Background
Ligation of a patent ductus arteriosus (PDA) in preterm infants causes profound hemodynamic changes that can result in low cardiac output syndrome and hypotension. The effect of PDA ligation on left ventricular myocardial function has not been studied using tissue Doppler and myocardial deformation imaging, mainly because of the limited validation of these methods in preterm infants. The primary objective of the present study was to determine the feasibility and reliability (intraobserver and interobserver variability) of tissue Doppler and myocardial deformation imaging for evaluating myocardial function in preterm infants undergoing surgical PDA ligation. Additionally, we sought to study the immediate effect of surgical ligation on the left ventricular tissue Doppler and strain measurements in the first 24 hours after surgery.
Methods
Echocardiography was performed in 19 preterm infants before, 1 hour after, and 18 hours after PDA ligation born at 24–29 weeks of gestation. The tissue Doppler velocities of the lateral tricuspid and lateral and septal mitral valve annuli were obtained. The global and regional longitudinal peak systolic strain values were determined using two-dimensional speckle tracking echocardiography. The results of the three measurement points were compared using repeated measures analysis of variance. The intra- and interobserver variability was assessed using the intraclass correlation coefficient and Bland-Altman analysis.
Results
The median gestational age was 25.0 weeks (interquartile range 24.9–25.9) and the birth weight was 750 g (interquartile range 600–810). For the global longitudinal strain, the intraobserver intraclass correlation coefficient was 0.92 (95% CI, 0.78–0.97, P < .001), and the interobserver intraclass correlation coefficient was 0.93 (95% CI, 0.66–0.98, P < .001). Bland-Altman analysis showed no significant bias between the two observers, with good agreement. The systolic and diastolic tissue Doppler velocities of the mitral valve decreased significantly immediately after ligation and remained significantly lower than the preoperative levels at 18 hours. Also, the global longitudinal strain values significantly decreased 1 hour after the procedure (global longitudinal strain before −19.7% ± −3.8% vs −11.5% ± −3.5%; P = .001) but had significantly improved 18 hours after the procedure (−15.1% ± −2.9%, P = .01).
Conclusions
The present study has shown the feasibility and reliability of using tissue Doppler and strain imaging in premature infants with a hemodynamically significant PDA. Significant changes in myocardial function were observed immediately after PDA ligation, suggesting important changes in myocardial performance immediately after ductal ligation.
Assessing myocardial function in preterm infants is challenging because of the many different factors influencing myocardial function and the lack of well-standardized echocardiography techniques to assess myocardial performance in this population. Intrinsically, the preterm myocardium is different from the adult myocardium, with impaired systolic functional reserve and different diastolic properties. Additionally, the myocardium is exposed to significant changes in the loading conditions in the transitional period immediately after birth. A patent ductus arteriosus (PDA) is a common finding in preterm infants, resulting in chronic left ventricular (LV) volume loading. Preterm infants are at high risk of developing hemodynamic compromise after PDA ligation owning to the sudden change in the loading conditions. This is usually manifested clinically by hypotension and increasing oxygen requirements occurring 6–12 hours postoperatively. There has been recent interest in better characterizing these changes and evaluating the myocardial functional adaptations using echocardiography. Our group has studied the conventional parameters affecting cardiac function in preterm infants. We observed a decrease in fractional shortening and LV output (LVO) immediately after surgical ligation. Our data also suggest that LVO less than 200 mL/kg/min could be used to select those infants who might benefit from early administration of milrinone treatment. Tissue Doppler imaging (TDI) and myocardial deformation imaging using speckle tracking echocardiography are newer ultrasound modalities that have been applied to study adult and pediatric heart disease, but very few data are available on their use in preterm infants. Both techniques have the advantage of directly viewing the myocardium and measuring regional and global myocardial function. Previous studies have demonstrated acceptable accuracy and reliability in assessing regional myocardial function in children and adults. Both speckle tracking echocardiography and TDI have demonstrated the ability to detect early myocardial dysfunction in different diseases. The application of TDI and speckle tracking echocardiography in the preterm population has not been extensively explored, and some initial data have suggested a problem with the reliability of strain measurements in this population. The aim of the present study was to assess the feasibility and reliability of tissue Doppler velocities and longitudinal strain measurements in preterm infants with a large PDA. Additionally, we sought to serially study the acute effects of PDA ligation on these measurements to better understand the effect of acute load changes on the preterm myocardium.
Methods
Inclusion and Exclusion Criteria
All preterm neonates with a gestation of 24–30 weeks admitted to the Hospital for Sick Children for PDA ligation were considered eligible for inclusion. The referrals for PDA ligation were triaged according to the clinical and echocardiographic findings using the PDA staging system proposed by McNamara and Sehgal. The criteria used to define a hemodynamically significant PDA included a transductal diameter larger than 1.5 mm, unrestrictive flow through the duct with low flow Doppler velocities, predominant left-to-right flow, and clinical signs of pulmonary overcirculation or systemic hypoperfusion, or both. The parents of all eligible infants were provided with an information sheet, and all the parents provided informed written consent. Infants with major congenital abnormalities and cardiac lesions other than a PDA or a patent foramen ovale were excluded from the present study. The infants were treated according our standard clinical treatment protocols. None of the infants received inotropic support before surgery. In addition, no infants required inotropes in the immediate postoperative period. According to our protocols, the infants were given milrinone if the LVO was less than 200 mL/kg/min on a 1-hour postoperative echocardiogram. This was required in 12 (63%) of 19 infants. The infants admitted to our neonate intensive care unit for surgical duct ligation are generally referred back to their original neonate intensive care unit for additional neonatal care within 24 hours after the surgery. This limited the clinical and echocardiographic follow-up data available after 24 hours. The institutional research ethics board approved the present study.
Clinical Data Collection
The clinical details collected included the infants’ gestational age and weight at birth and at ligation. The gender and mode of delivery were also collected. All data, including the baseline hemodynamic, respiratory characteristics, and echocardiograms, were obtained at three different points: 4–6 hours preoperatively, 1 hour postoperatively, and 16–18 hours postoperatively. The clinical data collected included the heart rate, blood pressure, oxygen requirement, oxygen saturation, mean airway pressure, and pH.
Echocardiography Measurements
At the three intervals, all patients underwent an echocardiographic study using a Vivid 7 ultrasound scanner (GE Medical, Milwaukee, WI) with a 10-MHz neonatal probe. A standardized functional protocol was used, and the measurements were generally performed according to recently published guidelines. The imaging data were digitally stored, and off-line analysis was performed on a dedicated workstation (EchoPAC, GE Medical). The LV dimensions were measured, and the fractional shortening was calculated using M-mode measurements from a parasternal long-axis view at the level just distal to the mitral valve leaflets. This allowed serial measurements of the LV dimensions at exactly the same level, with a high temporal resolution. The ejection fraction was measured using the biplane Simpson method from the apical four- and two-chamber views. The left atrial/aortic root ratio was measured using the M-mode measurements. The LVO was assessed by obtaining the pulsed wave Doppler blood velocity in the aortic root and measuring the diameter of the aortic root using two-dimensional echocardiography. The LVO was calculated using the formula: LVO = (aortic circumferential surface area × velocity − time integral × heart rate)/weight. The circumferential surface area was calculated as (aortic root diameter) 2 × 0.785. The normal values range from 170 to 350 mL/kg/min in the neonatal population. The right ventricular (RV) output was obtained by measuring the velocity of the blood in the RV outflow tract using pulsed wave Doppler and determining the cross-sectional area at the pulmonary valve level using two-dimensional imaging. The LV wall stress was calculated using the mean arterial pressure measurements. The tissue Doppler velocities were obtained from the apical four-chamber view. A pulsed wave Doppler sample gate of 1–2 mm was used and was positioned at the lateral tricuspid and lateral and septal mitral annuli. Off-line analysis used the average readings of the S′, E′, and A′ myocardial velocities in 2–3 cardiac cycles. On the tissue Doppler traces (lateral mitral annulus), we measured the isovolumic contraction time (interval between the end of the A′ wave to the beginning of the S′ wave) and the isovolumic relaxation time (interval from the end of the S′ wave to the beginning of the E′ wave). The isovolumic contraction and relaxation peak velocities were also measured ( Figure 1 ). The myocardial performance index was calculated using the formula: isovolumic relaxation time + isovolumic contraction time/LV ejection time (defined as the duration of the S′ wave).

For longitudinal strain analysis, gray-scale images were recorded from the apical four-, two- and three-chamber views. A frame rate of 80–100 frames/sec was used for storage and analysis. The images were optimized to visualize the myocardial walls. The analysis was performed using the two-dimensional strain program (EchoPAC, GE Medical). In brief, the endocardial border was manually traced at end systole. Tracking was automatically performed, and the analysis was accepted after visual inspection and when the software indicated adequate tracking. If tracking was suboptimal, the endocardial border was retraced. If satisfactory tracking was not accomplished within 5 minutes, the nontracking segments were excluded from the analysis. The end-systolic strain values were measured at aortic valve closure. The global LV longitudinal strain was calculated by averaging all the measured segmental values. The average longitudinal strain of the different LV walls (septal, lateral, inferior, posterior, anterior, and anteroseptal) was calculated by averaging the basal, mid, and apical end-systolic strain segmental values in each wall.
Statistical Analysis
Continuous variables were tested for normality using a histogram illustration of the data and the Shapiro-Wilk test for normality. Normally distributed variables are presented as the mean ± SD, and skewed data are presented using the median and interquartile range. The hemodynamic, respiratory, and echocardiographic parameters were compared across the three points using one-way analysis of variance with repeated measures. The Greenhouse-Geisser adjusted P value was used if the assumption of sphericity was violated. Post hoc pairwise comparisons among the three points were conducted using the Bonferroni adjustment. Skewed data were compared using the Kruskal-Wallis test. The inter- and intraobserver variability of the strain measurements and TDI velocities were assessed using 15 studies. The intraobserver variability was assessed by 1 investigator (A.K.) performing off-line analysis on the same patients 4 weeks apart to reduce recall bias. The interobserver variability was assessed by a second investigator (A.J.), who was unaware of the previous results, performing the investigation on the same set of 15 infants. The interobserver and intraobserver agreement was assessed using the intraclass correlation coefficient, version 2.1. Additionally, agreement between investigators was demonstrated using the Bland-Altman method by calculating the bias (mean difference) and the 95% limits of agreement (1.96 SD around the mean difference). For all Bland- Altman plots, the percentage of difference (the difference between two measurements divided by the average of the two measurements multiplied by 100) was used. We accepted P < .05 as significant. The statistical analysis was performed using SPSS, version 19 (SPSS, Chicago, IL).
Results
Nineteen preterm infants were enrolled in the present study from February 2011 to March 2012. Their median gestational age at birth was 25.0 weeks (interquartile range [IQR] 24.9–25.9), and their median birth weight was 750 g (IQR 600–810). The infants underwent the procedure on day 25 (IQR 20–34) of life at a median gestation and weight of 28.9 weeks (IQR 28.0–30.9) and 948 g (IQR 810–1,039), respectively. Tissue Doppler velocities and conventional echocardiographic measurements could be obtained in all infants. Strain measurements could not be obtained in 4 of 57 studies.
Baseline Hemodynamic and Respiratory Characteristics
The serial hemodynamic and respiratory data are summarized in Table 1 . No significant differences were found in the heart rate, systolic blood pressure, or mean blood pressure across the three measurement points. The mean diastolic blood pressure was significantly greater 1 and 18 hours postoperatively compared with the preoperative value. The mean airway pressure and oxygen requirement remained similar across the three points. After PDA ligation, 12 infants (63%) received milrinone for low cardiac output on the 1 hour echocardiogram; 6 (32%) received a blood transfusion, 4 (21%) developed postligation hemodynamic instability about 8 hours after surgery (postligation cardiac syndrome), and 1 developed a significant chylothorax and subsequently died 4 days after surgery.
Parameter | Preoperative | 1 Hour postoperatively | 18 Hours postoperatively | P value |
---|---|---|---|---|
Heart rate (beat/min) | 159 ± 13 | 148 ± 14 | 155 ± 19 | .07 |
SBP (mm Hg) | 59 ± 8 | 58 ± 11 | 57 (14) | .7 |
DBP (mm Hg) | 26 ± 4 ∗ † | 37 ± 7 ∗ | 33 ± 9 † | < .001 |
Mean BP (mm Hg) | 39 ± 5 | 44 ± 8 | 43 ± 11 | .08 |
MAP (cm H 2 O) | 9.9 ± 1.4 | 9.3 ± 2.1 | 10.2 ± 3.0 | .4 |
pH | 7.32 ± 0.05 | 7.35 ± 0.08 | 7.31 ± 0.07 | .2 |
∗ Significant pairwise comparisons.
Feasibility and Reliability
A total of 57 longitudinal strain assessments were attempted in 19 infants (19 studies for each point). In 16 (84%) of 19 infants, we were able to obtain strain measurements for all three points. In 3 infants, the strain measurements were not possible at least one measurement point because of poor image quality. An additional 10 individual segments (of 1,026 individual segments tracked) were not included in the calculation of global strain because the software failed to adequately track them. The intra- and interobserver variability analysis, including the percentage bias, 95% limits of agreements, and intraclass correlation coefficient, for the tissue Doppler velocities and global longitudinal strain measurements are summarized in Table 2 . For the different measurements, no significant bias was observed, with narrow limits of agreement. Figure 2 presents the Bland-Altman plots for the intra- and interobserver variability of the global longitudinal strain measurements.
Variable | Intraobserver | Interobserver | ||||
---|---|---|---|---|---|---|
Mean ± SD | Percent bias (95% LOA) | ICC (95% CI, P value) | Mean ± SD | Percent bias (95% LOA) | ICC (95% CI, P value) | |
Mitral valve | ||||||
S′ | 3.70 ± 1.42 | 3.26 (−8.13–14.65) | 0.99 (0.94–1.00, < .001) | 3.80 ± 1.23 | −0.93 (−16.14–14.28) | 0.72 (0.37–0.90, .001) |
E′ | 4.47 ± 3.76 | −3.00 (−21.78–15.78) | 0.99 (0.98–1.00, < .001) | 4.73 ± 3.67 | −3.38 (−31.58–24.82) | 0.90 (0.74–0.97, < .001) |
A′ | 4.39 ± 2.42 | −5.3 (−31.21–20.61) | 0.98 (0.94–0.99, < .001) | 4.33 ± 2.50 | 9.94 (−13.72–33.60) | 0.98 (0.91–0.99, < .001) |
IVS | ||||||
S′ | 3.79 ± 0.98 | 1.94 (−11.40–15.32) | 0.97 (0.90–0.99, < .001) | 3.75 ± 0.90 | −2.14 (−33.36–29.08) | 0.90 (0.74–0.97, < .001) |
E′ | 4.23 ± 2.39 | 1.08 (−16.78–18.94) | 0.99 (0.97–1.00, < .001) | 4.37 ± 2.42 | 0.00 (−28.36–28.36) | 0.82 (0.56–0.94, < .001) |
A′ | 5.87 ± 1.49 | −3.22 (−18.29–11.85) | 0.97 (0.89–0.99, < .001) | 5.70 ± 1.38 | 6.93 (−24.05–37.91) | 0.70 (0.27–0.90, .003) |
TV | ||||||
S′ | 5.97 ± 1.79 | −0.35 (−9.93–9.23) | 0.98 (0.96–0.99, < .001) | 6.18 ± 1.82 | −7.72 (−28.44–13.00) | 0.95 (0.74–0.99, < .001) |
E′ | 7.44 ± 3.60 | −1.98 (−15.83–11.87) | 0.99 (0.98–1.00, < .001) | 7.31 ± 3.65 | 5.01 (−23.58–33.60) | 0.94 (0.83–0.98, < .001) |
A′ | 8.25 ± 1.44 | 0.42 (−10.92–11.74) | 0.94 (0.74–0.99, < .001) | 8.27 ± 1.41 | 3.21 (−10.11–16.53) | 0.91 (0.61–0.98, < .001) |
GLS | −18.25 ± −4.61 | 0.1 (−20.9–21.1) | 0.92 (0.78–0.97, < .001) | −17.16 ± −4.42 | 6.0 (−8.00–20.00) | 0.93 (0.66–0.98, < .001) |
Conventional Echocardiographic Parameters
The serial measurements of the conventional echocardiographic parameters are listed in Table 3 . As expected, the LV dimensions decreased immediately after PDA ligation but did not change further within the next 24 hours. The LV end-diastolic diameter was significantly larger in the preoperative period than at 1 hour postoperatively ( P < .001) and 18 hour postoperatively ( P = .03). Similarly, the left atrial/aortic root ratio and shortening fraction were both greater in the preoperative period than in both postoperative periods. The LVO values were significantly different across all three points. No significant differences were seen in the RV output, and no significant changes in LV wall stress could be found among the different points.
Parameter | Preoperative | 1 Hour postoperatively | 18 Hours postoperatively | P value |
---|---|---|---|---|
LVEDD (mm) | 16.4 ± 2.4 ∗ † | 12.9 ± 2.8 ∗ | 12.3 ± 4.6 † | .001 |
LVPW diastolic thickness (mm) | 2.1 ± 0.7 ∗ | 2.7 ± 0.5 ∗ | 2.5 ± 0.5 | .01 |
LV length (mm) | 21.4 ± 2.6 ∗ † | 18.8 ± 2.1 ∗ | 19.7 ± 2.9 † | < .001 |
LV sphericity index | 0.76 ± 0.12 † | 0.70 ± 0.17 | 0.63 ± 0.25 † | .15 |
Left atrial/aortic ratio | 2.48 ± 0.38 ∗ † | 1.79 ± 0.25 ∗ | 1.9 ± 0.36 † | < .001 |
Shortening fraction (%) | 36.9 ± 5.6 ∗ † | 23.6 ± 7.4 ∗ | 27.3 ± 6.5 † | < .001 |
Simpson’s ejection fraction (%) | 66.6 ± 6.9 ∗ † | 54.5 ± 11.1 ∗ | 57.1 ± 10.9 † | < .001 |
LV wall stress (g/cm 2 ) | 20.8 ± 5.4 | 23.4 ± 7.4 | 20.9 ± 8.6 | .24 |
LVO mL/kg/min | 420 ± 137 ∗ | 167 ± 56 ∗ | 239 ± 76 ∗ | < .001 |
RVO (mL/kg/min) | 256 ± 99 | 213 ± 77 | 246 ± 98 | .3 |
∗ Significant difference ( P < .05) between preoperative and 1-hour postoperative measurements.
† Significant difference between preoperative and 18-hour postoperative measurements.
Tissue Doppler Velocities
The tissue Doppler velocities of the mitral and tricuspid annuli and the basal interventricular septum are listed in Table 4 . A significant decrease occurred in all mitral valve annulus tissue Doppler velocities (S, E′, and A′) after PDA ligation, with a significant improvement after 18 hours (except for the septal). The tricuspid value annular velocities demonstrated a smaller amount of change across the three points. The isovolumic contraction and relaxation times had significantly increased at 1 hour postoperatively and had persisted on the 18-hour postoperative scan compared with preoperatively. The isovolumic contraction velocity increased significantly after ligation and had significantly decreased by 18 hours postoperatively.
Preoperative | 1 Hour postoperatively | 18 Hours postoperatively | P value | |
---|---|---|---|---|
Tissue Doppler velocity (cm/sec) | ||||
MV annulus | ||||
S | 5.3 ± 0.9 ∗ † | 3.3 ± 0.9 ∗ | 4.0 ± 0.8 † | < .001 |
E | 8.9 ± 4.2 ∗ † | 3.7 ± 1.5 ∗ | 4.2 ± 1.3 † | < .001 |
A | 9.6 ± 3.2 ∗ † | 5.8 ± 2.6 ∗ | 5.1 ± 1.5 † | < .001 |
IVS | ||||
S | 5.1 ± 0.9 ∗ | 3.3 ± 0.8 ∗ | 4.0 ± 0.6 ∗ | < .001 |
E | 6.2 ± 2.2 ∗ | 3.7 ± 1.5 ∗ | 4.8 ± 1.8 | .001 |
A | 7.1 ± 1.4 ∗ † | 5.2 ± 1.2 ∗ | 5.6 ± 1.1 † | < .001 |
TV annulus | ||||
S | 7.8 ± 1.9 ∗ † | 6.3 ± 2.0 ∗ | 6.2 ± 1.2 † | .004 |
E | 9.3 ± 3.6 ∗ † | 6.0 ± 2.3 ∗ | 6.4 ± 2.7 † | .001 |
A | 10.4 ± 2.9 ∗ | 8.3 ± 1.3 ∗ | 8.6 ± 2.1 | .01 |
MV annulus parameters | ||||
Isovolemic contraction time (msec) | 34 (6) ∗ | 57 (12) ∗ | 45 (9) ∗ | < .001 |
Isovolemic contraction wave velocity (cm/sec) | 5.8 (2.1) ∗ | 7.3 (2.5) ∗ † | 5.8 (2.4) † | .02 |
Isovolemic relaxation time (msec) | 46 (11) ∗ † | 67 (14) ∗ | 57 (11) † | < .001 |
Isovolemic relaxation wave velocity (cm/sec) | 5.2 (1.3) ∗ † | 3.6 (1.0) ∗ | 3.1 (0.7) † | < .001 |
Myocardial performance index | 0.52 (0.12) ∗ | 0.87 (0.14) ∗ | 0.70 (0.08) ∗ | < .001 |
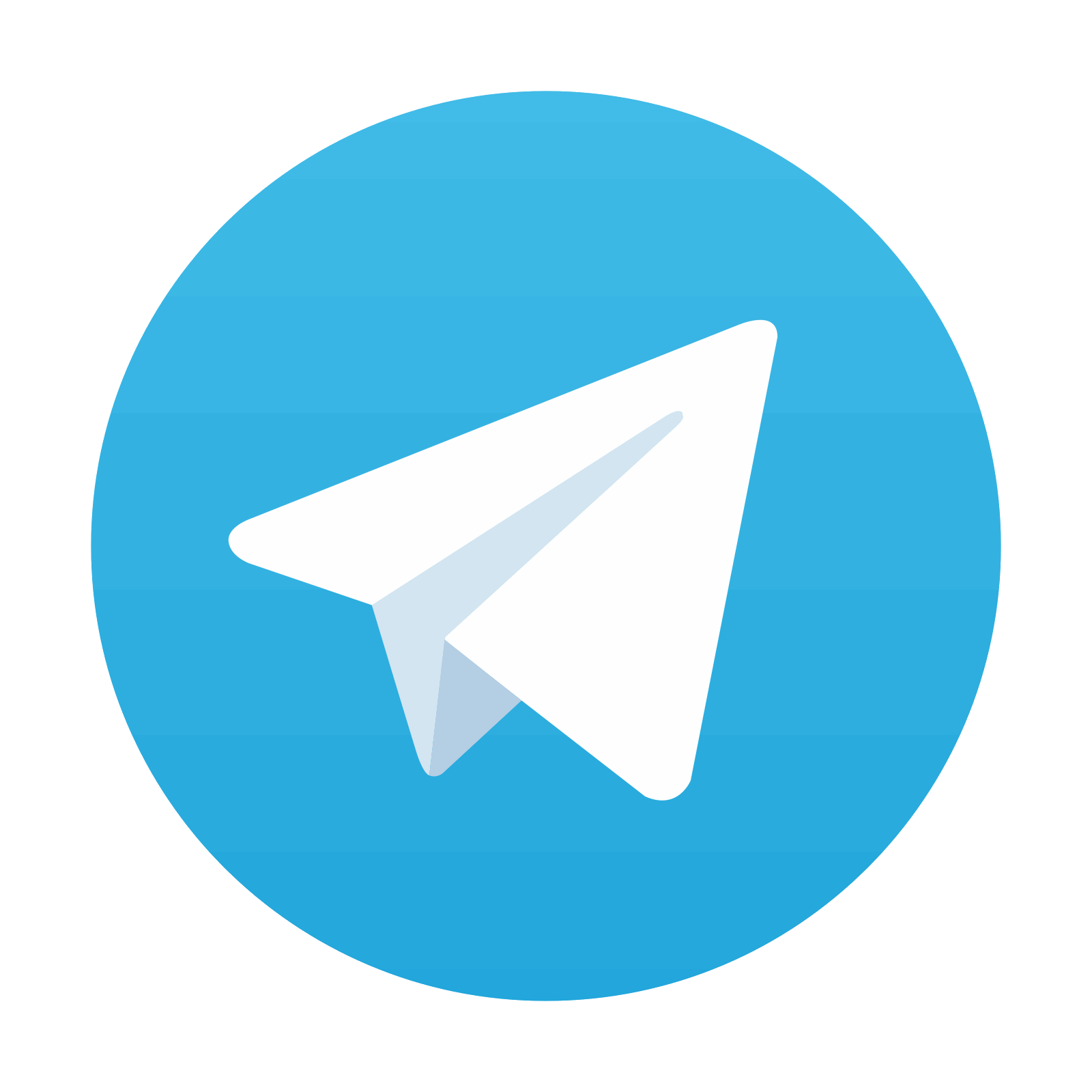
Stay updated, free articles. Join our Telegram channel

Full access? Get Clinical Tree
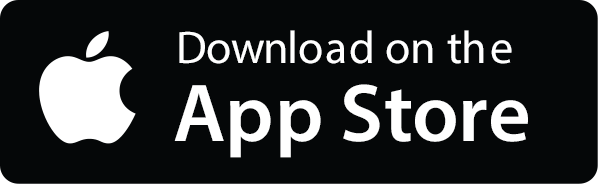
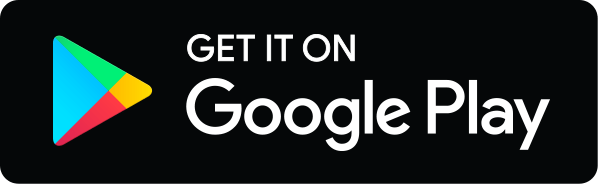
