Background
Long-term outcome in repaired tetralogy of Fallot (TOF) is related to chronic pulmonary insufficiency (PI), right ventricular (RV) dilation, and deterioration of RV function. The aim of this study was to characterize clinical differences between restrictive and nonrestrictive RV physiology in young patients with repaired TOF.
Methods
Patients were prospectively enrolled from February 2008 to August 2009. Each had a clinic visit, brain natriuretic peptide assessment, exercise test, cardiac magnetic resonance study, and echocardiographic examination with assessment of regional myocardial mechanics. Consistent antegrade diastolic pulmonary arterial flow with atrial contraction identified restrictive RV physiology.
Results
Twenty-nine patients (median age, 12 years; range, 8–33 years; nine male patients) were studied. Twelve had restrictive RV physiology. The median time since initial TOF repair was 12 years (range, 5–27 years). Restrictive physiology appeared more prevalent after transannular patch repair and was not influenced by other demographic features. The restrictive group had more PI (46% vs 28%, P = .002), larger RV end-diastolic volumes (128 vs 98 mL/m 2 , P = .046), but similar ejection fractions, brain natriuretic peptide levels, New York Heart Association classes, and exercise capacity. RV basal and mid free wall peak diastolic strain rate differed between groups, negatively correlating with exercise time and positively correlating with PI in patients with restrictive physiology.
Conclusions
Restrictive RV physiology correlates with a larger right ventricle and increased PI after TOF repair but does not negatively affect other markers of myocardial health. Diastolic regional RV myocardial mechanics, particularly diastolic velocity and peak diastolic strain rate, differ for postoperative TOF patients with restrictive and nonrestrictive RV physiology; longitudinal study is necessary to understand the relationship of regional myocardial mechanics and patients’ clinical status.
Long-term outcome after repair of tetralogy of Fallot (TOF) is related to chronic pulmonary insufficiency (PI), right ventricular (RV) dilation, and deteriorating ventricular function. Pulmonary valve incompetence increases in severity over time and has been linked to deterioration of exercise capacity and RV function. RV diastolic dysfunction, RV fibrosis, and eventual left ventricular (LV) dysfunction also influence outcomes in these patients.
Traditionally, restrictive diastolic RV physiology has been characterized by the echocardiographic presence of end-diastolic antegrade flow in the main pulmonary artery (MPA) after atrial contraction. Restrictive diastolic RV physiology has been identified in survivors of TOF repair. Its presence has been considered potentially beneficial in patients late after repair, although the literature is inconsistent. In adults 15 to 35 years after repair, Gatzoulis et al. showed that those with restrictive right ventricles had smaller cardiothoracic ratios on chest x-ray and better exercise performance than those without restrictive RV physiology. Eroglu et al. concluded that RV restriction, at midterm follow-up after transannular patch repair of TOF, was more often associated with a smaller right ventricle and less prolongation of the QRS complex on electrocardiography. Yet Lu et al. recently showed that patients with repaired TOF with larger RV end-diastolic volumes had more evidence of restrictive physiology, a feature also noted recently in a Toronto cohort. Finally, Helbing et al. showed no significant difference in RV size, PI, or RV ejection fraction (EF) for young (mean age, 12 ± 3 years) patients with repaired TOF with restrictive and nonrestrictive RV physiology, and they also found that exercise function was diminished in patients with TOF with restrictive physiology.
Using cardiac magnetic resonance (CMR) imaging, the gold standard for RV assessment, the ranges of biventricular sizes and systolic function in patients with repaired TOF have been defined. In the present era, clinical decision making for patients with repaired TOF, especially regarding the appropriate timing of pulmonary valve replacement, remains challenging. Caregivers seek to avoid the irreversible ventricular dysfunction from chronic PI and volume overload, described in retrospective studies by Therrien et al. , in which RV volume thresholds (end-diastolic volume indexed to body surface area, 170 mL/m 2 ; end-systolic volume indexed to body surface area, 85 mL/m 2 ) predicted poor RV remodeling and recovery of RV function after pulmonary valve replacement.
Before these volumetric thresholds are attained, regional myocardial deformation may be altered and can be prospectively evaluated from echocardiography-derived speckle-tracking analysis to measure myocardial strain and provide direct information about the contractile performance of the right ventricle. Regional RV myocardial mechanics have been previously described in healthy children and in patients with congenital heart disease, with recent studies exploring regional myocardial mechanics in patients after repair of TOF. We sought to prospectively characterize and compare the clinical status and regional myocardial mechanics of young patients with restrictive versus nonrestrictive RV physiology after TOF repair at our institution, using the complementary modalities of CMR imaging and echocardiography.
Methods
Study Population
This was a prospective study with approval from the Children’s Hospital of Wisconsin Institutional Review Board. Informed consent and child assent were obtained from consecutively recruited patients with repaired TOF receiving care in the Herma Heart Center at the Children’s Hospital of Wisconsin from February 2008 to August 2009. Patients with a documented primary diagnosis of TOF were identified by review of clinic schedules and approached for enrollment in the study at the time of their clinic visits or while waiting for clinical testing. Patients were considered eligible if they were 8 to 40 years of age, had repaired TOF, and had at least mild residual PI on echocardiography. Furthermore, they were deemed eligible for study participation only if their cardiologists planned collection of all clinical data within 3 months of their clinic visits; these clinically indicated studies included assessment of New York Heart Association (NYHA) classification from clinic visit, brain natriuretic peptide (BNP) level, transthoracic echocardiography, CMR imaging, and Bruce-protocol exercise treadmill testing. Patients were excluded if they had (1) TOF with atrioventricular septal defect, (2) any major developmental disability that might limit their ability to complete required testing (e.g., DiGeorge syndrome), or (3) relative contraindications to CMR imaging (e.g., pregnancy, pacemaker, defibrillator). Of 76 patients with TOF (age range, 8–40 years) seen during this time period at Children’s Hospital of Wisconsin, 35 were eligible, and 29 successfully completed the protocol (with the remaining six having incomplete data collection within the 3-month time frame).
Clinical Evaluation
Pertinent medical history (e.g., original diagnoses, past palliations, cardiac catheter interventions, operative repairs, current medications, symptoms) as well as physical examination findings from the recent clinic visit were extracted from the medical record. Each individual’s NYHA classification was designated, by two independent cardiologists, on review of reported symptoms described in the recent clinic visit note. BNP level was obtained at the time of CMR imaging as an additional means of evaluating clinical status.
Exercise Stress Testing
All study subjects underwent Bruce-protocol exercise testing on a treadmill ergometer with incremental increases in speed and grade to voluntary exhaustion. Blood pressure and heart rate were obtained every minute. A 12-lead electrocardiogram was obtained every 3 min or at times of arrhythmia occurrence. Oxygen consumption was determined (SensorMedics, Inc., Yorba Linda, CA) and computer recorded on a breath-by-breath basis. Maximal oxygen consumption (V o 2 max) was expressed as absolute (liters per minute), relative (milliliters per kilogram per minute), and percentage of predicted. Maximal effort was considered if the peak heart rate exceeded 90% predicted, if a plateau occurred in oxygen consumption in the absence of a rise with increasing work, or if the respiratory quotient was >1.10. Postexercise monitoring included 12-lead electrocardiography and blood pressure monitoring through 5 min of recovery.
CMR Imaging
CMR imaging was performed per institutional protocol using a Siemens 1.5-T Symphony magnetic resonance scanner (Siemens Medical Solutions USA, Inc., Malvern, PA). Ventricular dimensions and function were assessed from electrocardiographically gated, breath-held steady-state free precession (TrueFISP; Siemens Medical Solutions USA, Inc.) cine CMR pulse sequences for the ventricular short-axis plane (matrix size, 192 × 192 or better; repetition time, 45–75 msec; echo train length, 1–2 msec; slice thickness, 6–8 mm). Steady-state free precession (TrueFISP) or gradient-echo (FLASH; Siemens Medical Solutions USA, Inc.) cine imaging was obtained for the RV outflow tract (sagittal and coronal planes) and the branch pulmonary arteries (axial plane). Through-plane flow assessment of MPA was obtained, using velocity-encoded retrospectively electrocardiographically gated, free-breathing phase-contrast CMR imaging (repetition time, 46 msec; echo train length, 3.9 msec; slice thickness, 5 mm) by prescribing a plane perpendicular to MPA flow. CMR data analyses were performed offline using a commercially available software package (Mass & Flow Analysis Package; MEDIS Medical Imaging Systems, Leiden, The Netherlands). RV and LV volumes, masses, and EFs were measured as described by Alfakih et al. Data were then normalized to each study subject’s body surface area to allow comparisons among patients.
Echocardiography
Echocardiography was performed using a Sequoia ultrasound system (Siemens Medical Solutions USA, Inc.). Standard electrocardiographically triggered digital capture occurred, and two-dimensional, color flow, and Doppler flow assessments were done per institutional protocol. The peak RV outflow tract gradient was measured offline from a continuous-wave Doppler recording using syngo Ultrasound Workplace (Siemens Medical Solutions USA Inc.). RV and LV diastolic function was measured offline using Doppler (peak E and peak A velocities and the E/A ratio) and Doppler tissue imaging of the tricuspid and mitral lateral annuli, according to methods described previously. Figure 1 shows key Doppler tissue imaging measurements. Finally, restrictive RV physiology was confirmed with spectral Doppler tracings showing consistent (at least three heartbeats) antegrade end-diastolic flow into the MPA with atrial contraction by standard methods described by Redington et al. ( Figure 2 ). Because these were clinical echocardiographic examinations, respiratory monitoring was not standard procedure, and patients were simply breathing comfortably. Thus, consistency of this MPA flow pattern was important to enable accurate classification of restrictive RV physiology, because this pattern has been described as a normal finding during inspiration in normal controls. We did not use the stricter definition of Vukomanovic et al. that requires antegrade flow into the MPA of >30 cm/sec for five heartbeats. Patients were assigned to one of two groups, those with restrictive RV physiology and those with nonrestrictive physiology, for later analyses.


Myocardial Mechanics
Velocity Vector Imaging technology (Siemens Medical Solutions USA, Inc.), based on speckle-tracking, was used to analyze global and regional RV myocardial motion. These analyses were performed on high-quality, digitally acquired two-dimensional echocardiographic images of the right ventricle (single-beat acoustic capture from the apical four-chamber view with a frame rate of 70–120 frames/cardiac cycle). Digital clips that clearly visualized the RV endocardium were chosen, from which the subendocardial border was manually traced and automatically tracked by syngo Velocity Vector Imaging technology, according to methods described by Kutty et al. , with the frame of reference located at the RV apex. Tracings were independently reviewed by a second reader to optimize accuracy in tracking the endocardial border. Measurement of regional peak longitudinal tissue velocities in systole and diastole, peak longitudinal systolic RV strain, peak longitudinal systolic and diastolic RV strain rates, peak longitudinal displacement of the RV free wall, and times to these peak parameters were performed for the RV free wall segments ( Figure 3 ). Because the interventricular septum is shared with the left ventricle, this research focused on the RV free wall segments.

Statistical Analyses
Medians and ranges are given for continuous demographic variables and frequencies and percentages for categorical variables. Pearson’s correlation coefficients were calculated between two continuous variables. Continuous data were compared for the groups with restrictive versus nonrestrictive RV physiology after TOF repair using Wilcoxon-Mann-Whitney tests if the data were skewed and t tests otherwise. Fisher’s exact tests were used to compare categorical variables between the two groups. Given the large number of comparisons made, P values < .005 were considered significant. This is not an adjustment for a specific number of tests, interrelated or otherwise, but rather a realization that a P value < .05 is too generous a level, but a full Bonferroni adjustment (for >100 comparisons) would be far too stringent and lead to many false-negatives, given the sample size. A P value < .05 shows an interesting trend, possibly worthy of additional study in future research. SAS version 9.1 (SAS Institute Inc., Cary, NC) was used for statistical analyses.
Results
Clinical Status
Twenty-nine patients (median age, 12.0 years; range, 8.0–33.0 years; nine male patients) with TOF (86% with pulmonary stenosis and 14% with pulmonary atresia) and at least mild PI after repair were prospectively studied from February 2008 to August 2009. Twelve patients had restrictive RV physiology, while 17 had nonrestrictive RV physiology. Overall, the median time since initial repair was 12.0 years (range, 5.0–27.0 years) and since the last operation was 10.0 years (range, 1.0–25.0 years). The median time between CMR imaging and echocardiography was 43 days (range, 0–91 days), with clinic visits, BNP assessments, and stress testing occurring at the time of either of these imaging studies. The presence of restrictive physiology appeared more prevalent in those who underwent transannular patch repair ( P = .048). Restrictive RV physiology was not dependent on age, sex, body surface area, initial diagnosis, history of prior palliation with aortopulmonary shunt, the initial type of operative repair, or the number of operations ( Table 1 ). Clinical status was not significantly different between postoperative TOF patients with restrictive physiology and those with nonrestrictive physiology ( Table 2 ).
Variable | Restrictive physiology ( n = 12) | Nonrestrictive physiology ( n = 17) | P |
---|---|---|---|
Sex | .422 † | ||
Male | 5 (41.7%) | 4 (23.5%) | |
Female | 7 (58.3%) | 13 (76.5%) | |
Age (y) | 11.5 (9.0–33.0) | 14.0 (8.0–24.0) | .964 ∗ |
Height (cm) | 149.5 (130–169) | 150.6 (125–189) | .825 ∗ |
Weight (kg) | 37.0 (25.0–70.0) | 46.4 (22.5–84.4) | .580 ∗ |
BSA (m 2 ) | 1.22 (0.96–1.73) | 1.43 (0.91–2.04) | .707 |
Diagnosis | .773 † | ||
TOF/PS | 11 (91.7%) | 13 (76.5%) | |
TOF/PAt | 1 (8.3%) | 3 (17.6%) | |
TOF absent valve | 0 (0.0%) | 1 (5.9%) | |
Initial palliation with BT shunt | .471 † | ||
Yes | 6 (50.0%) | 6 (35.3%) | |
No | 6 (50.0%) | 11 (64.7%) | |
First repair at initial operation | 6 (50.0%) | 11 (64.7%) | .783 † |
TAP | 4 (33.3%) | 4 (23.5%) | |
Non TAP | 0 (0.0%) | 2 (11.8%) | |
RV-PA conduit | 1 (8.3%) | 2 (11.8%) | |
Surgical valvotomy | 1 (8.3%) | 1 (5.9%) | |
Transatrial muscle resection | 0 (0.0%) | 2 (11.8%) | |
Surgical status at time of CMR imaging | .048 † | ||
TAP | 9 (75.0%) | 4 (23.5%) | |
Non TAP | 0 (0.0%) | 3 (17.6%) | |
RV-PA conduit | 2 (16.7%) | 7 (41.2%) | |
Surgical valvotomy | 1 (8.3%) | 3 (17.6%) | |
Number of operations | .469 † | ||
1 | 6 (50.0%) | 9 (52.9%) | |
2 | 5 (41.7%) | 4 (23.5%) | |
3 | 1 (8.3%) | 4 (23.5%) | |
Time since initial repair (y) | 10.5 (9.0–27.0) | 12.0 (5.0–23.0) | .947 ∗ |
Age at first full repair (y) | 1.02 (0.03–3.19) | 0.93 (0.07–4.11) | .982 |
Time since last operation (y) | 10.5 (7.0–25.0) | 10.0 (1.0–21.0) | .424 ∗ |
Medications | |||
None | 12 (100.0%) | 16 (94.1%) | |
Digoxin | 0 (0.0%) | 1 (5.9%) |
Variable | Restrictive physiology ( n = 12) | Nonrestrictive physiology ( n = 17) | P |
---|---|---|---|
BNP (pg/mL) | 19 (4–128) | 12 (4–42) | .170 ∗ |
Resting QRS duration (msec) | 142 (80–175) | 140 (80–180) | .704 ∗ |
V o 2 max (%) | 76 (48–116) | 66 (48–94) | .143 ∗ |
Endurance (exercise time) (min) | 11.0 (4.6–13.8) | 9.6 (6.9–15.5) | .132 ∗ |
Time 50th percentile (%) | 98.5 (48–125) | 95.0 (68–130) | .580 ∗ |
NYHA class | .187 † | ||
I | 11 (91.7%) | 11 (64.7%) | |
II | 1 (8.3%) | 6 (35.3%) |
CMR Imaging
Although patients with restrictive RV physiology had more PI (46% vs 28%, P = .002) and a trend toward slightly larger RV end-diastolic volume indices (128 vs 98 mL/m 2 , P = .046), no differences were detected in RV mass index, RV EF, or LV EF ( Table 3 ). No CMR parameter correlated significantly with exercise. Rare subjects had branch pulmonary artery narrowing detected by CMR imaging. Right pulmonary artery narrowing was seen in one subject with nonrestrictive physiology and in none with restrictive physiology, while left pulmonary artery narrowing was seen in one subject from each group.
Variable | Restrictive physiology ( n = 12) | Nonrestrictive physiology ( n = 17) | P ∗ |
---|---|---|---|
RV EF (%) | 58 (47–73) | 57 (44–80) | .773 |
RV end-diastolic volume index (mL/m 2 ) | 128 (82–166) | 98 (63–180) | .046 |
RV mass index (g/m 2 ) | 44 (34–60) ( n = 10) | 37 (24–53) | .059 |
LV EF (%) | 63 (48–71) | 64 (51–74) | .191 |
PI fraction by flow (%) | 46 (27–60) | 28 (4–52) | .002 † |
Echocardiography
Echocardiographic evaluation, using available tricuspid regurgitant jet, showed similar median RV pressures for those with restrictive RV physiology ( n = 10; 29.5 mm Hg; range, 20–68 mm Hg) and those with nonrestrictive RV physiology ( n = 14; 29 mm Hg; range, 18–72 mm Hg) ( P = .883). Using traditional Doppler and Doppler tissue imaging methods, there was little difference in diastolic function between groups, with only a trend toward greater tricuspid annular early diastolic velocity in the restrictive group ( Table 4 ). Doppler tissue imaging data had interpretable tracings for all patients except but one subject with nonrestrictive physiology. The average residual RV outflow tract gradient, by continuous-wave Doppler, was no different between groups (17.5 ± 9.5 mm Hg in patients with restrictive physiology vs 25.5 ± 21.2 mm Hg in those with nonrestrictive physiology, P = .376). Assessment of LV diastolic function by Doppler tissue imaging showed no differences between the two groups ( Table 5 ).
Variable | Restrictive physiology ( n = 12) | Nonrestrictive physiology ( n = 17) | P ∗ | Normal values | |
---|---|---|---|---|---|
10–13 y | 14–18 y | ||||
Peak E velocity for tricuspid inflow (cm/sec) | 65.7 ± 16.9 | 68.8 ± 17.3 | .636 | 59.6 ± 11.4 | 60.4 ± 10.9 |
Peak A velocity for tricuspid inflow (cm/sec) | 45.0 ± 15.1 | 45.0 ± 15.6 | .992 | 39.2 ± 11.3 | 34.5 ± 11.2 |
E/A ratio for tricuspid inflow | 1.57 ± 0.59 | 1.69 ± 0.66 | .616 | 1.61 ± 0.47 | 1.88 ± 0.56 |
Early diastolic tricuspid annular velocity (E′) (cm/sec) | 14.6 ± 2.6 | 12.4 ± 2.8 | .044 | 16.5 ± 3.1 | 16.7 ± 2.8 |
Diastolic velocity (A′) with atrial contraction (cm/sec) ( n = 16) | 7.0 ± 2.2 | 7.0 ± 1.5 | >.999 | 10.3 ± 3.4 | 10.1 ± 2.6 |
Systolic annular motion (S′) (cm/sec) ( n = 16) | 9.5 ± 2.2 | 9.1 ± 1.7 | .556 | 13.9 ± 2.4 | 14.2 ± 2.3 |
Isovolumic contraction time (msec) | 112.5 ± 18.5 | 118.2 ± 34.5 | .606 | 98.1 ± 21.7 | 101.9 ± 20.4 |
Isovolumic relaxation time (msec) | 77.9 ± 25.7 | 76.9 ± 18.3 | .896 | 61.7 ± 19.9 | 62.9 ± 18.9 |
E/E′ ratio | 4.7 ± 1.7 | 5.7 ± 1.3 | .105 | 3.5 ± 1.4 | 3.8 ± 1.4 |
Variable | Restrictive physiology ( n = 12) | Nonrestrictive physiology ( n = 17) | P ∗ | Pediatric normal values | |
---|---|---|---|---|---|
10–13 y | 14–18 y | ||||
Peak E velocity for mitral inflow (cm/sec) | 110.3 ± 26.2 | 109.9 ± 23.4 | .982 | 94.5 ± 16.0 | 90.3 ± 17.8 |
Peak A velocity for mitral inflow (cm/sec) | 41.6 ± 9.8 | 49.5 ± 12.6 | .142 | 49.5 ± 13.8 | 45.5 ± 13.2 |
E/A ratio for mitral inflow | 2.78 ± 0.97 | 2.34 ± 0.80 | .156 | 2.02 ± 0.58 | 2.13 ± 0.65 |
Early diastolic mitral annular velocity (E′) (cm/sec) | 21.1 ± 4.1 | 19.4 ± 4.1 | .161 | 19.6 ± 3.4 | 20.6 ± 3.8 |
Diastolic velocity (A′) with atrial contraction (cm/sec) ( n = 16) | 7.0 ± 2.6 | 7.6 ± 1.4 | .446 | 6.4 ± 1.8 | 6.7 ± 1.6 |
Systolic annular motion (S′) (cm/sec), ( n = 16) | 9.2 ± 2.0 | 9.5 ± 2.0 | .777 | 10.8 ± 2.9 | 12.4 ± 2.9 |
Isovolumic contraction time (msec) | 102.5 ± 23.0 | 101.9 ± 22.1 | .943 | 76.6 ± 16.2 | 78.9 ± 15.4 |
Isovolumic relaxation time (msec) | 62.1 ± 15.4 | 65.9 ± 15.4 | .513 | 62.6 ± 12.4 | 69.5 ± 15.5 |
E/E′ ratio | 5.4 | 5.8 | .44 | 4.9 ± 1.3 | 4.7 ± 1.3 |
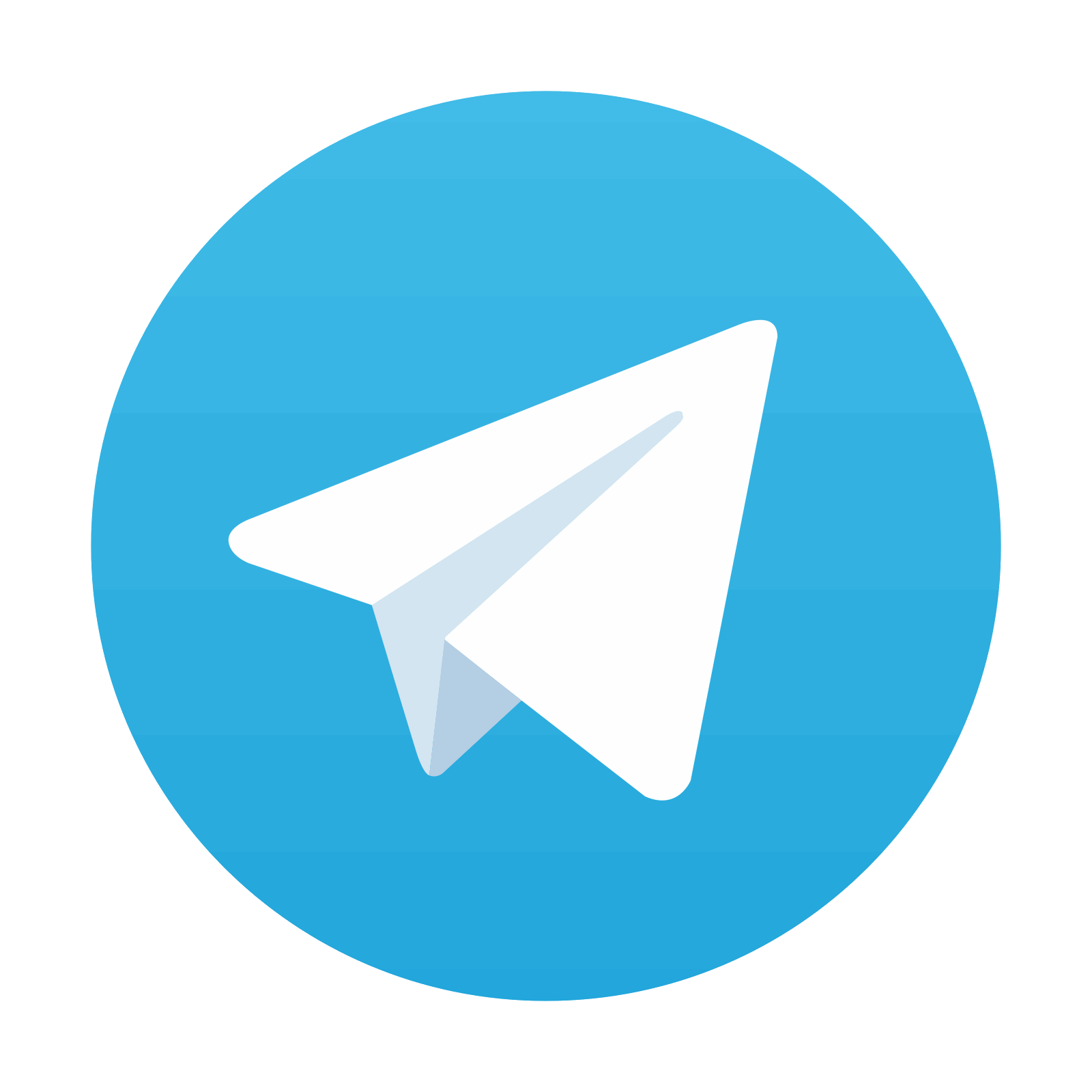
Stay updated, free articles. Join our Telegram channel

Full access? Get Clinical Tree
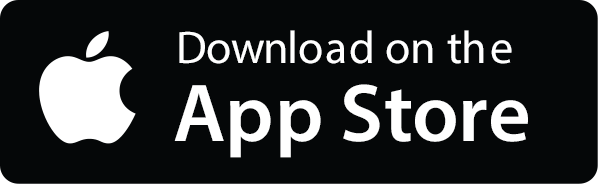
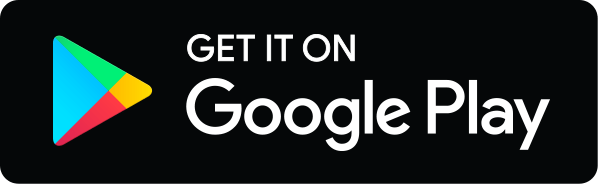
