Ali Dodge‐Khatami1 and Christopher E. Greenleaf2 1University of Aachen RWTH, Aachen, Germany 2University of Texas Health Science Center, Houston, TX, USA Isolated right ventricular outflow tract obstruction (RVOTO) is a spectrum diagnosis with heterogeneous morphology and pathophysiology, leading to equally wide choices of surgical and catheter‐based therapies, whose goals range from achieving biventricular repair to single ventricular palliation, en passant by the grayer zone of one‐and‐a‐half repair (1.5VR). The lesions include anything from mild pulmonary valve stenosis to pulmonary atresia with intact ventricular septum (PA‐IVS), a hypoplastic right ventricle (RV), and right ventricular–dependent coronary circulation (RVDCC). This chapter will address those lesions with RVOTO without a ventricular septal defect and normal ventriculo‐arterial connections, which include PA‐IVS, neonatal critical pulmonary stenosis, supravalvar, valvar, and infundibular pulmonary stenosis. Pulmonary atresia with intact ventricular septum is characterized by an imperforate pulmonary valve with no antegrade flow from the RV to the pulmonary arteries, varying degrees of RV development, and no ventricular septal defect (Figure 20.1). It was first described in 1784, and is frequently associated with a dysplastic or Ebstein‐like tricuspid valve (TV), and abnormal ventriculo‐coronary connections [1]. Similar to the broad diagnosis of isolated RVOTO, PA‐IVS has an equally diverse pathophysiology leading to complex surgical management decisions pre‐, intra‐, and postoperatively [2]. PA‐IVS is a rare lesion, with an incidence of approximately 4 cases per 100,000 live births, representing only about 1–3% of all congenital cardiac lesions [1–3]. This may underestimate the real incidence because of elective terminations [4]. The cause of PA‐IVS is unknown. Kutsche and Van Mierop postulate that this lesion occurs some time after cardiac septation completion, late in development [3]. They also surmise that the development is due to a prenatal inflammatory process. Fetal echocardiography studies identify antegrade flow across the pulmonary valve in patients that eventually progresses to PA‐IVS [5]. During cardiac development, antegrade flow across the TV will lead to varying amounts of RV growth, before the pulmonary valve closes and becomes atretic. The later the pulmonary valve closes during fetal growth, the more the RV will be developed [5]. There have been studies looking at a potential genetic link between patients with PA‐IVS, suggesting an autosomal recessive trait [4], or an autosomal dominant inheritance with incomplete penetrance [6]. Despite this, the vast majority of cases are sporadic. Almost all hearts with PA‐IVS have situs solitus and normal atrioventricular and ventriculo‐arterial connections [7]. Based on population‐based studies [2] and retrospective clinical series describing angiographic findings [8], atresia is valvar in 75% of cases and muscular in the remaining 25%. Typically, the RV contains the inlet, trabecular, and outlet portions, and is labeled tripartite [9, 10]. Muscular obliteration of the outflow tract and sometimes of the body of the RV results in a “bipartite” RV in 33.6% of patients, and a “unipartite” chamber in 7.7%. The remaining 58.7% have “tripartite” morphology. Coronary arterial abnormalities are found in about 46% of infants. All PA‐IVS hearts have a large atrial septal defect (ASD). Figure 20.1 Pathologic specimen from a neonate with pulmonary atresia with intact ventricular septum viewed from main pulmonary artery. Developed sinuses and valve commissures are evident. Source: Reproduced with permission from Mavroudis C, Backer CL (eds.), Pediatric Cardiac Surgery, 3rd ed. St. Louis, MO: Mosby; 2003. Unlike pulmonary atresia with ventricular septal defect, the branch pulmonary arteries are usually of normal caliber. The main pulmonary artery typically tapers down to an atretic valve. There is often plate‐like membranous or valvar pulmonary atresia. This is characterized by a small valve annulus, with small but identifiable cusps and fused commissures. Infrequently, there is muscular pulmonary atresia with closure of the muscular infundibulum. This is associated with the more severe cases of RV hypoplasia. RV size varies with differing levels of muscular growth. By definition, all PA‐IVS patients have at least a tricuspid inlet portion of the RV. In some cases, there is muscular overgrowth leading to a smaller ventricular chamber and no outlet portion, or bipartite ventricle. In rare instances, there is so much overgrowth that there is no discernible outlet or trabecular portion of the ventricle, which is labeled unipartite [9]. The size of the TV annulus correlates very closely with the size of the RV, which is almost always hypertrophied and hypoplastic [7, 11]. A TV z‐score of 0 to –2 is associated with a tripartite ventricle. A TV z‐score of –2 to –4 is associated with varying degrees of RV hypoplasia. A TV z‐score of less than –4 is associated with a unipartite RV. In very rare circumstances, there is ventricular displacement of the TV similar to an Ebstein anomaly [9, 11]. A developed TV and an imperforate pulmonary valve lead to a highly pressurized RV. The elevated pressure in the RV probably leads to connections between the RV to intramyocardial sinusoids, which are reservoirs for myocardial blood flow during gestation before the development of the coronary arteries [12, 13]. The evidence for this theory is that ventriculo‐coronary fistulas only occur when there is a hypertrophied ventricle. These high‐pressure sinusoids can connect with the coronary arteries leading to coronary‐cameral fistulas, which are present in 31–68% of patients [7, 8, 14–17]. The coronary arteries themselves may be atretic or stenotic, showing evidence of myo‐intimal proliferation. If there is no aortocoronary connection or severe proximal coronary artery stenoses, then the distal coronary arteries rely exclusively on coronary‐cameral fistulas. The myocardium then relies on desaturated RV blood, which leads to a right ventricular–dependent coronary circulation (RVDCC) [7, 18]. RVDCC has been defined as having stenosis in two or more coronary arteries associated with coronary‐cameral fistulas, whereas stenosis in only one coronary artery is not sufficient to qualify as RVDCC [19], as adequate antegrade flow to at least one coronary territory is still present. Identifying this physiology must be done preoperatively to prevent inappropriate RV decompression, which would ultimately lead to myocardial ischemia and death [8, 20]. RVDCC is present in about 30% of patients with PA‐IVS, requires palliation avoiding decompression of the RV, and almost always results in single‐ventricle palliation [8, 15, 17–19, 21–23]. Most infants with PA‐IVS are born at term. As defined by their physiology, they are duct dependent and cyanotic. Hypoxia becomes completely refractory after ductal closure, and fatal without therapy [2]. On examination, there is widened pulse pressure from runoff through the patent arterial duct, a murmur of tricuspid regurgitation, and only a single second heart sound. The electrocardiogram shows left ventricular (LV) dominance, unlike the typical neonatal electrocardiogram. The chest x‐ray is often normal with a normal‐sized cardiac silhouette, unless RV dilatation has occurred. RV pressures are often systemic or even suprasystemic, unless the RV is dilated with poor function, associated with severe tricuspid regurgitation [7]. In patients with true RVDCC and an absence of antegrade coronary blood flow, keeping the RV pressures high is essential, as coronary reserve depends on desaturated blood flow and relatively low diastolic pressures. Any event leading to lower RV pressures, such as hypovolemia, anemia, tachycardia, fever [19], acute TV regurgitation induced by catheter, or relief of RV outflow obstruction, will lead to irreversible myocardial ischemia and a potentially fatal outcome. LV function is usually normal, but arrhythmia and LV dysfunction may arise in patients with suprasystemic RV pressures [21]. Figure 20.2 Right ventricular angiogram showing right ventricle–coronary sinusoids and retrograde flow from the right ventricle into the coronaries, and then into the ascending aorta. Courtesy of Dr. M.R. Ebeid, University of Mississippi Medical Center, Jackson, MS, USA. Echocardiography is the gold standard for diagnosis. It will show an imperforate RV outflow tract, ductal size, and morphology; hypoplasia of the RV; TV regurgitation and morphology; RV–coronary artery fistulas; and the atrial septum. Initial medical management must include ensuring ductal patency with a prostaglandin infusion, establishment of vascular access for resuscitation, correction of metabolic acidosis, and the initiation of inotropic support if perfusion is inadequate. In most centers, cardiac catheterization is recommended and performed to evaluate for RVDCC [8, 24] or plan an initial therapeutic procedure, depending on institutional protocols (Figures 20.2 and 20.3). The anatomy, physiology, and hence the management of this lesion are extremely heterogeneous and thereby tailored to individual situations, without true consensus among the interventional and surgical communities. There are many morphologic criteria that have been proposed to guide therapy, whose first decision‐making step aims to enhance the chance of eventually achieving biventricular repair (BVR), rather than having to accept single‐ventricle palliation. However, to date the process is still unpredictable, as no reliable way has been established to predict which RVs will grow to allow biventricular physiology, and which remain too small [25], further clouded by the middle‐zone potential for a “successful one‐and‐a‐half repair” [14]. Figure 20.3 (A, B) Right ventricular angiogram showing membranous pulmonary atresia, before and after balloon catheter perforation. Courtesy of Dr. M.R. Ebeid, University of Mississippi Medical Center, Jackson, MS, USA. Although it is intuitive that biventricular physiology should be superior to that of a single ventricle, there is no evidence specifically in patients with PA‐IVS to support this contention [26–28]. While erring on the side of single‐ventricle palliation will deprive those patients with borderline RVs the advantages of biventricular physiology, an overly aggressive strategy to push for BVR is associated with an increased risk of death [11, 14]. It is nonetheless generally assumed, with heavy institutional biases, that in gray zones with a borderline RV and TV size, and in the absence of RVDCC, decompression of the RV is performed with the hope that the RV will grow to allow biventricular physiology [21, 25]. The initial goal of therapy is to provide an alternative source of pulmonary blood flow to the patent arterial duct, so that a newborn is no longer prostaglandin dependent. This may be achieved by (i) surgical pulmonary valvotomy or hybrid balloon valvuloplasty [29, 30] to create antegrade flow from the RV to the pulmonary arteries; (ii) systemic‐to‐pulmonary artery shunting (modified Blalock–Taussig–Thomas or central shunt); (iii) interventional catheter perforation of the atretic pulmonary valve, with or without an RV outflow tract stent; (iv) interventional catheter stenting of the patent arterial duct; or (v) a combination of procedures/surgeries involving systemic‐to‐pulmonary artery shunting and promoting antegrade flow from the RV [21, 24]. In patients without RVDCC who are deemed good candidates for RV decompression, either surgically, by catheter‐based perforation, or by hybrid collaboration, protocols and practice vary with regards to associating a systemic‐to‐pulmonary artery shunt or ductal stenting [24, 29, 31]. While some groups may perform RV decompression with a concomitant shunt, others prefer RV decompression alone, an attempt at weaning prostaglandins, and adding a shunt only secondarily if hypoxemia persists [32]. Although both approaches are accepted and safe, the latter understandably tends to increase hospital length of stay. Enlarging a restrictive interatrial communication surgically by atrial septectomy or percutaneously by balloon septostomy is considered on an individual basis [11, 33]. While no physiologic contraindications to performing a shunt or patent arterial duct stenting exist [24], the same cannot be said for decompressing the RV. It is generally accepted that RV–coronary artery fistulas do not preclude RV decompression [16], but RVDCC are often cited as a contraindication to any decompression of the RV [20, 23]. Kotani and associates do not believe that RVDCC is a contraindication for RV decompression, and perform controlled pulmonary valve perforation, aiming to keep the RV/LV pressure ratio at 1.0, with satisfactory results [21]. Another reason for not preferring RV decompression relates to the need for avoiding cardiopulmonary bypass: if relative or absolute contraindications to bypass exist, hybrid strategies using sternotomy and RV access to perform catheter‐based balloon perforation–dilatation may be deployed, with encouraging results [29, 30]. Anatomic concerns with regard to performing a shunt as the initial procedure may include branch or central pulmonary artery size/morphology, anatomic head and neck vessel anomalies, or contraindications to cardiopulmonary bypass if bypass is necessary to perform the shunt. Challenges in deploying a patent arterial duct stent may include ductal morphology (shape, tortuosity, and size), periprocedural ductal spasm with hemodynamic instability, and/or issues with vascular access in small patients [24]. Experimental in utero intervention with pulmonary valve balloon dilatation has been reported in a small series, with encouraging yet indefinite results [34]. Finally, in patients with aorto‐coronary atresia leading to the most severe form of RVDCC, cardiac transplantation is recommended [8, 19], as the risk of myocardial ischemia and perioperative death is extremely high when attempting shunt procedures. In most centers, patient attrition from death while on the waiting list for an organ makes transplantation an unpractical and unattractive option for this particularly unstable subset of patients. Z‐scores of the TV on echocardiography are a fairly objective measure of RV cavity size, are even used as an index for RV growth [11], and are hence generally considered for decision‐making during definitive repair. When attempting BVR, which consists of creating or maintaining an unobstructed RV–PA (pulmonary artery) connection and closing the ASD, many use a score larger than –3 for BVR. A TV z‐score less than –8 usually points to single‐ventricle palliation, although slight cutoff variations exist [15, 17, 21, 35]. With a TV z‐score between –8 and –3, some consider a one‐and‐a‐half repair [21], consisting of maintaining or creating antegrade flow from the RV to the pulmonary arteries, associated with a bidirectional Glenn (superior cavopulmonary connection) to partially unload the RV. Concomitant to RV decompression, RV overhaul consists of performing infundibular RV muscle resection in an attempt to increase RV size [36] and, hence, render the RV more suitable for BVR; the challenge lies in resecting enough hypertrophied muscle to achieve larger RV cavities, without resecting too much muscle, which could decrease RV contraction [21, 37]. Staged decompression, which involves additional (serial) catheter pulmonary valvotomy after initial palliation, is a strategy used to enhance the chances of eventually achieving BVR, and has the potential to make RV–coronary artery fistulas regress in more than half of patients [21]. Interestingly, in their series of 32 patients undergoing the single‐ventricle pathway for PA‐IVS, Gulesarian and colleagues did not find angiographic regression of coronary‐cameral fistulas or coronary artery stenosis among 20 survivors [8]. The implication for subsequent planned second or third operations of the staged pathway suggests caution during the Glenn or Fontan operations, typically performed with cardiopulmonary bypass, with the potential to decompress the RV and precipitate myocardial ischemia from coronary steal [20]; although this phenomenon has been observed during Glenn procedures [8, 18], it has not been noted during a completion Fontan operation [8]. Veno‐venous bypass has been proposed to avoid myocardial ischemia by keeping the RV beating and filled with oxygenated blood [20]; however, Glenn and Fontan procedures have been successfully performed using standard bypass, with controlled filing and maintained ejection of the RV [8]. At mid‐ to late‐term follow‐up, overall survival has been between 81% and 88% at 1, 5, 10, and 15 years [8, 21, 25], which breaks down to 100% in those with BVR or one‐and‐a‐half repairs, versus 75% after single‐ventricle palliation [21]. Similar results were reported by Cleuziou and coworkers, with actuarial survival for all patients of 79%, 68%, and 60% at 1, 20, and 25 years, respectively [35]. In the largest series reported from the Congenital Heart Surgeons’ Society (CHSS) spanning a decade from 1987–1997, the overall survival was 77% at 1 month, 70% at 6 months, 60% at 5 years, and 58% at 15 years [14]. With morphologically driven protocols emphasizing single‐ventricle or biventricular pathways, many institutions minimize the negative effects of unfavorable morphology so that approximately 85% of neonates reach a definitive surgical end‐point [14]. The report from a population‐based study in UK and Ireland showed that low birth weight, unipartite RV morphology, and RV dilatation were independent risk factors for death [2]. Others have reported RVDCC as an independent risk factor for mortality [32]. However, neither the largest study to date from the CHSS nor the UK and Ireland registry revealed coronary aberrations to be associated with an increased mortality risk [2, 14], suggesting that even the most unfavorable of morphologies can be managed successfully when patient‐related factors are built into the protocols. With an aggressive strategy striving for biventricular repair in borderline TVs and RVs that includes both RV decompression and a systemic‐to‐pulmonary artery shunt, approximately 50–60% achieve BVR [14, 21] and another 10% one‐and‐a‐half repair, so that approximately 70% of patients can avoid single‐ventricle palliation [21]. Both the TV z‐score and RV size are important determinants of achieving BVR, and as these two variables correlate only moderately, interplay between them is important in decision‐making toward a favorable outcome [14]
CHAPTER 20
Isolated Right Ventricular Outflow Tract Obstruction
Pulmonary Atresia with Intact Ventricular Septum
Definition, Etiology
Morphology
Clinical Presentation and Initial Management
Interventional/Surgical Management
Initial Palliation
Definitive Repair, Outcomes, Late Survival
Stay updated, free articles. Join our Telegram channel

Full access? Get Clinical Tree
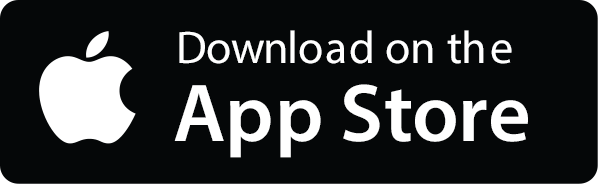
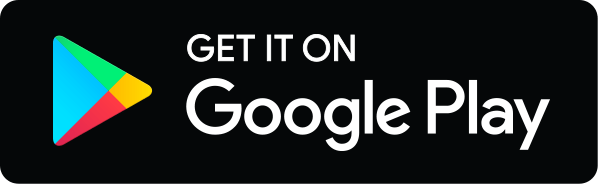