Background
Hypoxia depresses myocardial contractility in vitro but does not affect or may even improve indices of myocardial performance in vivo, possibly through associated changes in autonomic nervous system tone. The aim of this study was to explore the effects of hypoxic breathing on speckle-tracking echocardiographic indices of left ventricular function, with and without β 1 -adrenergic inhibition.
Methods
Speckle-tracking echocardiography was performed in 21 healthy volunteers in normoxia and after 30 min of hypoxic breathing (fraction of inspired oxygen, 0.12). Measurements were also obtained after the administration of atropine in normoxia ( n = 21) and after bisoprolol intake in normoxia ( n = 6) and in hypoxia ( n = 10).
Results
Hypoxia increased heart rate (from 68 ± 11 to 74 ± 9 beats/min, P = .001), without changing mean blood pressure ( P = NS), and decreased total peripheral resistance ( P = .003). Myocardial deformation magnitude increased (circumferential strain, −19.6 ± 1.9% vs −21.2 ± 2.5%; radial strain, 19.2 ± 3.7% vs 22.6 ± 4.1%, P < .05; longitudinal and circumferential strain rate, −0.88 ± 0.11 vs −0.99 ± 0.15 sec −1 and −1.03 ± 0.16 vs −1.18 ± 0.18 sec −1 , respectively, P < .05 for both; peak twist, 8.98 ± 3.2° vs 11.1 ± 2.9°, P < .05). Except for peak twist, these deformation parameters were correlated with total peripheral resistance ( P < .05). Atropine increased only longitudinal strain rate magnitude (−0.88 ± 0.11 vs −0.97 ± 0.14 sec −1 , P < .05). The increased magnitude of myocardial deformation persisted in hypoxia under bisoprolol ( P < .05). In normoxia, bisoprolol decreased heart rate (73 ± 10 vs 54 ± 7 beats/min, P = .0005), mean blood pressure (88 ± 7 vs 81 ± 4 mm Hg, P = .0027), without altering deformation.
Conclusions
Hypoxic breathing increases left ventricular deformation magnitude in normal subjects, and this effect may not be attributed to hypoxia-induced tachycardia or β 1 -adrenergic pathway changes but to hypoxia-induced systemic vasodilation.
Acute hypoxia may result from different situations, such as asphyxia and airway obstruction, and is associated with a rapid depletion in available oxygen at all tissue levels as well as in the myocardium. Hypoxia has been reported to decrease myocardial fiber contractility under in vitro experimental conditions and in intact animal preparations, but it does not affect or even improves indices of myocardial performance, such as left ventricular (LV) ejection fraction (LVEF) and cardiac output, in vivo in human studies. The discrepancies between these in vivo and in vitro studies could be explained by hypoxia-associated sympathetic activation. In addition to direct inotropic effects of catecholamines, hypoxia-associated tachycardia could in itself be a cause of increased contractility. In addition, previous human studies have used echocardiography-derived LVEF measurements, which inherently have poor reproducibility and sensitivity to detect subtle changes in LV function. Speckle-tracking echocardiography (STE) may overcome these limitations.
We therefore explored in healthy volunteers the effects of acute hypoxic exposure on conventional echocardiographic and STE-derived measurements of LV function. To characterize the role of the sympathetic nervous system in the myocardial effects of hypoxia, we evaluated the effects of β-blockade on myocardial function in hypoxia.
We assessed the effects of atropine in normoxia to explore the possibility of a chronotropic-related myocardial effect.
Methods
Study Population
Twenty-one participants (mean age, 27 ± 7 years) were studied in normoxia, in hypoxia, and in normoxia after atropine administration. In a second stage, 10 subjects (five of whom were new participants; mean age, 26 ± 7 years) were studied in normoxia and in hypoxia under β-blockade, and six of these subjects were also studied in normoxia under β-blockade.
Inclusion criteria were as follows: age 20 to 40 years; normal results on physical examination, 12-lead electrocardiography, and standard echocardiography; and resting heart rate (HR) > 55 beats/min (>60 beats/min in the β-blocker substudy).
Exclusion criteria were contraindication to the use of atropine or bisoprolol, smoking, and physical training of >2 hours per week.
The study protocol was approved by our local ethics committee (P2009/304, P2010/270). All participants provided written informed consent.
Study Design
Normoxia, Hypoxia and Normoxia with Atropine ( n = 21)
Normoxia and Normobaric Hypoxia
The study was carried out as diagrammed in Figure 1 . Comprehensive echocardiography and STE were performed in normoxia and after 30 min of hypoxic breathing. The hypoxic test was performed using a tightly adapted mask permitting inspiration of a mixture of 12% oxygen in nitrogen (Messer Belgium NV, Machelen, Belgium). This degree of hypoxia, corresponding to an altitude of 4,500 m, has been shown to be well tolerated, with minimal changes in P co 2 . HR, blood pressure (BP), arterial oxygen saturation (SaO 2 ) (Datex, Aartselaar, Belgium) and expired P co 2 (PNT Digital; Medical Electronic Construction, Brussels, Belgium) were continuously monitored.

Echocardiography was performed in a stable state, after a plateau SaO 2 had been reached for ≥20 min. The maximal duration of the hypoxic test, including echocardiography, was 90 min.
Atropine Infusion in Normoxia
Imaging data were acquired in normoxia after an intravenous bolus (0.5 mg, with a maximum of 1 mg) of atropine (Sterop Laboratories, Brussels, Belgium), ≥1 hour after the hypoxic test, allowing the return to baseline of HR, SaO 2 , and BP.
Hypoxia under β-blockade ( n = 10) and Normoxia under β-blockade ( n = 6)
Echocardiographic measurements were obtained in normoxia without β-blockade and in hypoxia after administration of bisoprolol (a β 1 -blocking agent with a minimal effect expected on total peripheral resistance (TPR) in 10 subjects. Bisoprolol (Mylan SPRL, Overijse, Belgium) was administered at a dose of 5 mg/day for 48 hours. In a preliminary study, a higher dose of bisoprolol could not be used, because of the occurrence of lipothymia during hypoxia. In a second set of experiments in six subjects, the same echocardiographic measurements were obtained under β-blockade in normoxia.
Echocardiography
Images were acquired using an iE33 echocardiographic system (Philips Medical Systems, Andover, MA). LVEF and LV volumes were evaluated using the apical biplane method of disks. LV stroke volume was calculated as LV stroke volume = Π × (LV outflow tract diameter/2) 2 × LV outflow tract velocity-time integral and was indexed to body surface area. TPR was estimated as TPR (Wood units) = mean BP/cardiac output.
Mitral annular systolic velocity (mitral S wave) was obtained by spectral tissue Doppler and averaged from septal and lateral values. The LV myocardial performance (Tei) index was obtained from a single cardiac cycle using the following formula: [(interval between cessation and onset of the mitral inflow velocity−ejection time)/ejection time]. The ratio of isovolumic relaxation time to RR time interval was calculated as described by Huez et al.
Right ventricular (RV) fractional area change, tricuspid annular plane systolic excursion, RV myocardial performance (Tei) index, myocardial isovolumic acceleration, and peak systolic velocity at the tricuspid valve (tricuspid S wave) were measured as previously described. The transtricuspid pressure gradient was obtained using the Bernoulli equation using the peak velocity of the regurgitant jet. Systolic pulmonary arterial pressure (PAP) was calculated as the sum of the transtricuspid pressure gradient and the estimated right atrial pressure, on the basis of the collapsibility of the inferior vena cava.
The mean PAP was calculated as 0.61 × systolic PAP + 2 mm Hg. Pulmonary vascular resistance (Wood units) was calculated as [(peak velocity of the tricuspid regurgitant jet/RV outflow tract velocity-time integral) × 10 + 0.16].
All Doppler measurements were averaged from three consecutive beats.
STE
Strain, strain rate, and LV twist parameters were analyzed offline using two-dimensional speckle-tracking echocardiographic software (QLAB Advanced Quantification Software version 7.1; Philips Medical Systems).
Quantification of myocardial deformation was performed as previously reported. Briefly, global LV peak systolic longitudinal strain (GSLS) and global LV peak systolic longitudinal strain rate (GSLSR) were calculated in an 18-segment model from the apical two-chamber, three-chamber, and four-chamber views, acquired with the same frame rate. Global LV peak systolic radial strain (GSRadS) and global LV peak systolic radial strain rate (GSRadSR) and global LV circumferential strain (GSCirS) and global LV circumferential strain rate (GSCirSR) were averaged from peak systolic strain in each segment from basal, mid (papillary muscle level), and apical short-axis views using the same frame rate. Frame rates between 60 and 90 sec −1 were used for speckle-tracking echocardiographic recordings. Inadequately tracked segments (17% of segments analyzed) were excluded from the analysis. Postsystolic shortening was defined as maximal systolic strain occurring after aortic valve closure. Because GSLS, GSLSR, GSCirS, and GSCirSR are expressed as negative values (shortening), we termed a larger negative systolic strain or strain rate measurement “increased strain (rate) magnitude” and, conversely, smaller negative numbers “decreased strain (rate) magnitude.”
LV twist and twist rate were assessed from basal and apical short-axis views using the same frame rate. LV twist was defined as the net difference between apical rotation and basal rotation at isochronal time points. Time intervals were normalized for the systolic time interval and the diastolic time interval to enable comparison between RR cycles of different durations. Because apical and basal rotation and rotation rate data cannot be acquired from the same cardiac cycle, and to allow comparison within and among subjects, cubic spline interpolation (MATLAB version R2007b; The MathWorks, Inc., Natick, MA) was performed. Twist-area loops were constructed as previously described. LV torsion was obtained by dividing the LV twist value by the LV end-diastolic length (in centimeters), as measured in a four-chamber view.
Measurement Reproducibility
Intraobserver and interobserver variability was assessed in 20 subjects selected randomly by two observers (C.D., P.U.), on the same cardiac cycle, blinded to previous measurements. To assess intraobserver variability for peak LV twist and GSLS, measurements were repeated 1 month after the first reading on the same recordings. Interobserver variability was assessed from measurements performed by a second operator on the same echocardiographic recordings. Reproducibility was assessed by calculating the absolute difference between repeated measurements expressed as a percentage of their mean and by intraclass correlation coefficients.
Statistical Analysis
Results are expressed as mean ± SD. Data were tested for normality using the Kolmogorov-Smirnov test. For multiple comparisons, one-way analysis of variance for repeated measurements with post hoc Bonferroni’s correction, or a Friedman test if the data were not distributed normally, was used. Student’s t tests for paired samples, or Mann-Whitney tests if the data were not distributed normally, were used for comparisons within the same group between different states.
Correlations were tested using linear regression analysis and Pearson’s correlation coefficient. Two-sided P values < .05 were considered significant.
Results
Effect of Normobaric Hypoxia
Clinical characteristics of the subjects are shown in Table 1 . The mean age of the subjects was of 27 ± 7 years (seven men, 14 women), and the mean body surface area was 1.73 ± 0.16 m 2 . Compared with normoxia, hypoxic breathing decreased SaO 2 (as expected), increased HR, and did not alter BP. There was a small decrease in P co 2 .
Variable | Normoxia ( n = 21) | Hypoxia ( n = 21) | Normoxia with atropine ( n = 21) |
---|---|---|---|
HR (beats/min) | 68 ± 11 | 74 ± 9 ∗ | 79 ± 10 ∗ † |
Systolic BP (mm Hg) | 109 ± 9 | 114 ± 11 | 113 ± 9 |
Diastolic BP (mm Hg) | 64 ± 10 | 63 ± 8 | 69 ± 9 |
Mean BP (mm Hg) | 79 ± 9 | 80 ± 8 | 85 ± 12 |
SaO 2 (%) | 97 ± 1 | 76 ± 8 ∗ | 98 ± 1 † |
Arterial P co 2 (mm Hg) | 42 ± 2 | 40 ± 3 ∗ | 41 ± 4 |
GSLS (%) | −17.7 ± 2.0 | −18.4 ± 2.2 | −17.2 ± 2.1 † |
GSLSR (sec −1 ) | −0.88 ± 0.11 | −0.99 ± 0.15 ∗ | −0.97 ± 0.14 ∗ |
GSCirS (%) | −19.6 ± 1.9 | −21.2 ± 2.5 ∗ | −19.4 ± 1.9 † |
GSCirSR (sec −1 ) | −1.03 ± 0.16 | −1.18 ± 0.18 ∗ | −1.09 ± 0.14 |
GSRadS (%) | 19.2 ± 3.7 | 22.6 ± 4.1 ∗ | 18.6 ± 4.6 † |
GSRadSR (sec −1 ) | 1.04 ± 0.30 | 1.23 ± 0.26 | 1.09 ± 0.29 |
Peak LV systolic twist (°) | 8.98 ± 3.2 | 11.1 ± 2.9 ∗ | 9.9 ± 2.6 |
Peak LV systolic torsion (°/cm) | 1.15 ± 0.38 | 1.42 ± 0.34 ∗ | 1.29 ± 0.33 |
Peak apical systolic rotation (°) | 6.9 ± 2.6 | 8.7 ± 2.6 ∗ | 7.3 ± 2.6 |
Peak basal systolic rotation (°) | −2.4 ± 1.6 | −2.8 ± 1.5 | −3.17 ± 1.23 ∗ |
Peak untwisting rate (°/sec) | −94.6 ± 35.5 | −111.4 ± 37.8 | −92.7 ± 26.2 † |
Conventional Echocardiography
LVEF and cardiac index increased compared with normoxia. Stroke volume and LV volumes remained similar, and TPR decreased. Mitral S wave slightly increased, and the LV Tei index was not modified. The mitral E/A ratio decreased and the isovolumic relaxation time/RR interval ratio increased. The transtricuspid pressure gradient, mean PAP, and pulmonary vascular resistance increased. Other standard echocardiographic parameters were not altered by hypoxia ( Table 2 ).
Variable | Normoxia ( n = 21) | Hypoxia ( n = 21) | Normoxia with atropine ( n = 21) |
---|---|---|---|
LVEF (%) | 65 ± 4 | 68 ± 7 ∗ | 63 ± 5 † |
LV mass index (g/m 2 ) | 88.9 ± 14.7 | ||
Cardiac index (L/min/m 2 ) | 2.8 ± 0.6 | 3.3 ± 0.7 ∗ | 3.2 ± 0.4 ∗ |
Stroke volume index (mL/m 2 ) | 42.1 ± 7.7 | 45.4 ± 8.2 | 38.8 ± 7.3 † |
TPR (Wood units) | 17.3 ± 4.1 | 14.7 ± 3.4 ∗ | 15.6 ± 2.6 |
LV end-diastolic volume index (mL/m 2 ) | 41.9 ± 8.3 | 40.5 ± 10.9 | 40.2 ± 9.9 |
LV end-systolic volume index (mL/m 2 ) | 15.1 ± 3.3 | 13.4 ± 4.5 | 15.7 ± 6.4 |
Mitral S wave (cm/sec) | 8.1 ± 1.1 | 8.7 ± 1.4 | 8.9 ± 1.8 |
Peak E wave (cm/sec) | 87 ± 17 | 87 ± 14 | 74 ± 10 ∗ † |
Peak A wave (cm/sec) | 42 ± 9 | 48 ± 6 | 52 ± 9 ∗ |
Mitral E/A ratio | 2.1 ± 0.5 | 1.8 ± 0.4 | 1.4 ± 0.2 ∗ † |
IVRT/RR interval (%) | 6.8 ± 1.5 | 7.7 ± 1.2 ∗ | |
Ea (mean lateral-septal) (cm/sec) | 14.3 ± 2.4 | 14.0 ± 1.6 | 12.1 ± 2.3 ∗ † |
E/Ea ratio | 6.2 ± 1.2 | 6.3 ± 0.9 | 5.9 ± 1.8 |
LV Tei index | 0.43 ± 0.20 | 0.39 ± 0.07 | 0.47 ± 0.11 |
Tricuspid S wave (cm/sec) | 13.2 ± 2.4 | 13.3 ± 2.5 | 12.3 ± 2.1 |
TAPSE (mm) | 2.4 ± 0.4 | 2.5 ± 0.4 | 2.2 ± 0.3 ∗ † |
TTPG (mm Hg) | 13.7 ± 3.9 | 19.5 ± 6.3 ∗ | |
PVR (Wood units) | 1.4 ± 0.4 | 1.7 ± 0.5 ∗ | |
Mean PAP (mm Hg) | 13.4 ± 2.4 | 17.0 ± 3.9 ∗ | |
RV Tei index | 0.27 ± 0.19 | 0.26 ± 0.19 | 0.19 ± 0.11 |
RV FAC (%) | 50 ± 8 | 47 ± 7 | 47 ± 13 |
IVA (m/s 2 ) | 2.5 ± 1.2 | 2.1 ± 0.8 |
STE
The magnitudes of GSLSR, GSCirS and GSCirSR, and GSRadS were increased in hypoxia compared to normoxia ( Figures 2 A, 2 B, and 3 ), as were peak LV twist ( Figure 4 ), torsion, and apical rotation. Postsystolic shortening was observed for longitudinal strain in four subjects in normoxia and in two subjects after hypoxic breathing ( Table 1 ).
Intraobserver variability was 2.4% for LV twist and <1% for GSLS, and interobserver variability was 3.8% for LV twist and 2.9% for GSLS. Intraclass correlation coefficients between the same observer and two different observers for LV twist were 0.97 and 0.94, respectively ( P < .001). Intraclass correlation coefficients between the same observer and two different observers for GSLS were 0.96 and 0.91, respectively ( P < .001).
Effects of Atropine Infusion in Normoxia
The effects of atropine on HR and BP are shown in Table 1 . The mean LVEF in normoxia under atropine (63 ± 5%) was similar to that in normoxia alone ( P = .555) and less than that in hypoxia ( P = .001). The stroke volume index (38.8 mL/m 2 ) was not changed compared with normoxia alone ( P = .128) and was less than in hypoxia ( P < .001).
STE
In normoxia with atropine, GSLS, GSCirS, and GSRadS were similar to those seen in normoxia alone. GSLSR and basal rotation magnitude were increased compared with normoxia ( Table 1 , Figure 2 A and 2 B).
Effects of β-blockade
Hypoxia under β-blockade
Clinical Characteristics
The mean age of the subjects was 26 ± 7 years (six men, four women), and the mean body surface area was 1.84 ± 0.17 m 2 . Before starting the hypoxic test, β-blockade was associated with decreases in HR (63 ± 8 vs 76 ± 12 beats/min, P = .001) and BP (systolic, 107 ± 8 vs 117 ± 13 mm Hg, P = .0187; diastolic, 63 ± 8 vs 73 ± 6 mm Hg, P = .0105; mean, 77 ± 5 vs 88 ± 7 mm Hg, P = .032, in normoxia with β-blockade vs normoxia alone, respectively). Thereafter, HR increased with hypoxic breathing from 63 ± 8 to 76 ± 9 beats/min ( P < .0001), whereas BP was unchanged (systolic, 107 ± 8 vs 104 ± 8 mm Hg, P = .1188; diastolic, 63 ± 8 vs 59 ± 9 mm Hg, P = .3128; mean, 77 ± 5 vs 74 ± 8 mm Hg, P = .2128).
Conventional Echocardiography
LVEF, cardiac index, stroke volume, LV volumes, mitral S wave, and LV Tei index were not altered in hypoxic breathing under β-blockade compared with normoxia. TPR decreased. The peak E wave was increased, and the E/A ratio remained unchanged. The transtricuspid pressure gradient and mean PAP tended to increase. Tricuspid annular plane systolic excursion, tricuspid S wave, RV Tei index, RV fractional area change, and peak isovolumic acceleration were unchanged.
STE
GSLS, GSRadS and GSRadSR, and apical rotation magnitude increased ( Table 3 , Figure 3 ).
Variable | Normoxia ( n = 10) | Hypoxia with β-blockade ( n = 10) | P | Normoxia ( n = 6) | Normoxia with β-blockade ( n = 6) | P |
---|---|---|---|---|---|---|
HR (beats/min) | 76 ± 12 | 76 ± 9 | .133 | 73 ± 10 | 54 ± 7 | <.001 |
Systolic BP (mm Hg) | 117 ± 13 | 104 ± 8 | <.01 | 117 ± 8 | 111 ± 8 | <.05 |
Diastolic BP (mm Hg) | 73 ± 6 | 59 ± 9 | <.001 | 74 ± 7 | 66 ± 4 | <.01 |
Mean BP (mm Hg) | 88 ± 7 | 74 ± 8 | <.001 | 88 ± 7 | 81 ± 4 | <.01 |
SaO 2 (%) | 97 ± 2 | 83 ± 4 | .000 | |||
Arterial P co 2 (mm Hg) | 43 ± 6 | 41 ± 6 | NS | |||
LVEF (%) | 68 ± 5 | 70 ± 6 | .325 | 66 ± 4 | 66 ± 7 | .903 |
LV mass index (g/m 2 ) | 87.1 ± 13.6 | 87.1 ± 13.6 | 1 | |||
Cardiac index (L/min/m 2 ) | 3.1 ± 0.8 | 3.3 ± 0.6 | .386 | 2.7 ± 0.6 | 2.1 ± 0.4 | <.05 |
Stroke volume index (mL/m 2 ) | 44.7 ± 7.5 | 45.0 ± 7.6 | .869 | 42.1 ± 5.5 | 42.6 ± 7.4 | .693 |
TPR (Wood) | 16.4 ± 4.0 | 12.5 ± 1.9 | <.05 | 18.6 ± 3.4 | 21.8 ± 5.6 | <.05 |
LV end-diastolic volume index (mL/m 2 ) | 45.9 ± 6.7 | 44.2 ± 8.3 | .3636 | |||
LV end-systolic volume index (mL/m 2 ) | 15.9 ± 4.2 | 15.2 ± 2.9 | .684 | |||
Mitral S wave (cm/sec) | 8.7 ± 1.0 | 9.5 ± 1.0 | .101 | |||
Peak E wave (cm/sec) | 83 ± 10 | 93 ± 7 | .001 | |||
Peak A wave (cm/sec) | 43 ± 12 | 44 ± 17 | .910 | |||
Mitral E/A ratio | 2.0 ± 0.5 | 1.9 ± 0.4 | .617 | |||
IVRT/RR interval (%) | 7.9 ± 1.3 | 7.5 ± 1.0 | .2905 | |||
Ea (mean lateral-septal) (cm/sec) | 15.2 ± 2.0 | 14.6 ± 1.9 | .384 | |||
E/Ea ratio | 5.4 ± 1.2 | 6.5 ± 1.2 | <.05 | |||
LV Tei index | 0.34 ± 0.04 | 0.35 ± 0.10 | .586 | |||
Tricuspid S wave (cm/sec) | 13.2 ± 1.5 | 11.6 ± 4.5 | .315 | |||
TAPSE (mm) | 2.6 ± 0.4 | 2.8 ± 0.3 | .061 | |||
TTPG (mm Hg) | 18.3 ± 3.5 | 21.4 ± 4.8 | .063 | |||
PVR (Wood units) | 1.9 ± 0.3 | 1.9 ± 0.6 | .710 | |||
Mean PAP (mm Hg) | 16.2 ± 2.1 | 18.1 ± 2.9 | .059 | |||
RV Tei index | 0.19 ± 0.13 | 0.21 ± 0.12 | .476 | |||
RV FAC (%) | 53 ± 12 | 45 ± 8 | .176 | |||
IVA (m/s 2 ) | 2.7 ± 1.2 | 2.1 ± 0.4 | .379 | |||
GSLS (%) | −16.9 ± 2.2 | −19.3 ± 1.7 | <.05 | −19.1 ± 0.6 | −18.2 ± 2.3 | .419 |
GSLSR (sec −1 ) | −0.92 ± 0.11 | −1.01 ± 0.07 | .079 | −1.01 ± 0.07 | −0.87 ± 0.12 | .052 |
GSCirS (%) | −21.0 ± 3.6 | −23.9 ± 4.6 | .072 | −21.3 ± 2.8 | −19.5 ± 5.7 | .420 |
GSCirSR (sec −1 ) | −1.15 ± 0.14 | −1.22 ± 0.18 | .383 | −1.15 ± 0.10 | −1.03 ± 0.12 | .111 |
GSRadS (%) | 20.2 ± 4.3 | 27.0 ± 7.3 | <.01 | 20.5 ± 4.1 | 21.5 ± 10.6 | .760 |
GSRadSR (sec −1 ) | 1.11 ± 0.17 | 1.45 ± 0.31 | <.05 | 1.21 ± 0.23 | 1.21 ± 0.35 | .9974 |
Peak LV systolic twist (°) | 9.8 ± 2.9 | 11.9 ± 3.5 | .112 | 11.7 ± 3.5 | 10.0 ± 2.3 | .212 |
Peak LV systolic torsion (°/cm) | 1.18 ± 0.40 | 1.42 ± 0.45 | .115 | 1.37 ± 0.45 | 1.18 ± 0.32 | .223 |
Peak apical systolic rotation (°) | 7.5 ± 2.2 | 9.9 ± 2.9 | <.05 | 8.3 ± 2.2 | 8.5 ± 2.3 | .687 |
Peak basal systolic rotation (°) | −2.7 ± 1.4 | −2.6 ± 1.1 | .767 | −4.1 ± 1.2 | −2.2 ± 0.7 | <.05 |
Peak untwisting Rate (°/sec) | −103.0 ± 41.7 | −133.6 ± 45.2 | .128 | −117.3 ± 22.6 | −95.8 ± 18.4 | .078 |
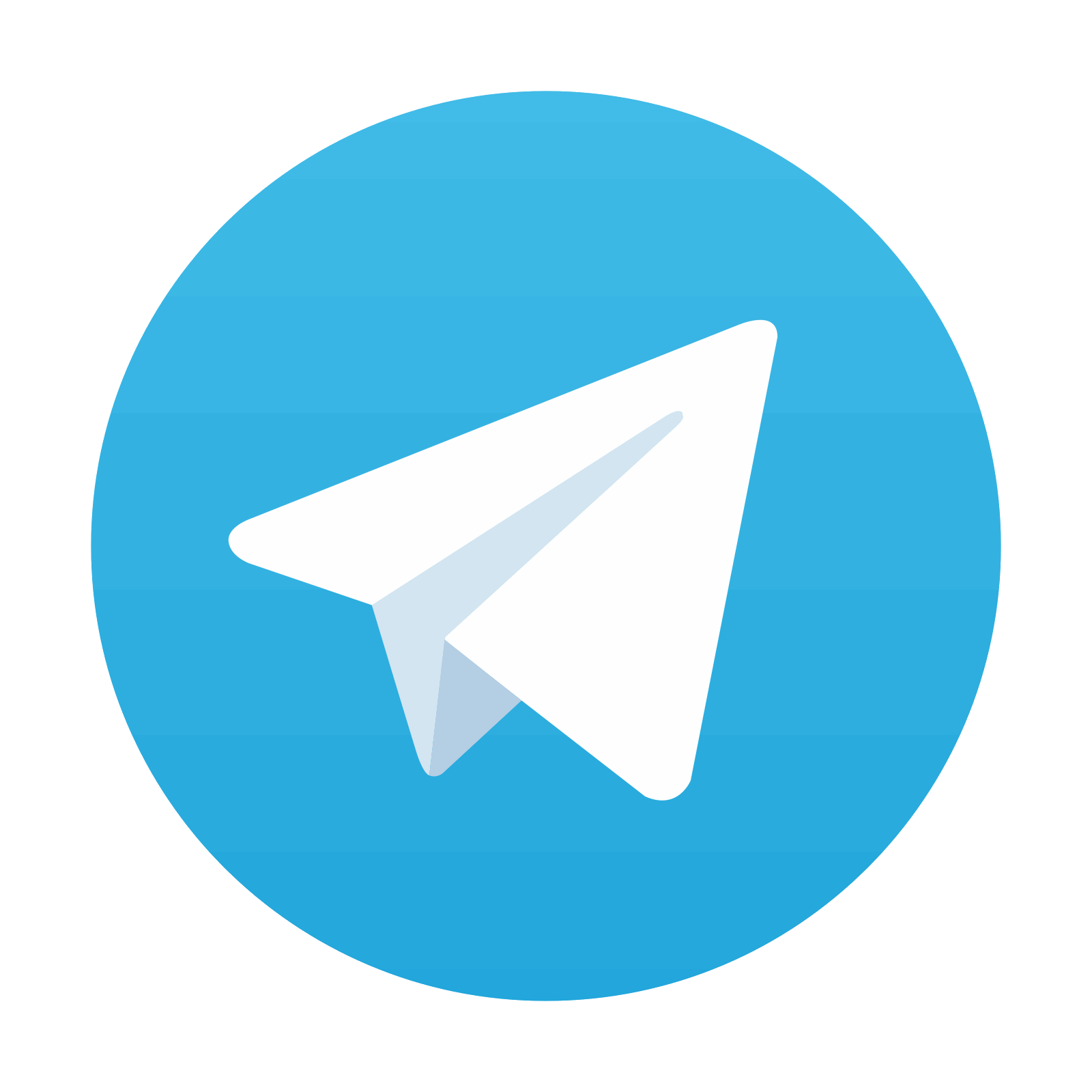
Stay updated, free articles. Join our Telegram channel

Full access? Get Clinical Tree
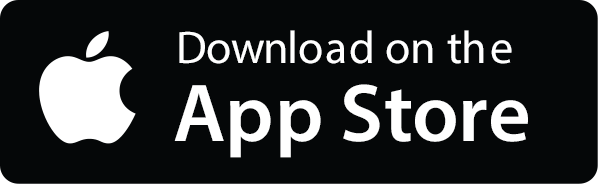
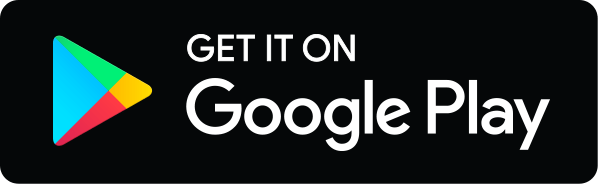
