Background
Acute myocarditis (AM) often involves the left ventricular (LV) subepicardium that might be displayed by cardiac magnetic resonance even late after the acute phase. In the absence of global or regional LV dysfunction, conventional transthoracic echocardiography (TTE) does not accurately identify tissue sequelae of AM. We sought to evaluate the diagnostic value of two-dimensional (2D) and three-dimensional (3D) speckle-tracking echocardiography to identify patients with a history of AM with preserved LV ejection fraction (LVEF).
Methods
Fifty patients (group 1: age, 31.4 ± 10.5 years; 76% males) with a history of cardiac magnetic resonance–confirmed diagnosis of AM (according to the Lake Louise criteria) were retrospectively identified and then (21.7 ± 23.4 months later) evaluated by complete echocardiography including 2D and 3D speckle-tracking analysis, as well as 50 age- and gender-matched healthy controls (group 2: age, 31.2 ± 9.5 years: 76% males). Patients with a history of severe clinical presentation of AM (sudden death, ventricular arrhythmia, heart failure, alteration of LVEF) were excluded.
Results
At diagnosis, peak troponin and C-reactive protein were 11.97 (interquartile range, 4.52-25.92) μg/L and 32.3 (interquartile range, 14.85-70.45) mg/L, respectively. Mean delay between acute phase and follow-up study TTE was 21.7 ± 23.4 months. LVEF was not statistically different between groups (62.1% vs 63.5%, P = .099). Two-dimensional global longitudinal strain (GLS) was lower in magnitude in group 1 (−17.8% vs −22.1%, P < .0001) as were 2D layer-specific subepicardial GLS (−15.4% vs −19.7%, P < .0001) and subendocardial GLS (−20.71% vs −25.08%, P < .0001). Three-dimensional global longitudinal, circumferential, area, and radial strains were lower in magnitude in group 1 (−11.80% vs −14.98%, P < .0001; −12.57% vs −15.12%, P < .0001; −22.28% vs −25.87%, P < .0001; 31.47% vs 38.06%, P < .0001, respectively). Receiver operating characteristic curve analysis showed that subepicardial GLS displayed a better diagnostic performance to detect sequelae of AM as compared with GLS (area under the curve = 0.97 vs 0.93, P = .045).
Conclusions
In patients with a history of AM, a subtle LV dysfunction can be detected by 2D and 3D speckle-tracking echocardiography, even though LVEF is conserved, adding incremental information over conventional TTE.
Highlights
- •
In patients with a history of AM, a subtle myocardial dysfunction can be detected even late after the episode.
- •
Both LV and right ventricular strain parameters are altered compared with healthy controls.
- •
Layer-specific 2DSTE is useful to detect subepicardial alteration of LV longitudinal function.
- •
These tools represent a potential novel approach for noninvasive multimodality evaluation of AM patients.
The diagnosis of acute myocarditis (AM) can be challenging due to the lack of specific diagnostic features. Clinical presentation of AM is heterogeneous and includes asymptomatic, pseudo acute coronary syndrome, severe acute heart failure, ventricular arrhythmia, and sudden death. The disease remains sometimes unrecognized, therefore the real incidence of nonfatal myocarditis is likely greater than actually diagnosed. Prognosis in myocarditis patients also varies. AM has been reported in up to 12% of cases of sudden cardiac death, and long-term follow-up studies have documented the development of secondary dilated cardiomyopathy in up to 30% of patients. Little is known about the outcome of patients with mild cases of AM with preserved left ventricular (LV) function, but scarce data support a benign prognosis of this condition. Historically, the diagnostic gold standard of AM relies on endomyocardial biopsy. However, endomyocardial biopsy is employed only in severe AM including heart failure or LV dysfunction since serious complications may occur after such invasive strategy. The current reference standard for noninvasive diagnosis of myocarditis is cardiac magnetic resonance (CMR) imaging according to the Lake Louise consensus criteria. This modality enables the disclosure of myocardial damage, which typically involves the subepicardium. Late gadolinium enhancement (LGE) reflects irreversible myocardial injury (i.e., necrosis and fibrosis). Conventional two-dimensional (2D) echocardiography has traditionally played a limited role in the diagnostic workup of AM. The advent of novel imaging modalities such as 2D and three-dimensional (3D) speckle-tracking has expanded the scope of echocardiography, providing accurate assessment of LV global and regional functions with a possibility to detect subtle LV global and regional dysfunctions. Recent software allows a layer-specific analysis of myocardial deformation with separation of endocardial and epicardial strains. Whether or not these new imaging techniques have incremental value over conventional echocardiography in the workup of myocarditis remains unclear.
We hypothesize that layer-specific 2D and 3D speckle-tracking echocardiography (2DSTE, 3DSTE) would help to discriminate patients with normal LV ejection fraction (LVEF) and a history of AM from healthy controls.
Material and Methods
Study Population
We retrospectively identified patients admitted between 2008 and 2015 in our institution for AM, confirmed by the Lake Louise CMR criteria. Patients with history of severe clinical presentation (sudden death, ventricular arrhythmia, heart failure, alteration of LVEF, dilated cardiomyopathy) were excluded as well as patients with age <18 years or poor visual analysis of LV wall motion by TTE (two or more uninterpretable LV segments). Patients underwent CMR at the acute phase and were reevaluated remotely by complete echocardiography. Age- and sex-matched subjects (normal volunteers, with no history of prior cardiac disease) were recruited to compose a control group. All the patients, including control patients, gave written informed of consent at the time of echocardiography to participate in the study, which was approved by our Institutional Review Board.
Cardiac Magnetic Resonance
CMR was performed in the first few days following the acute episode of AM on a 1.5 Tesla (Aera XQ MRI, Siemens, Erlangen, Germany) or a 3 Tesla (Achieva 3.0 T X-series, Phillips Medical Systems, Best, The Netherlands) imaging system equipped with a dedicated six-channel cardiac coil. The CMR imaging protocol included was standardized and included T2-weighted sequences in the search for myocardial edema, and postcontrast (Gadovist at a dose of 0.1 mmol/kg, Bayer Healthcare, Leverkusen, Germany) early-gadolinium enhancement T1-weighted fast spin echo sequences and 3D LGE inversion recovery gradient echo sequences (spatial resolution, 1-2 mm) in the search for myocardial hyperemia and necrosis/fibrosis, respectively. The interpretation of LGE was based on magnitude LGE images and confirmed with a phase-sensitive reconstruction. Hyperenhancement (with a nonischemic pattern) was considered positive if present in two orthogonal views. The diagnosis of AM was made according to Lake Louise consensus criteria. The localization of LGE was defined by using a 17-segment tomographic standard model.
Echocardiography
Unlike the CMR study, which was performed in the acute phase of AM, echocardiography was performed at the time of study inclusion, at a mean period of time of 22 ± 23 months after initial diagnosis of AM, using a Vivid S9 Ultrasound Machine and a M5Sc transducer (GE Vingmed Ultrasound AS, Horten, Norway). Routine 2D grayscale cine loops were obtained in end-expiratory apnea from the parasternal views and three apical (four-chamber, two-chamber, and three-chamber) views and stored digitally. Frame rates were higher than 60 Hz. Echocardiographic recordings were analyzed offline by two experienced observers (T.C., M.F.) blinded to patient data, using commercially available software (EchoPAC version 113, GE Vingmed Ultrasound AS). Each observer independently studied one batch including 25 patients from each group (25 AM and 25 controls), and each batch of 50 patients was analyzed in a random order. LV volumes and ejection fractions were assessed by the apical biplane Simpson method.
Two-Dimensional STE
The myocardial strain was evaluated on a frame-by-frame basis by automatic tracking of acoustic markers (speckles) throughout the cardiac cycle. The myocardial borders were traced from the three apical views in the end-systolic frame of the 2D images for analysis of the longitudinal strain. Region of interest width was manually adapted if necessary so as to exclude pericardium from analysis.
The peak systolic strain was defined as the maximum value of the peak negative strain (myocardial shortening) or peak positive strain (myocardial lengthening) during systole. The peak systolic longitudinal strain was assessed in all 17 longitudinal LV segments, and the segmental values were averaged to give the global longitudinal strain (GLS). Right ventricular (RV) longitudinal strain was assessed in apical four-chamber view and obtained by averaging all six segments of RV free wall and septum (RV GLS) or only the three free wall RV segments (RV free wall GLS). Postsystolic shortening (PSS) was defined as the segmental shortening in diastole beyond minimum systolic segment length (peak negative strain in diastole minus peak negative strain in systole). If minimum segment length was within systole, PSS was zero by definition. Therefore, PSS could only take negative values. Early systolic lengthening was defined as peak positive early strain (representing maximum segmental systolic lengthening). Two-dimensional strain parameters were assessed both in the subendocardial (internal) layer of the myocardium and in the subepicardial (external) layer of the myocardium.
Three-Dimensional STE
All examinations were performed with Vivid E9 (GE-Healthcare, Horten, Norway) echocardiographic system equipped with the 4-V phased-array matrix transducer. Six electrocardiogram-gated consecutive beats subvolumes were acquired from the apical approach using second-harmonic imaging during a single breath hold to generate the pyramidal full-volume data set. Acquisitions were performed at the highest possible frame rate (>20 frames/sec), and care was taken to avoid LV foreshortening and encompass the entire LV cavity within the data set. The quality of the acquisitions was then verified in each patient by selecting the multislice display mode available on the machines to ensure optimal imaging of the entire LV wall at each short-axis level. If it was considered suboptimal, the data set was reacquired. Data sets were stored digitally in raw data format and exported to a separate workstation equipped with the dedicated post-treatment software (EchoPAC v113) for offline analysis of 3DSTE LV myocardial deformation measurements, LV volumes, mass, and ejection fraction. A single experienced investigator (T.C.) analyzed the data sets in random order, unaware of the identity of the patients. Data sets were analyzed using the 4DAutoLVQ package (EchoPAC v113, GE Vingmed Ultrasound AS). The end-diastolic frames needed for contour detection were automatically displayed in a quad view. Manual alignment by pivoting and translating the four-chamber plane was performed to align the three apical views so that the corresponding intersection line of all planes was placed in the middle of the LV cavity and crossing the LV apex and the center of mitral valve in each view. Manual input of two reference points (at the apex and at the tip of the mitral leaflet) on the end-diastolic and end-systolic frames of the four-chamber view slice was performed. The software automatically detected LV cavity endocardial border in 3D and provided the measured LV volumes. If endocardial border detection was judged unsatisfactory by the operator, LV endocardial borders were manually adjusted in a multiplanar layout by a point-click method. After LV volumes and ejection fraction measurements, an automatic trace of the epicardial border was displayed to identify the region of interest required for LV mass and myocardial deformation measurements by 3DSTE. The GLS, global circumferential strain (GCS), global area strain (GAS), and global radial strain (GRS) were calculated as weighted averages of the regional values from the 17 myocardial segments and presented as color-coded bull’s-eye plot. Epicardial trace could be manually adjusted with the same point-click method. If more than three segments were rejected, global strain values were not calculated and these patients ( n = 6, three AM patients and three controls) were excluded from the final analysis.
Reproducibility of Strain Values
Two- and three-dimensional strain analyses were performed repeatedly by two independent observers on 10 randomly selected patients, and the reproducibility of the echocardiographic parameters was evaluated by determining the intraclass correlations for intraobserver and interobserver variability. These were, respectively, 99% and 96% for 2D GLS, 96% and 93% for epicardial GLS, and 95% and 83% for 3D GLS.
Statistics
Normal distribution of continuous variables was checked with Kolmogorov-Smirnov one-sample test. Results are expressed as the mean ± SD for continuous normally distributed variables, while categorical variables are expressed as frequencies. Continuous variables were compared using Student’s t -test, and categorical variables were analyzed using Fisher’s exact test. Receiver operating characteristic (ROC) curve analyses were performed in order to determine the ability of GLS, endocardial GLS, epicardial GLS, 3D GLS, 3D GCS, 3D GSS, and 3D GRS to discriminate AM patients from controls. The area under the curve (AUC) and the ROC curves were calculated. The ROC curve areas were compared according to Hanley and McNeil, the P values were two-tailed, and values <.05 were considered to be significant. Statistical analyses were performed using the software SPSS version 20 (SPSS Inc., Chicago, IL) and Medcalc version 13.1.1 (Medcalc, Ostend, Belgium).
Results
Clinical Characteristics
Patients with myocarditis were all symptomatic at the acute phase with chest pain in 94%, arrhythmia and/or lipothymia in 4%, and heart failure in 2%. An infectious disease context was present in 56%, like ear, nose, and throat viral disease, flu symptoms, or gastroenteritis. Electrocardiogram displayed ST segment elevation in 70%, negative T wave in 22%, and PQ segment depression in 20%. Electrocardiogram was normal in 14%. Peak troponin was 11.97 (interquartile range [IQR], 4.52-25.92) μg/L and peak C-reactive protein was 32.3 (IQR, 14.85-70.45) mg/L. Patients were treated by aspirin/nonsteroidal anti-inflammatory drugs in 50% of cases.
Patients from the myocarditis group were reevaluated at a mean follow-up of 22 ± 23 months (median time of 12 months; IQR, 7-42). At that time, all the patients were asymptomatic. Of note, two of them (4%) presented a recurrent episode of AM in the interval.
The myocarditis group was matched to a control group, and there were no statistically clinical differences between groups with respect to clinical characteristics ( Table 1 ), LVEF, wall motion score index, or LV and left atrial sizes ( Table 2 ). However, the E/e’ ratio was higher in the myocarditis group ( Table 2 ).
Myocarditis ( N = 50) | Control ( N = 50) | P | |
---|---|---|---|
Age (years) | 31.4 ± 10.5 | 31.2 ± 9.5 | .91 |
Male | 38 (76%) | 38 (76%) | ≥ .99 |
Smoker | 18 (36%) | 12 (24%) ∗ | .19 |
Hypertension | 2 (4%) | 1 (2%) † | .56 |
Dyslipidemia | 3 (6%) | 0 (0%) | .08 |
Diabetes mellitus | 0 (0%) | 0 (0%) | ≥ .99 |
Overweight/obesity | 4 (8%) | 1 (2%) † | .17 |
Previous myocarditis | 3 (6%) | 0 (0%) | .08 |
∗ Mean pack-years of 5 ± 2 among the 12 smokers of the control group.
† The same individual from the control group suffered from obesity and hypertension.
Myocarditis ( N = 50) | Control ( N = 50) | P | |
---|---|---|---|
Heart rate (bpm) | 70 ± 11.2 | 73 ± 12.3 | .3 |
LVEF (%) | 62.1 ± 3.7 | 63.5 ± 3.8 | .099 |
WMSi | 1 | 1 | ≥ .999 |
LVEDD 2D (mm/m 2 ) | 25.6 ± 2.5 | 26.5 ± 2.9 | .134 |
LVEDV 3D (mL/m 2 ) | 60.3 ± 13.5 | 57 ± 10 | .219 |
LV mass 3D (g/m 2 ) | 66.7 ± 6.9 | 67.8 ± 9.1 | .54 |
Valvulopathy | 0 (0%) | 1 (2.5%) | .494 |
Cardiac index (L/min/m 2 ) | 3.03 ± 0.8 | 3.05 ± 0.7 | .916 |
Pericardial effusion | 0 | 0 | ≥ .999 |
PASP (mmHg) | 23.2 ± 4.2 | 23.5 ± 3.5 | .747 |
LAV (mL/m 2 ) | 24.3 ± 7.6 | 21.4 ± 5.2 | .054 |
E/e’ | 5.4 ± 1.2 | 4.6 ± 0.9 | .001 |
RV DTI S’ (cm/sec) | 13.2 ± 2 | 14.2 ± 2.1 | .028 |
RV global strain (%) | −20.6 ± 3.5 | −22.9 ± 3.3 | .003 |
RV free wall strain (%) | −24.6 ± 5.4 | −27.2 ± 4.3 | .023 |
GLS (%) | −17.8 ± 1.7 | −22.1 ± 2.2 | .0001 |
GLS epicardial (%) | −15.4 ± 1.5 | −19.7 ± 1.8 | .0001 |
GLS endocardial (%) | −20.7 ± 2.2 | −25.1 ± 2.6 | .0001 |
Cardiac Magnetic Resonance
The diagnosis of AM was confirmed in all the patients by CMR. A total of 177 myocardial segments (mean of 3.5 ± 1.5 segments per AM patient) showed LGE with LV inferolateral and anterolateral walls being the most affected ( Table 3 ). LGE pattern was subepicardial in 167 segments and midmural in 10 segments.
Regional strain | According to CMR LGE | P | |
---|---|---|---|
Myocardial segment | Absence of LGE | Presence of LGE | |
1 | −16.8 ± 4.9 ( n = 34) | −14 ± 4.4 ( n = 7) | .17 |
2 | −15.2 ± 2.7 ( n = 37) | −14 ± 0 ( n = 2) | .51 |
3 | −14.9 ± 0.5 ( n = 38) | −15 ± 0 ( n = 1) | .98 |
4 | −18.3 ± 4.0 ( n = 27) | −17.2 ± 3.4 ( n = 12) | .39 |
5 | −17.3 ± 3.4 ( n = 16) | −16.6 ± 2.6 ( n = 23) | .47 |
6 | −16.9 ± 5.1 ( n = 22) | −15.8 ± 2.6 ( n = 17) | .40 |
7 | −17.9 ± 3.9 ( n = 37) | −18.2 ± 1.7 ( n = 4) | .85 |
8 | −19.0 ± 2.5 ( n = 36) | −20.7 ± 0.6 ( n = 3) | .26 |
9 | −18.7 ± 2.2 ( n = 37) | −20.5 ± 0.8 ( n = 2) | .26 |
10 | −18.4 ± 7.9 ( n = 29) | −19.7 ± 1.9 ( n = 10) | .62 |
11 | −19.3 ± 3.4 ( n = 13) | −15.3 ± 7.8 ( n = 26) | .08 |
12 | −18.6 ± 3.8 ( n = 15) | −17.7 ± 3.5 ( n = 24) | .49 |
13 | −19.1 ± 3.7 ( n = 31) | −18.9 ± 3.6 ( n = 8) | .88 |
14 | −21.6 ± 3.5 ( n = 34) | −22.4 ± 2.6 ( n = 4) | .62 |
15 | −21.4 ± 3.6 ( n = 31) | −20.6 ± 2.6 ( n = 8) | .58 |
16 | −19.6 ± 3.8 ( n = 18) | −19.4 ± 3.9 ( n = 21) | .89 |
17 | −20.2 ± 3.12 ( n = 35) | −21.7 ± 2.8 ( n = 4) | .34 |
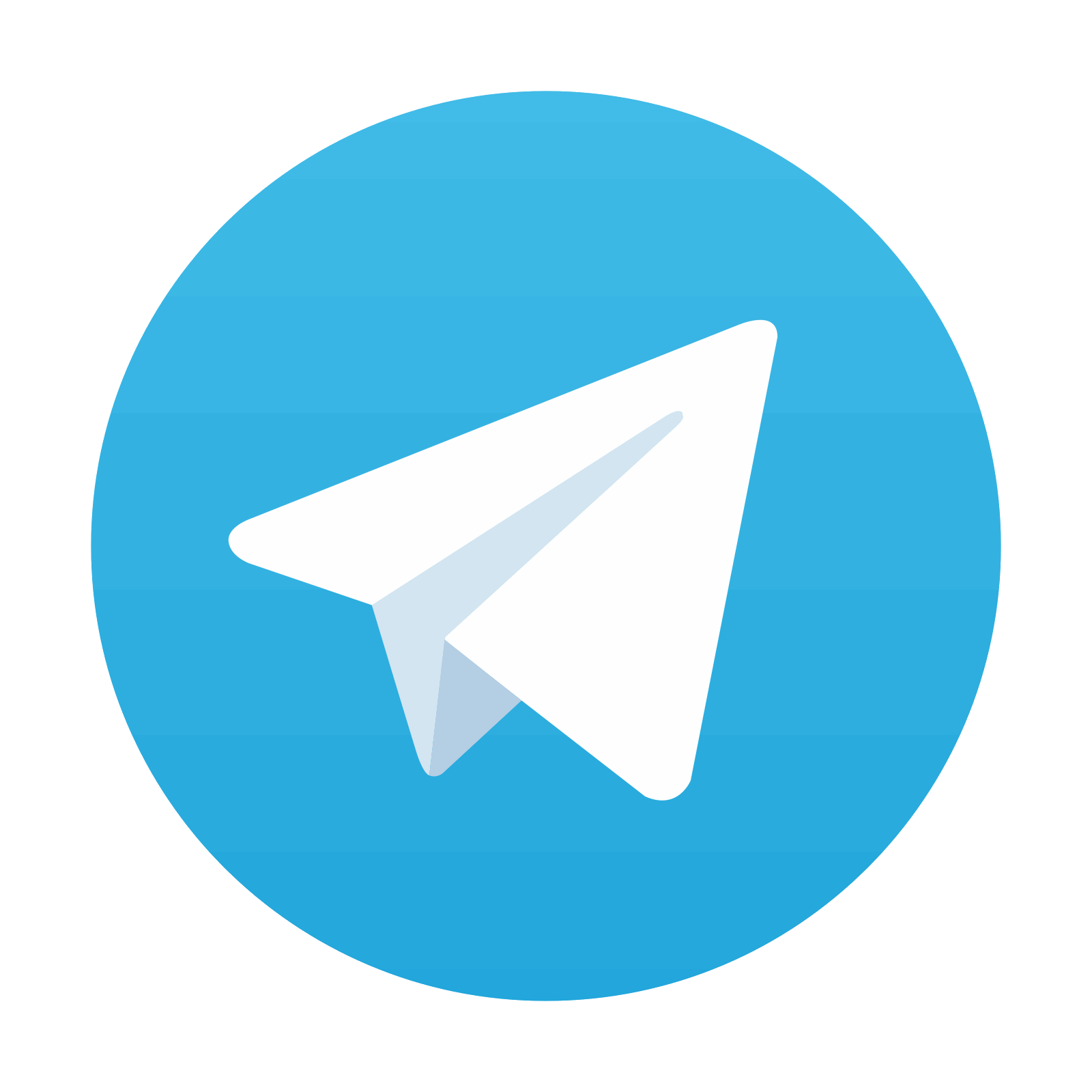
Stay updated, free articles. Join our Telegram channel

Full access? Get Clinical Tree
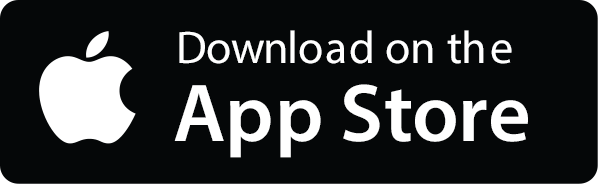
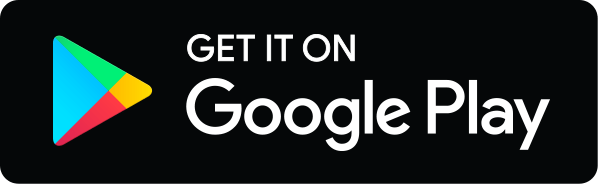
