Background
The application of left ventricular (LV) global strain by speckle-tracking is becoming more widespread, with the potential for incorporation into routine clinical echocardiography in selected patients. There are no guidelines or recommendations for the training requirements to achieve competency. The aim of this study was to determine the learning curve for global strain analysis and determine the number of studies that are required for independent reporting.
Methods
Three groups of novice observers (cardiology fellows, cardiac sonographers, medical students) received the same standardized training module prior to undertaking retrospective global strain analysis on 100 patients over a period of 3 months. To assess the effect of learning, quartiles of 25 patients were read successively by each blinded observer, and the results were compared to expert for correlation.
Results
Global longitudinal strain (GLS) had uniform learning curves and was the easiest to learn, requiring a minimum of 50 patients to achieve expert competency (intraclass correlation coefficient > 0.9) in all three groups over a period of 3 months. Prior background knowledge in echocardiography is an influential factor affecting the learning for interobserver reproducibility and time efficiency. Short-axis strain analysis using global circumferential stain and global radial strain did not yield a comprehensive learning curve, and expert level was not achieved by the end of the study.
Conclusions
There is a significant learning curve associated with LV strain analysis. We recommend a minimum of 50 studies for training to achieve competency in GLS analysis.
Highlights
- •
There are no current guidelines on training and competency for strain analysis.
- •
There is a learning curve for global longitudinal strain analysis.
- •
Minimum requirement of 50 studies recommended to achieve competency.
- •
Background in echocardiography influences interobserver reproducibility and time efficiency.
Attention ASE Members:
The ASE has gone green! Visit www.aseuniversity.org to earn free continuing medical education credit through an online activity related to this article. Certificates are available for immediate access upon successful completion of the activity. Nonmembers will need to join the ASE to access this great member benefit!
Methods
Study Design
This is a longitudinal study in which myocardial strain was retrospectively analyzed in 100 patients. All patients had good image quality with fewer than two uninterpretable segments by visual analysis. There were four groups of observers who undertook offline postprocessing strain analysis on the same 100 patients in the exact same order of sequence over a period of 3 months from April to June 2016. The four groups of observers were (1) experts, (2) cardiology fellows, (3) cardiac sonographers, and (4) medical students. Each group had two observers for interobserver validation and standardization within each group. By definition, the expert group I (J.C. and K.S.) was considered as the reference standard for development of the learning curve. The experts have experience equivalent to Level III ASE competency in echocardiography with greater than 3 years of extensive clinical and research experience in strain analysis and previous publications in the field of strain imaging. The remaining groups of novice observers have different backgrounds and levels of previous exposure to echocardiography, but none have had any previous experience in strain analysis. Group 2 comprised two cardiology fellows (N.G.O. and J.H.) who are currently undergoing a cardiology training program and have achieved the equivalence of at least Level II ASE competency in echocardiography. Group 3 comprised two accredited cardiac sonographers (R.C. and A.S.) who are competent in echocardiography acquisition with more than 2 years of experience from their postgraduate professional qualifications and certification in echocardiography. Group 4 comprised two medical students (I.S. and W.S.) undergoing their first M.D. postgraduate medical degree studies. The observers were independent, and the results were blinded to each observer. The study was approved by the ethics committee of the local institution.
Training Protocol
At baseline, apart from the experts, all observers had no previous experience in strain analysis. In the beginning, all three groups of novice observers were subject to an identical standardized training module prior to commencement of strain analysis. The permission for publication of results had been obtained from each observer. The training module was presented by the expert (J.C.) and consisted of a standard tutorial in addition to supervised practical hands-on training. The standard tutorial covered topics including (1) principles and fundamentals of strain imaging, (2) standard echocardiographic views with reference to basic cardiac anatomy, (3) strain analysis workflow with specific buttonology for offline analysis using vendor-specific software EchoPAC BT13 (GE, Horten, Norway), and (4) tips and pitfalls in strain analysis similar to protocol published by Negishi et al. After the tutorial, each observer was given the opportunity to perform hands-on offline strain analysis on three consecutive patients under the supervision and guidance of the expert. After formal standardized training, the observers undertook independent blinded strain analysis on a cohort of 100 patients in the same order of sequence over a defined period of 3 months.
Study Population
We retrospectively performed offline strain analysis on 100 patients. The study population consisted of patients who underwent two-dimensional (2D) echocardiography with clinical indications that included dobutamine stress echocardiography, assessment of chest complaint, known ischemic heart disease, valvular heart disease, other cardiomyopathies, and pericarditis. Only patients with good image quality were included with fewer than two segments uninterpretable by visual assessment. Other exclusion criteria not suitable for strain analysis were arrhythmias and pacemaker implants.
Echocardiography
Standard 2D echocardiography was performed with the patient in the left lateral decubitus position using a commercially available system (Vivid E9, General Electric Vingmed, Milwaukee, WI) equipped with a 3.5-MHz transducer. Standard 2D images triggered to the QRS complex and were saved in cineloop digital format. LV end-systolic and end-diastolic volumes were assessed, and LVEF was calculated from the apical four- and two-chamber views using Simpson’s biplane method. For the assessment of GLS, apical four-chamber, two-chamber, and long-axis views were acquired with a frame rate between 50 and 80 frames/sec. For the assessment of short-axis strain (GCS and GRS), images were acquired at parasternal short-axis views at a frame rate between 50 and 80 frames/sec of the LV at three levels: basal (level of mitral valve), mid (level of papillary muscle), and apical. Short-axis views were acquired as circular as possible and perpendicular to the long axis of the left ventricle. The set of echocardiographic images acquired were digitally stored to enable offline strain analysis by the different groups of blinded observers.
GLS
Global LV longitudinal strain was quantified using the automated function imaging (AFI) protocol from vendor-specific offline analysis software EchoPAC BT13 (GE Healthcare, Horten, Norway). The software analyzed and enabled frame-to-frame tracking of 2D motion of speckles within the myocardium, which represented natural acoustic markers. GLS analysis required the images of three apical views (apical long-axis, four- and two-chamber). First, the end-systolic frame is defined in the apical long-axis view by closure of the aortic valve (AVC) using the automatic function to ensure consistency of AVC between observers. This AVC interval is used as a subsequent reference for the four- and two-chamber view loops. After defining the mitral annulus and LV apex with 3 index points at the end-systolic frame in each apical view, the automated algorithm traces and tracks the motion of the endocardium. If the tracking does not seem to be accurate, the region of interest (ROI) can be readjusted by correcting the endocardial border or width of ROI or by selecting a new ROI altogether. The observer then visually checked and validated the automated endocardial border tracking of each myocardial segment and repeated the process of tracking if required. The automated algorithm, using a 17-segment model, calculated the GLS, which was the weighted average of the peak systolic longitudinal strain of all segments. The processing time required from the beginning to end of the GLS analysis was recorded. The average heart rate of image acquisition was 68 ± 12 bpm. The average frame rates of image acquisition were 53 ± 5.7 Hz. Out of a total of 1,700 segments for GLS, the software was able to track 98% of segments.
Global Short-Axis Strain
The GCS and GRS were derived from images of the parasternal short-axis views at three levels (basal, mid, apical) using commercially available 2D strain offline analysis software (EchoPAC BT13, GE Healthcare). The endocardial border of each short-axis level was manually traced in the end-systolic frame defined by AVC. An ROI was then drawn to include the entire myocardium. The semiautomated 2D strain algorithm then automatically segmented the LV short axis into six equidistant segments and selected suitable speckles for tracking frame by frame in the 2D short-axis plane. The observer then visually checked and validated the tracking quality and manually readjusted the ROI if required. Regional strain curves were then analyzed at end systole to minimize observer variability. The GCS and GRS were manually calculated by taking the average of the regional peak systolic circumferential and radial strain values of all segments in all three levels, respectively. The total processing time required for short-axis analysis to obtain GCS and GRS was recorded. Among a total of 1,800 segments for three levels of short axis (apex, mid, and basal), the software was able to track with a feasibility of 93% of all segments.
Statistical Analysis
Continuous data were presented as mean values ± SD. Learning curves were derived by analyzing the data in four quartiles of 25 patients. The GLS, GCS, and GRS measurements from each group of observers were compared to those of the experts using linear regression analysis with intraclass correlation coefficients (ICCs) and Bland-Altman analysis to derive the mean bias ± 1.96 SD. Learning curves for each type of strain were plotted in a graph with ICC value on the y axis and number of patients in quartiles on the x axis. An ICC plateau of >0.90 compared to expert was considered equivalent to expert-level achievement of accuracy and competency. Interobserver measurement variability within each group of observers was determined by using Spearman’s correlation, Bland-Altman analysis, and percentage difference (PD), which is defined as the absolute difference between two paired values divided by the average of the two values. It is expressed as a percentage. Interobserver variability among experts was determined in a randomly selected population of 25 patients. This forms the basis of reference standard for comparing 95% CI limits of agreements (LOA) between observers in the learning curve. When comparing continuous data such as timing between two groups, Student’s t test was used. A P value of < .05 was considered significant.
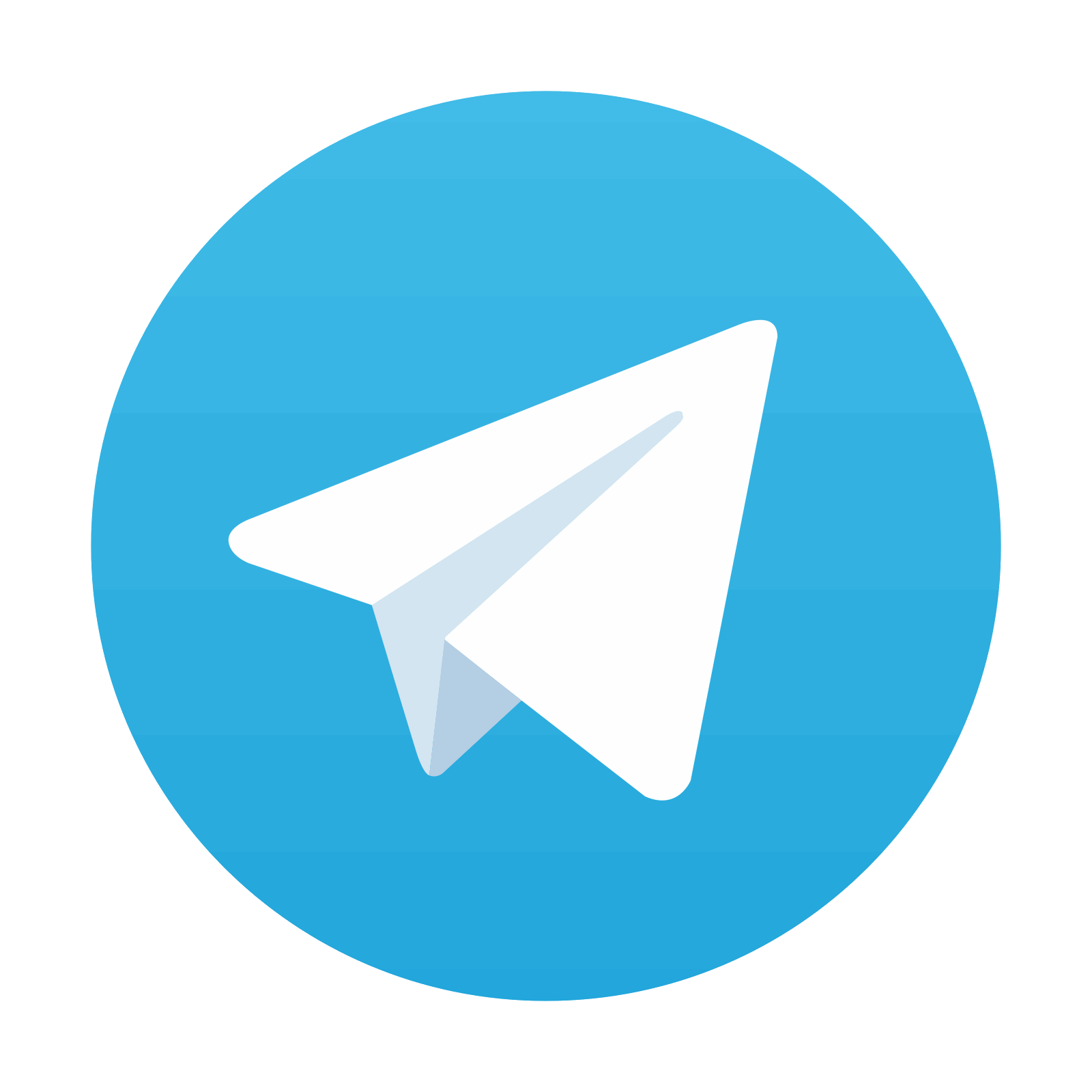
Stay updated, free articles. Join our Telegram channel

Full access? Get Clinical Tree
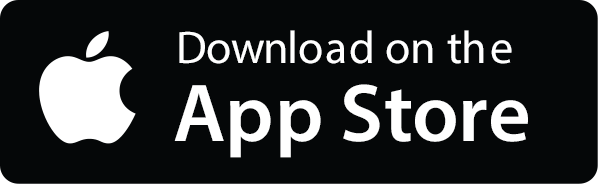
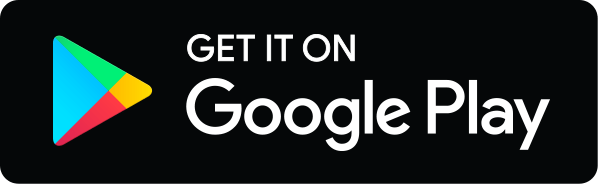