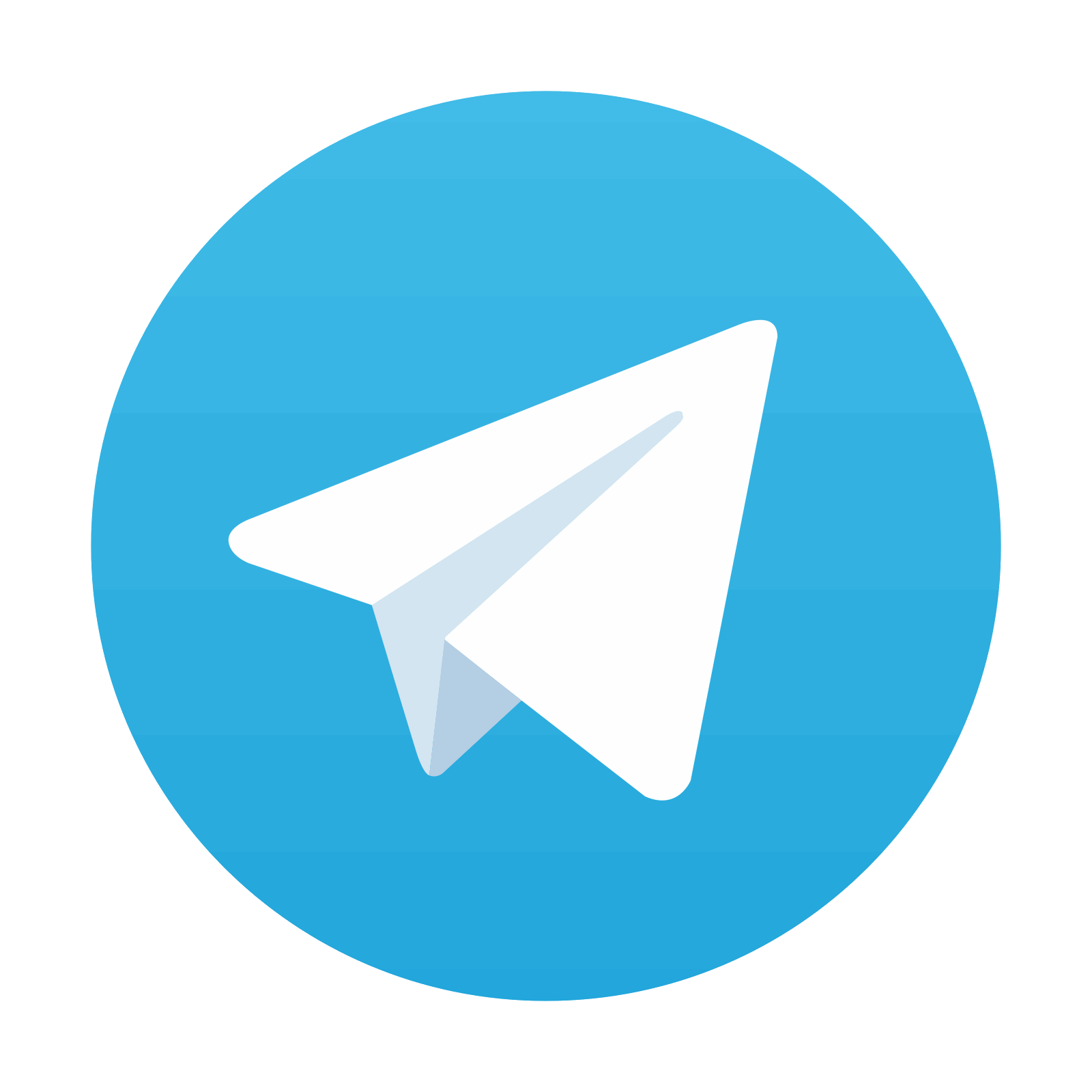
Stay updated, free articles. Join our Telegram channel

Full access? Get Clinical Tree
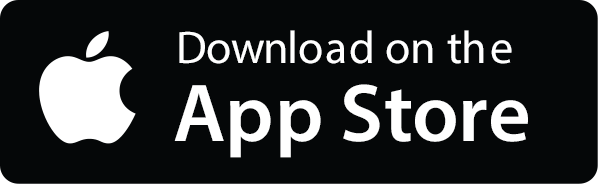
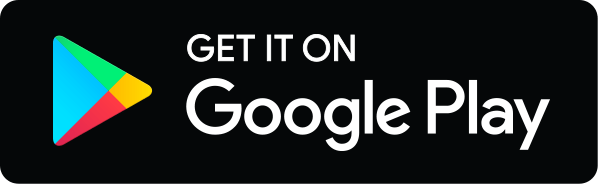
