(1)
Department of Neuroscience, University of Turin Ospedale Molinette, Turin, Italy
Abstract
Atherosclerosis is the principal pathological process affecting the extracranial and intracranial arteries responsible for ischemic stroke. Less commonly, ischemic stroke is due to other conditions, such as spontaneous dissection and other nonatherosclerotic arteriopathies as well as heart disease. All of these diseases are described in Chaps. 16, 17, 18, and 19.
Atherosclerosis is the principal pathological process affecting the extracranial and intracranial arteries responsible for ischemic stroke. Less commonly, ischemic stroke is due to other conditions, such as spontaneous dissection and other nonatherosclerotic arteriopathies as well as heart disease. All of these diseases are described in Chaps. 16, 17, 18, and 19.
Atherosclerosis is a generalized condition that predominantly involves certain vascular territories: the aorta, the iliac, and the coronary and extra and intracranial arteries. It is a typical pathology of adulthood, and the likelihood of occurrence increases with the age. There is a slight predominance among men.
15.1 Pathology
Initially, there is microinjury of the endothelial cells due to various stress factors (such as elevated cholesterol, hypertension, cigarette smoking, diabetes, and homocystinuria), which promote the passage and deposit of cholesterol (especially low-density lipoproteins) in the subendothelial space. This leads to a reaction of both endothelial and smooth muscle. The metabolic products of these cells result in further damage of the endothelial, promoting the passage into the subendothelial space of monocytes, which progressively engulf lipids and become foam cells. Migration of lymphocytes, cell necrosis, and collagen production follow (Garcia et al. 1998; Witznum and Steinberg 1991; Ohara et al. 1993; Libby and Clinton 1993). All these aspects lead to the formation of the atheromatous plaque covered by a well-formed fibrous cap, which can extend progressively into the lumen of the artery, resulting in stenosis. The evolution of the plaque can vary from a stable course to a slow or rapid growth. Hemodynamically, stenosis—leading to a major reduction of flow and thereby increasing the risk of ischemia—has to be rather severe, as previously described (Brice et al. 1964; Archie and Feldtman 1981). It is, however, important to note that in a relatively high number of cases, the plaque can slowly progress up to complete occlusion but remain asymptomatic. This occurs when the collateral circulation is efficient and there is no distal embolization during the process.
Erosion of endothelial cells and breakdown of the fibrous cap lead to exposure and delivery of the material inside the plaque, promoting distal embolism (Sitzer et al. 1995). Further reduction of the lumen up to occlusion can occur because of the formation of an intraluminal thrombus or an intraplaque hemorrhage. The latter can be due to the entry of blood in the plaque through erosion of the fibrous cap or disruption of the vasa vasorum which are abundant in advanced atherosclerotic lesions (Fisher and Ojeman 1986; Bo et al. 1989; Beach et al. 1993). The improvement of the diagnostic tools allows today to study the component of the plaque giving useful information about its possible evolution (see also Sect. 15.4.1).
15.2 Location
Atherosclerosis affects mainly the extracranial segments of arteries. Especially involved are the internal carotid artery (ICA) at the bifurcation and the common carotid and vertebral arteries at their origins. Other less frequent sites are the aortic arch and subclavian arteries. Atherosclerosis of the intracranial arteries is less frequent, but not as rare as was previously supposed. According to some reports, the incidence of significant intracranial atherosclerotic stenosis in patients with transient ischemic attacks (TIAs) or stroke has been given as 4 % (Thijs and Albers 2000). The incidence of these lesions is reported to be higher among blacks and Asians (Feldmann et al. 1990; Caplan et al. 1986; Caplan 1989; Wong et al. 1998; Min et al. 2000; Thijs and Albers 2000). The most common sites visible on the angiogram are the terminal ICA and the basilar and intracranial vertebral arteries. Also involved are the first segment of the middle cerebral artery (MCA; M1), the first segment of the anterior cerebral artery (ACA; A1), the pericallosal artery around the genu of the corpus callosum, and the first segments of the posterior cerebral artery (PCA; P1–P2) (Fisher et al. 1965; Fisher and Caplan 1971; Castaigne et al. 1973, 1981; Kavase et al. 1979; Hinton et al. 1979; Bradac and Oberson 1983; Caplan et al. 1986; Caplan 1989). Today, the intracranial lesions are the object of greater attention due to the possibility, in selected cases, of endovascular treatment.
15.3 Mechanisms Leading to Ischemia
The mechanisms leading to ischemia can be grouped in three categories:
Thromboembolic occlusion or severe stenosis of arteries leads to ischemia strictly related to the supplied territory. These infarcts are also called territorial infarcts by some authors (Ringelstein et al. 1983, 1985; Weiller et al. 1991).
The occlusion or severe stenosis can be proximal and the suffering territory is more distal and not in the vascular territory of a defined artery. Instead, the infarct is located at the borders of two different vascular territories (border-zone infarcts). A typical example is a severe stenosis or occlusion of the ICA leading to ischemia; this is also termed hemodynamic because of hypoperfusion in the distal area, in which the collateral circulation is insufficient or absent. These infarcts can be cortical or involve deep and/or superficial perforators.
A particular form of infarct is the lacunar infarct or lacune (from Latin, lacuna,-ae. small cavity). The term was first used by Dechambre in 1838 and later by Durand-Fardel in 1843 to express pathological findings due to small infarcts, hemorrhages, or other causes. More precise reports were presented by Pierre Marie (1901) and Ferrand (1902), who used the term “lacune” to describe small infarcts in the basal ganglia, capsula interna, and pons that presented some typical clinical aspects. The clinical relevance, however, and the peculiar aspects of this pathology, termed lacunar syndrome, were emphasized and comprehensively discussed by Fisher (1965, 1969, 1979). CT and MRI have played an important role in the diagnosis and identification of these lesions. Today, lacunar infarcts are reported to account for about 25 % of all cerebral infarcts (Bamford et al. 1987; Bogousslavsky et al. 1988b; Boiten and Lodder 1991). Lacunar infarcts are due to involvement of the perforators (deep and superficial), which are occluded directly by lipohyalinosis or through a thromboembolic process or atheroma, involving the large parent artery from which they arise; the perforators may be also occluded indirectly in cases with hypoperfusion due to more proximal occlusion or cardiac failure.
All these mechanisms can act in isolation or be variously associated with one another. Taking this into consideration, the neuroradiological diagnosis of patients should include CT and MRI to examine the brain parenchyma, followed by a complete study of the cerebral vessels in the extra and intracranial sector. The latter can be performed today with highly accurate CT and MR angiography and for the extracranial arteries with ultrasound examinations. However, angiography should be instituted every time the diagnosis is insufficiently clear, more informations about the vessels are required or in cases where endovascular treatment is planned.
Considering, in particular, the patients with acute stroke, the type, site, and size of the ischemia, the identification of the vessels and vascular territories involved can be today studied rapidly with CT, CT perfusion, and CT angiography. These informations are essential for the therapy especially in the cases of endovascular treatment.
15.4 Mechanism of Ischemia of the Anterior Circulation
15.4.1 Carotid Artery
Severe stenosis or occlusion of the ICA is not uncommonly asymptomatic provided that the development of the plaques is slow, allowing sufficient time for a collateral circulation to be established, and no distal embolization occurs. In the case of ischemia, despite frequently similar clinical syndromes, the pathogenetic process and the pattern visible on the angiographic examination can differ, as detailed in the following section:
The extracranial ICA is patent. There is, however, occlusion of its intracranial branches, commonly due to embolic material arising from atheroma of the common carotid artery or, more frequently, from the ICA; alternatively, it can be cardiac in origin. In cases of TIA, the occlusion may be temporary owing to spontaneous thrombolysis, and it may no longer be recognizable on the angiogram. This is, however, an important warning signal, which should promptly lead to carotid and cardiac examination. Among the involved branches of ICA which will be discussed in the following chapters, there is the ophthalmic artery, the first branch of the intradural ICA. Its occlusion leads to “amaurosis” which is frequently, but unfortunately not always, transient (fugax).
Considering the atheromatous plaque, an important aspect is the degree of stenosis of the lumen involved. Here, four degrees of stenosis have been distinguished: mild, when the lumen is reduced by less than 30 %; moderate, with 30–70 % reduction of the lumen; severe, if the reduction is greater than 70 %; and preocclusion state, more than 90 %. The greater the stenosis, the higher is the risk of formation of a superimposed thrombus, which can be the source of the emboli. The stenosis can increase, reducing the flow with hypoperfusion and leading to ischemia in the distal vascular border territories or in the vascular territories of the perforators. An important role in the latter situation is played by the association of a severe contralateral ICA lesion and severe heart diseases (Bogousslavsky and Regli 1986; Bladin and Chambers 1993). The stenosis can be great, with considerable impairment of the blood flow, which is evident on the angiogram as a subocclusion pattern (Fig. 15.8).
To measure the stenosis on the angiogram, a few methods have been proposed; among them, the European Carotid Surgery Trial (ECST 1998) and North American Symptomatic Carotid Endarterectomy Trial (NASCET 1991) are the most used (Fig. 15.1). Differences in the applied criteria lead to differences in the results with these methods. There is broad agreement that a stenosis of 70 % with NASCET (corresponding to a stenosis of about 80 % with ECST) is a clear indication for endarterectomy, thereby reducing significantly the risk of stroke. Alternatively, endovascular treatment has increasingly been successfully performed (Wholey et al. 2000; Bonaldi 2002; Ringleb et al. 2006; Wittkugel et al. 2008; Stingele et al. 2008; Clark et al. 2010) (Figs. 15.2 and 15.3).
Fig. 15.1
Methods to measure ICA stenosis on the angiogram: European Carotid Surgery Trial (ECST) and North American Symptomatic Carotid Endarterectomy Trial (NASCET)
Fig. 15.2
Two examples of atherosclerotic plaque leading to severe stenosis of the ICA. (a) Carotid angiogram showing the stenosis (arrow) with slowing of the flow. (b) Angiogram after angioplasty and stent showing normalization of the lumen and flow. (c) Carotid angiogram showing the stenosis (arrow). (d) Angiogram after angioplasty and stent with normalization of the lumen and improvement in flow
Fig. 15.3
Very old female patient with asymptomatic moderate to severe stenosis of the left ICA (a). The ICA was an important collateral way through the circle of Willis for the right carotid sector since the right ICA was occluded. A preventive endovascular treatment with stent and angioplasty (b) was performed without any clinical problems
There are, however, some other aspects of the atheroma that should be considered. Ulceration, with the risk of distal embolization, can also occur in relatively small atheroma, and the progression and evolution of the atheroma may vary. Taking this into account, attention today has been progressively given to the aspects and components of the atheroma (presence of a thin or ruptured fibrous cap, a large lipid-rich core, intraplaque hemorrhage, calcifications). This information can be obtained today using many methods (ultrasound, CT, and especially MR) in various combinations to each other. The importance of examining the composition of the plaque is emphasized in the literature by the reports of many authors (Ouhlous et al. 2005; Cappendijk et al. 2005; Cai et al. 2005; Wasserman et al. 2008; Gao et al. 2009; Watanabe and Nagayama 2010; Kurosaki et al. 2011; Liu et al. 2012; Yoon et al. 2012). The characterization of the plaque will give information about the possible risk of stroke and influence, considering also other aspects especially the age of the patient and the presence of other comorbidities, the therapy whether it should be medical, surgical, or endovascular (Stingele et al. 2008; Clark et al. 2010; Rosenkranz and Gerloff 2010; Watanabe and Nagayama 2010; Yoon et al. 2012).
Atheroma can also occur in the intracranial segments of the ICA, especially in the distal petrous and cavernous portion. The clinical importance of these lesions has been revaluated, and now endovascular treatment may be undertaken in selective symptomatic patients (Fig. 15.4). Finally, stenosis of both the proximal and distal ICA (tandem lesions) is also reported, with a frequency of about 10 % of cases (Craig et al. 1982; Kappelle et al. 1999). This aspect should be considered when planning therapy.
Fig. 15.4
Carotid angiogram, AP view. Severe stenosis of the proximal intracavernous segment of the ICA (a), with intracranial flow impairment, leading to transient ischemic attacks (TIAs) not responsive to medical therapy. Endovascular treatment with angioplasty and stent; restoration of the intracranial circulation (b)
With regard to asymptomatic lesions, it should be noted that there is no general agreement about the therapy (medical or more aggressive) to be applied. The decision depends on the degree of the stenosis, aspects of the plaque, the presence or absence of a good collateral circulation, age, general clinical condition of the patient, and the experience of the medical team involved (Fig. 15.3).
The ICA is occluded, and the occlusion extends from the ICA bifurcation to its intracranial segment, proximal to the ophthalmic artery. In such cases, it can be assumed that the thrombus has extended from the ICA bifurcation to its intracranial terminal segment. Another possibility is that an embolus or a thrombus formed on a preexisting atheroma has occluded the intracranial ICA, followed by retrograde formation of a thrombus extending proximally toward the ICA bifurcation. In many of the cases, the angiogram shows a good collateral circulation through the ECA and circle of Willis, avoiding ischemia in the involved hemisphere. In cases of stroke, this is frequently due to occlusion of intracranial branches due to emboli arising from the occluding thrombus. These are sometime recognizable on the indirectly injected intracranial branches visible on the angiogram obtained from the contralateral ICA, VA, and ECA. In these cases, it is probably better to perform a conservative therapy instead to try to reopen the occluded artery with the risk of further embolization. This approach can be reconsidered when the collateral circulation is impaired due to anomalies of the circle of Willis or the presence of other lesions involving especially the other ICA.
A particular condition also called pseudo-occlusion (Seckar et al. 1980; Bradac and Oberson 1983) is that, in which, despite the proximal occlusion appearing on the angiogram, the distal part of the ICA is still patent which can be demonstrated on the angiogram in later phases. In such very rare cases, a rapid surgical or endovascular treatment can completely restore the lumen of the artery.
The occlusion is on the terminal ICA involving not only the distal supraclinoid segments (communicating and choroidal) but also the proximal segment of its terminal branches (A1 and M1) forming the so-called T occlusion. This is a very serious clinical condition since there is a lack of a collateral circulation through ECA and circle of Willis (Figs. 15.5a–d, 15.6, and 15.7). The only possible collateral circulation is through distal leptomeningeal anastomoses between ACA, PCA, and MCA. In this context, some further unfavorable conditions can occur: both ACA arise from the occluded ICA and the A1 segment of the normal contralateral ICA is hypo-aplastic and so no leptomeningeal collateral flow from ACA toward MCA is possible. The same occurs when the A1 segment is completely thrombosed up to the A1–A2 angle and so no collateral flow is possible from the normal A1 toward the A2 of the affected side through the AcomA. The PCA (fetal origin) arises from the occluded ICA and the P1 is hypo-aplastic. The collateral flow through leptomeningeal anastomoses from PCA toward ACA and MCA is impaired. This latter condition can also be unfavorable for a collateral circulation toward the AchA since in this case this artery cannot be retrograde injected by the way of anastomosis with branches of PCA especially through the posterior choroidal arteries.
Fig. 15.5
Drawing, AP view. Different findings in occlusion of the ICA proximal to the ophthalmic artery (a) and distal to it with involvement of communicating, choroidal, A1 and M1 segments (T occlusion) (b). In (a) a collateral circulation occurs through the ECA-ophthalmic artery anastomosis and through the circle of Willis provided that this is not impaired by hypo/aplasia of the A1. In (b), the above-described collateral ways are involved in the thrombotic process. The only possible collateral circulation is leptomeningeal anastomoses between distal branches of anterior cerebral (ACA) and posterior cerebral arteries (PCA) with middle cerebral artery (MCA). (c) Lateral view. Occlusion of the internal carotid artery (ICA) proximal to the ophthalmic artery (OA). Possible collateral circulation through OA and circle of Willis. External carotid artery (ECA); first segment of anterior cerebral artery(A1); first segment of middle cerebral artery (M1); posterior cerebral artery (PCA); posterior communicating artery (PcomA); basilar artery (BA) and P1 segment; and anterior choroidal artery (AchA). (d) Distal occlusion of ICA involving the communicating, choroidal, M1 and A1 segments. Possible collateral circulation only through leptomeningeal anastomoses (LMA). Possible anastomoses between posterior and anterior choroidal arteries
Fig. 15.6
Thromboembolic acute occlusion of the distal left ICA (T occlusion). Left carotid angiogram (a) showing stenosis of the ICA with the distal thrombus extending intracranially (arrows). There is collateral circulation neither through the ECA nor the circle of Willis. The stump of the proximally occluded ACA is recognizable in (c). There is, however, a rich leptomeningeal collateral circulation (arrows) through distal branches of the left anterior cerebral artery (ACA), visible on the right carotid angiogram (b, c, and d) and through branches of the PCA visible on the vertebral angiogram (e). Medical therapy was chosen, and the patient recovered with minimal neurological deficit
Fig. 15.7
Old patient presenting with aphasia, right hemiplegia and progressive cognitive disturbances. (a) Left carotid angiogram showing severe stenosis of the left ICA (arrow). There was no filling of the distal ICA and no collateral circulation through the ECA. (b) Right carotid and vertebral angiogram showing no collateral circulation through the circle of Willis, with the exception of a minimal injection of the left A1. (c) After stent application at the left ICA bifurcation, a microcatheter was advanced into the distal ICA, followed by mechanical thrombolysis, leading to reopening of the distal ICA and the collateral circulation through the circle of Willis (arrow). The proximal ICA remained occluded. (d) CT 24 h post treatment showing hemorrhagic ischemia of the basal ganglia and minimal cortical temporo-parietal ischemia. The patient recovered slowly over subsequent weeks. (e) Different patient, CT study performed 3 days after a successful complete recanalization of ICA T-occlusion. The hemiplegic patient recovered well. In spite of this excellent clinical result, small ischemic lesions in the nucleus caudatus, pallidum and putamen were recognizable
Fig. 15.8
Patient already treated with stent for stenosis of the right internal carotid artery (ICA). Acute mild neurological symptoms owing to impairment of the circulation of the left cerebral hemisphere. On the angiogram, subocclusion of the left ICA (a) due to a large atheroma with severe flow impairment. Collateral circulation from the right carotid artery (b). On MRI (c) (diffusion-weighted images), there were ischemic lesions in the vascular territory of the medullary arteries as a result of hypoperfusion. The small associated cortical lesions were probably embolic. Endarterectomy was successfully performed a few days later, with good clinical results
The cause of terminal ICA occlusion varies. It can be due to emboli that are cardiac in origin or from atheromatous plaque of the CCA, carotid bifurcation or proximal ICA. More rarely, this condition is due to atheroma involving the distal ICA leading progressively to occlusion of the vessel. In these cases, endovascular treatment performed increasingly with a mechanical thrombolysis allows in many of these patients the reopening of the artery improving their clinical outcome (Castano et al. 2010; Moehlenbruch et al. 2012; Machi et al. 2012). In some other cases, endovascular treatment makes it possible to reopen the distal ICA, while the extracranial segment remains occluded. This allows restoration of the collateral circulation through the circle of Willis (Fig. 15.7a–d). However, it should be noted that in all these cases, the risk of a large hemorrhagic transformation of the existing ischemia is high (Zeumer et al. 1993; Jansen et al. 1995; Ringer et al. 2001; Qureshi et al. 2001; Song et al. 2002; Flint et al. 2007; Malgorzata et al. 2008; Choi et al. 2009). Furthermore, it should be remarked that in ICA “T” occlusion, the perforators of ACA, MCA, AchA and distal ICA, which are end-arteries, are always involved. This explains the frequent presence of ischemic lesions in the basal ganglia, even in the most favourable cases with a rapid and complete recanalization (Fig. 15.7e).
In patients who have a known occluded ICA, ischemia can occur months or years later. In these cases, the etiopathogenesis may be hemodynamic or embolic. Indeed, emboli arising from atheromas in the stump of the ICA, in the common carotid artery or ECA, can reach the intracranial vessels via the collateral circulation through branches of the ECA, particularly those of the internal maxillary artery, which leads to a retrograde injection of the ophthalmic artery and ICA (Countee and Vijayanathan 1979; Countee et al. 1981; Bradac and Oberson 1983).
15.4.2 Middle Cerebral Artery
The MCA is the most frequently involved vessel in cerebral infarction. The pathogenesis is commonly artery-to-artery (carotid bifurcation) or cardiac embolism, which leads to occlusion of the M1 or of more distal branches (Figs. 15.9, 15.10, 15.11a–i, 18.2, and 18.3).
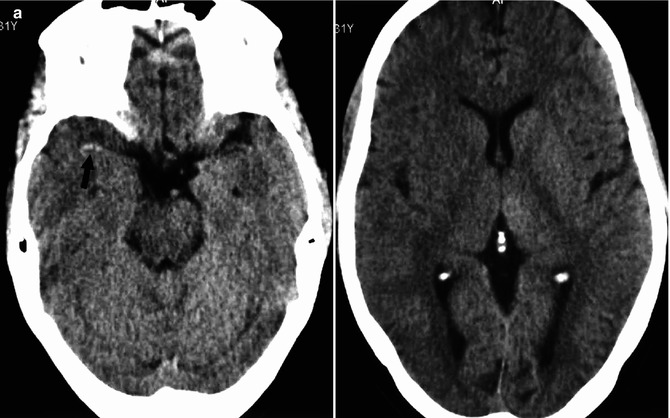
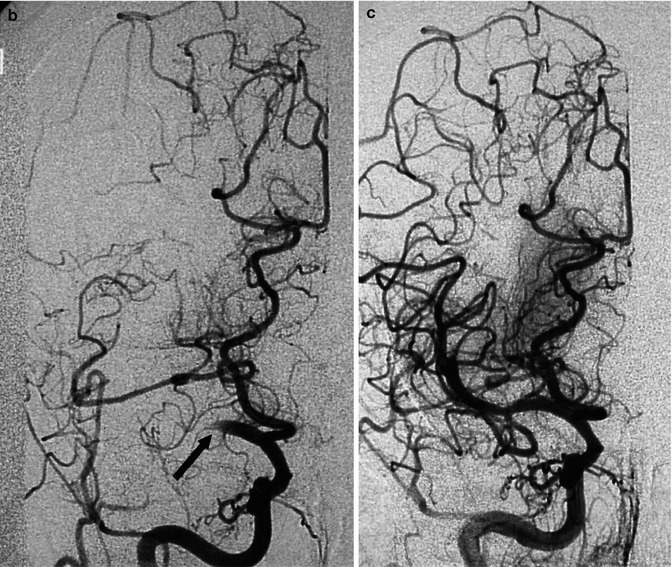
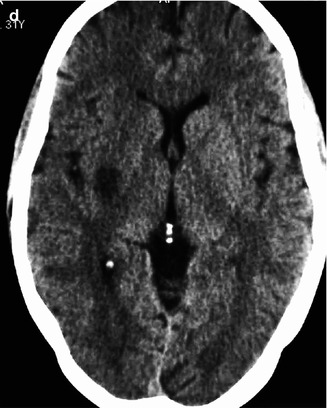
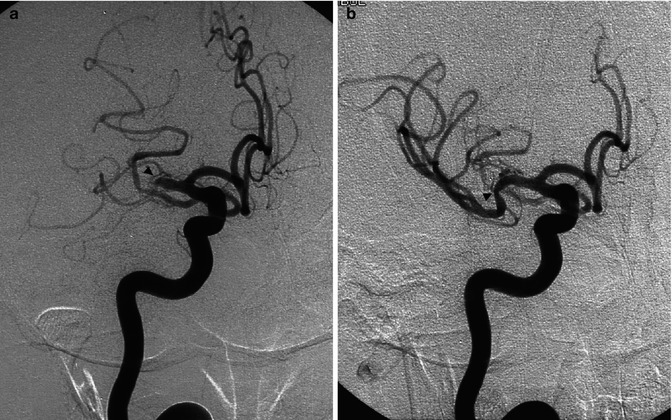
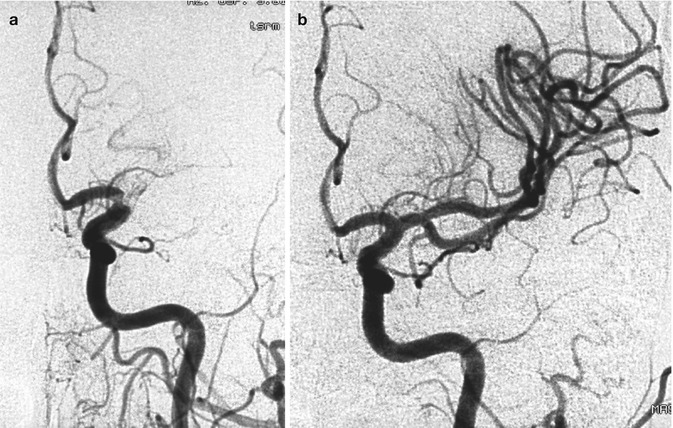
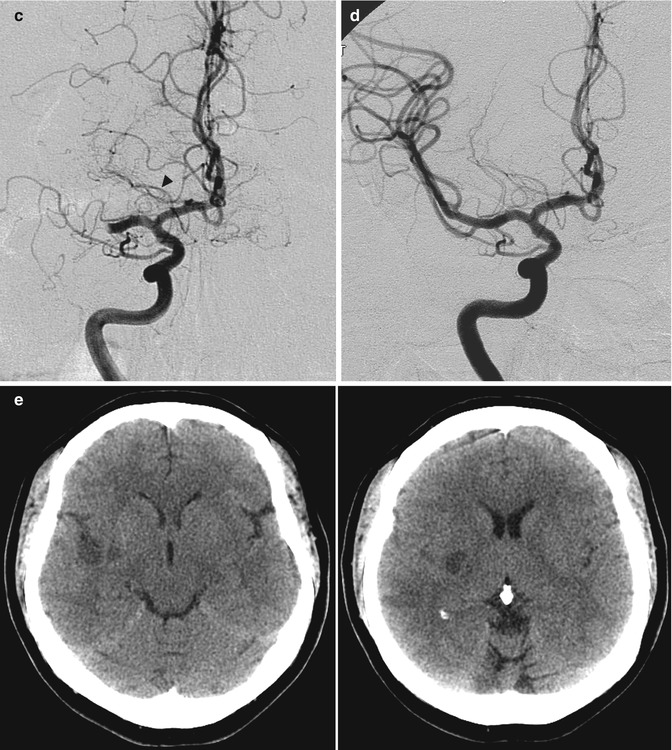
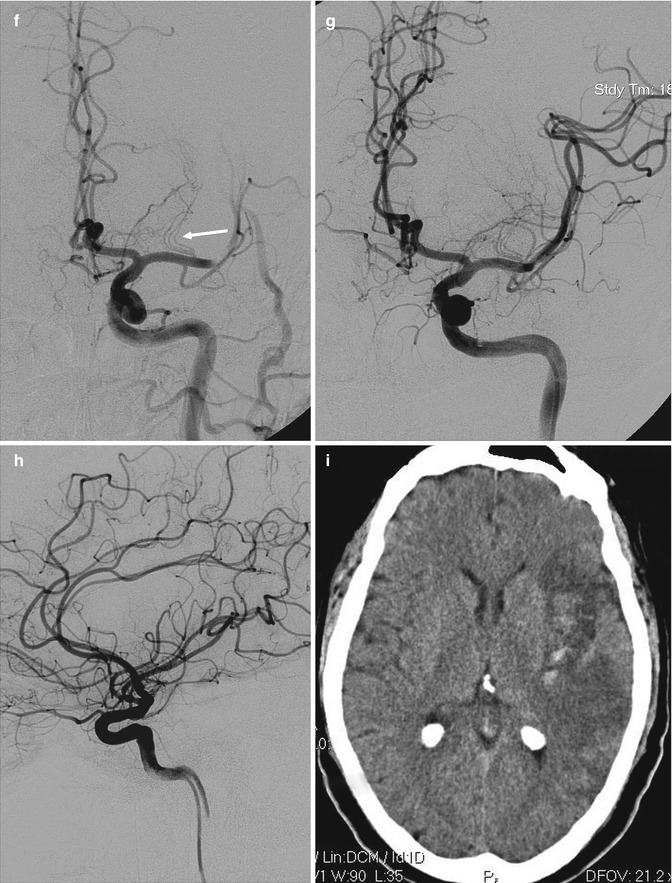
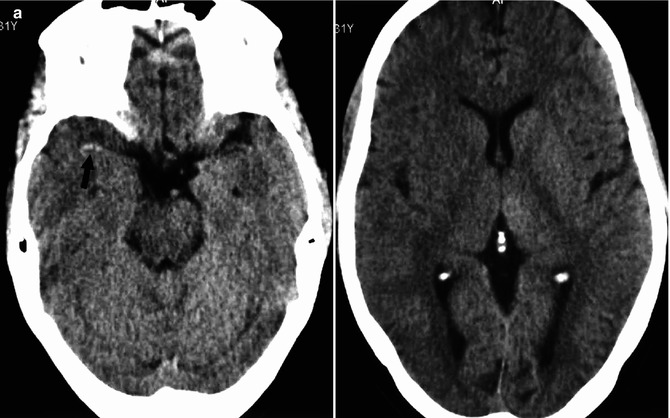
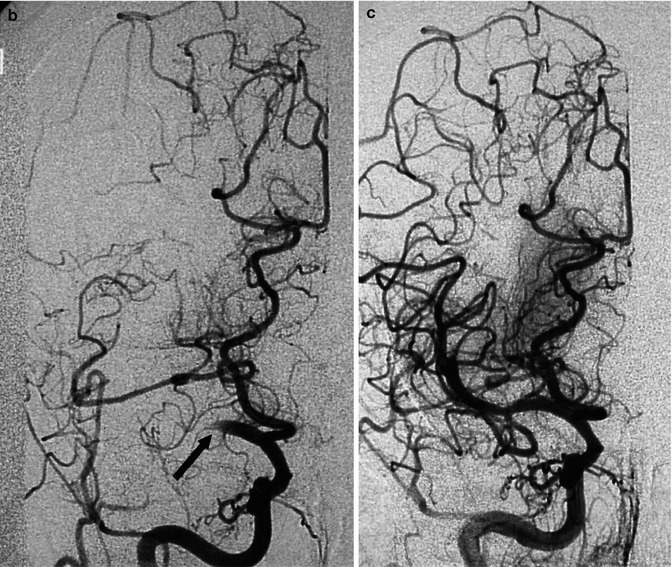
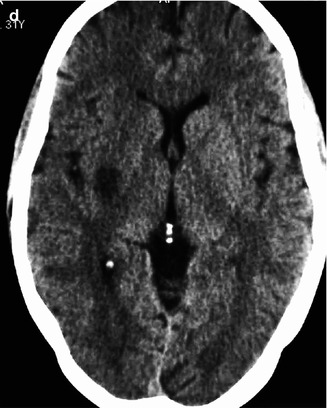
Fig. 15.9
Young cardiopathic patient with sudden onset of left hemiplegia. On CT (a), hyperdensity of the MCA (arrow) and ill-defined borders of the right basal ganglia are evident. Angiography (b) disclosed occlusion of the proximal segment of the M1 (arrow). Fetal origin of the PCA from ICA not involved by the occlusion. ACA arising from the left ICA. Partial collateral leptomeningeal circulation from the PCA. Selective pharmacological thrombolysis was performed, with reopening of the M1 (c) and complete clinical recovery. On CT (d) performed 3 days later, a small asymptomatic ischemic lesion in the vascular territory of the perforators was visible
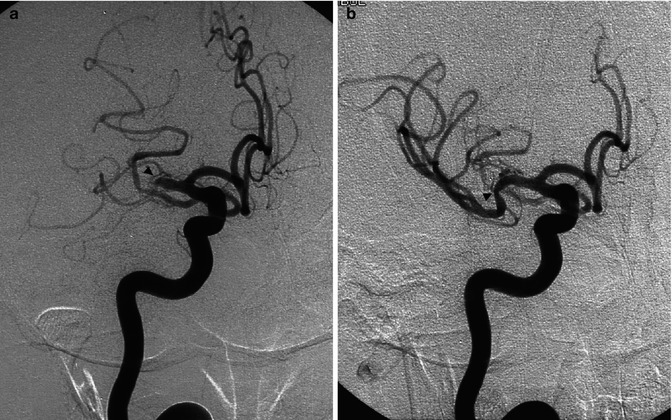
Fig. 15.10
Older woman with heart dysrhythmia presenting with sudden onset of hemiplegia with cognitive disorders. The right carotid angiogram (a) disclosed occlusion of the M1 segment (arrowhead). Endovascular treatment with reopening of the artery using a Solitaire stent was performed (b). The posttreatment angiogram showed the presence of microatheroma (arrowhead) associated with a tortuous distal M1, which probably favored the arrest of the embolus. A small infarct in the basal ganglia was visible on CT. In spite of this, the patient recovered completely. Note the presence of a fetal PCA not involved in the occlusion. Compare this Fig. with Fig. 18.3
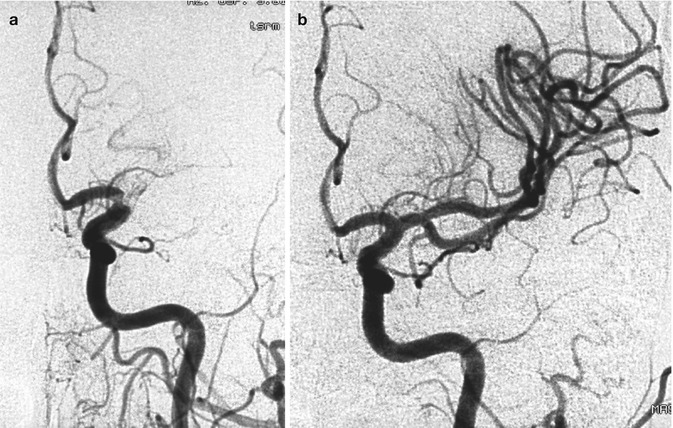
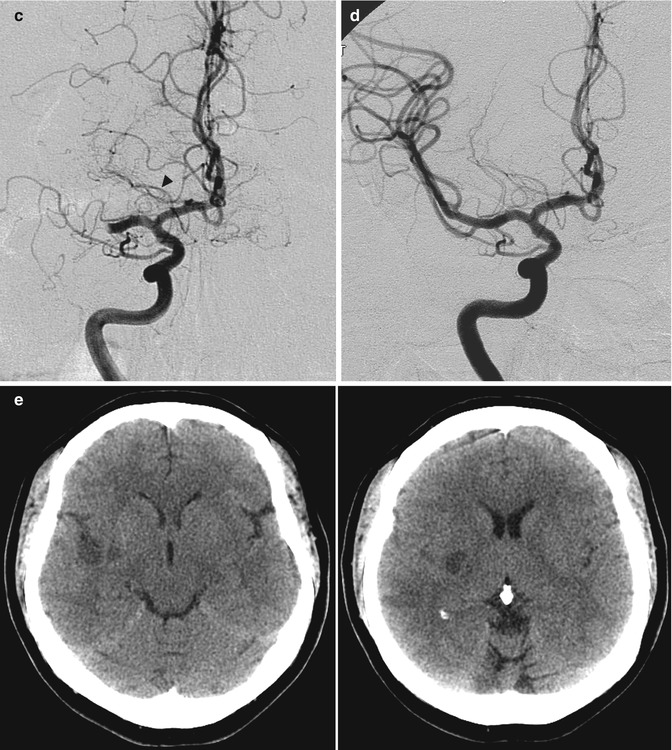
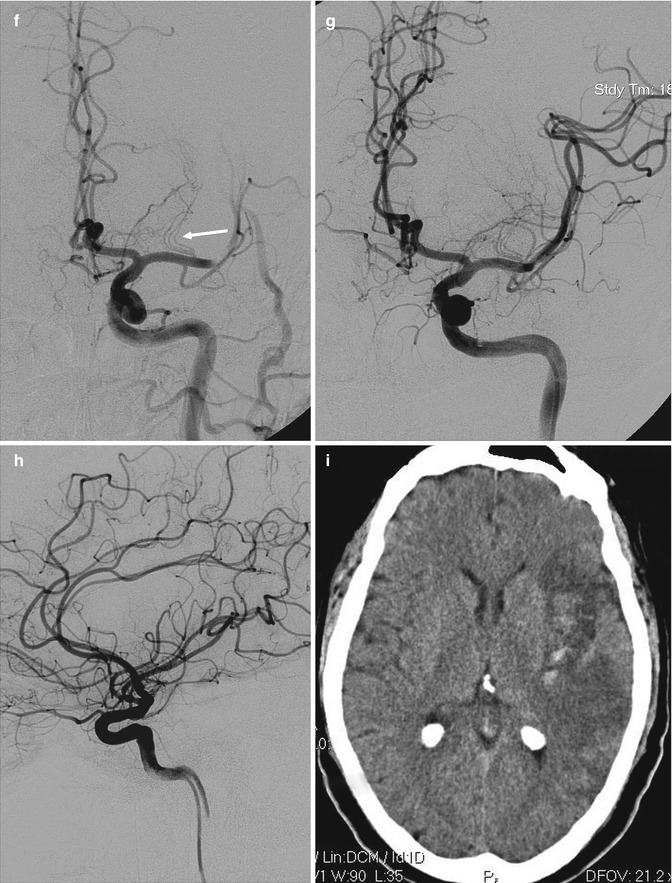
Fig. 15.11
(a, b) Young patient with acute occlusion of the M1 at its origin owing to cardiac embolism. Pharmacological selective thrombolysis (b) with complete reopening of the MCA and full clinical recovery. Small ischemic lesions in the basal ganglia were visible on CT performed some days later. There is an early bifurcation due to the very short M1 where the embolus was lodged. The perforators arise from the superior branch. (c–e) Probably embolic occlusion (middle-aged cardiopathic patient). Angiogram (c) showing the occlusion of proximal M1 with involvement of perforators. A temporal branch and a small artery directed to the insula are not involved. The AchA (arrowhead) is well recognizable as well as the perforators arising from A.C.A. The hemiplegic patient was rapidly treated with mechanical reopening (“Solitaire” device) of the artery. Angiogram post treatment (d). The patient recovered completely within a few days. The routinely performed CT (e) at this time showed small ischemic lesions in the lateral basal ganglia and also a minimal ischemia in the insula and temporal region. (f–i) Probably embolic occlusion (middle-aged diabetic and cardiopathic patient), presenting with acute aphasia and hemiplegia due to occlusion of MCA. Intravenous thrombolysis was performed without clinical improvement. The patient was transferred in the neuroradiology for further treatment. (f)The left carotid angiogram showed the occlusion at the passage between M1 and M2. The perforators (arrow) were not involved. (g) Mechanical reopening (Solitaire device) of the occlusion. (h) Some distal branches, as visible on the lateral angiogram, remained occluded, probably due to distal embolization from thrombus in the M1 or following endovascular treatment. (i) CT performed a few days later showed an insular ischemia with partial involvement of the temporal and parietal areas. Minimal hemorrhagic component. The basal ganglia were not involved. Partial clinical recovery in the following months
In some cases, after successful reopening of the vessel, it appears clear that the embolus has been arrested at the level of a stenosis of M1 due to a microatheroma (Fig. 15.10).
In occlusion of the M1, the perforators are always involved. The degree of involvement depends on the location of the embolus (in the proximal or more distal M1, morphological aspects of the M1, short or long, and the site of origin of the perforators) (see anatomy). Perforators are end arteries, and so their occlusion, even for a relatively short time, can lead to ischemia in their territories. This is confirmed in those cases where despite rapid reopening of the occluded M1 through the endovascular treatment, small ischemic lesions in the basal ganglia are frequently demonstrated in CT and MR (Bradac et al. 2008b) (Figs. 15.9a–d and 15.11c–e). Frequently, these patients remain asymptomatic or only minimal neurological deficit is present. This is a little surprising, since it is well known (De Long 2000) that the basal ganglia are involved in brain activity concerning movements, cognition, and behavior. A complementary functional MR study could be useful in the examination of these patients.
In other cases, in CT performed after successful endovascular reopening of the M1, small hemorrhages are recognizable in the basal ganglia following the hemorrhagic transformation of the ischemia in this area.
In proximal occlusion of MCA, the efficiency of the collateral circulation between branches of ACA, PCA, and distal branches of MCA plays an important role to protect the distal vascular territories. This, however, can be impaired by embolization involving the distal branches, which can be due to emboli arising from the thrombus lodged in the proximal MCA. Distal embolization can occur also during endovascular treatment with disruption or asportation of the thrombus lodged in the proximal MCA (Fig. 15.11f–i) Furthermore, distal branches can be selectively involved by small emboli, arising from ICA or hearth and passing through the proximal MCA (Figs. 18.2 and 18.3).
The therapeutic endovenous or selective intra-arterial pharmacological associated increasingly with mechanical thrombolysis using different devices has improved in many cases the prognosis of these patients (Arnold et al. 2002; Qureshi et al. 2001, 2002; Noser et al. 2005; Zaidat et al. 2005; Tauntopoulou et al. 2008; Eckert 2009; Choi et al. 2009; Roth et al. 2010; Moehlenbruch et al. 2012; Machi et al. 2012).
Microatheroma of the M1 (Caplan 1989; Bogousslavsky et al. 1991) is another less common cause of ischemia which directly involves the perforators. Distal cortical and white matter vascular territories can also be involved in these cases by either hypoperfusion or distal embolization. Stenosis of the M1, as with stenosis of other intracranial arteries, particularly of the terminal ICA and basilar artery when symptomatic and not responsive to medical therapy, is reported to have a bad prognosis (Thijs and Albers 2000; Wasid 2003). Endovascular treatment (angioplasty, stent) has opened new ways of therapy (Berkefeld et al. 2003; Gupta et al. 2003; Bose et al. 2007; Turk et al. 2008; Bradac et al. 2008b; Qureschi et al. 2008; Berkefeld and Zanella 2009; Miao et al. 2011; Dorn et al. 2012a) (Figs. 15.12 and 15.13) and produced promising results. The rate of complications with this treatment, however, is still relatively high. Hemorrhage due to vessel rupture or reperfusion, and new ischemic lesions can occur. The latter are commonly the result of occlusion of the perforating branches from the stent struts crossing their origin or displacement of microatheromatous material. Fortunately, and somewhat surprisingly, this is relatively seldom. Taking these considerations into account, the treatment should be performed only in selected patients in whom medical therapy has failed, especially when the stenosis is severe and not in the acute phase after stroke (Chaturvedi and Caplan 2003; Kurre et al. 2010). Another unsolved problem is the in “stent restenosis or thrombosis” which still occurs relatively frequently (Kurre et al. 2010). This negative evolution involves all treated intracranial stenosis, but it is reported to be more frequent in the anterior circulation and in this group in those of the intradural internal carotid artery especially in younger patients (Levy et al. 2007; Turk et al. 2008). Why this occurs is not known. It has been suggested that the intradural ICA stenosis in young patients represents a distinct pathology not linked to atherosclerosis but to an inflammatory disease (Levy et al. 2007; Turk et al. 2008) which reacts differently to the endovascular treatment.
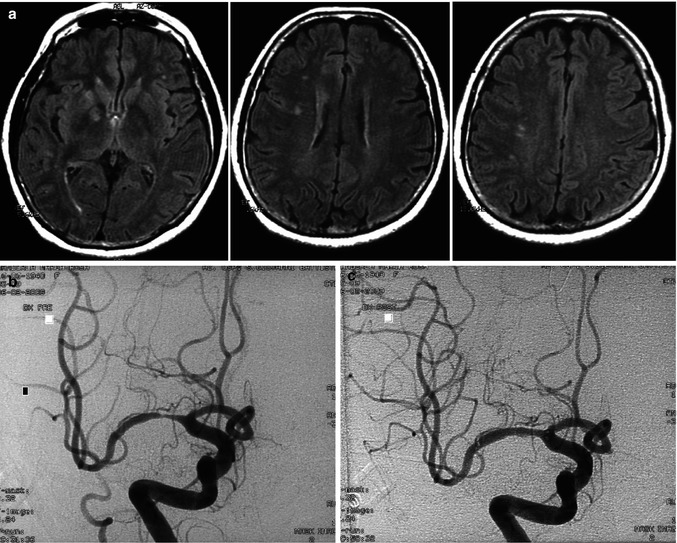
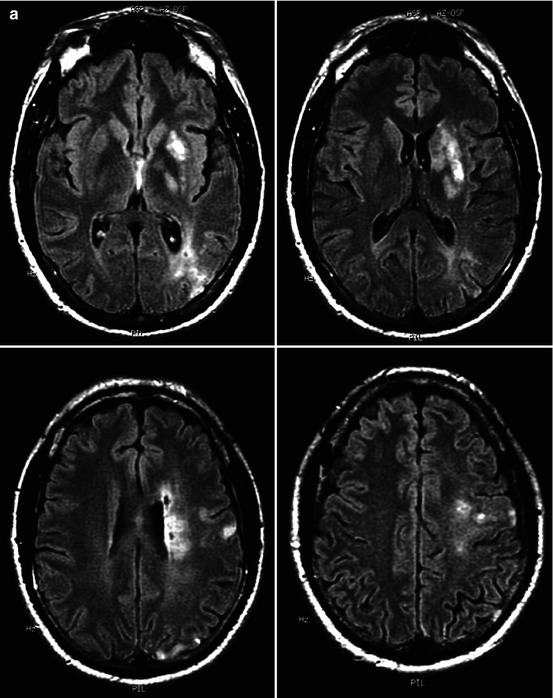
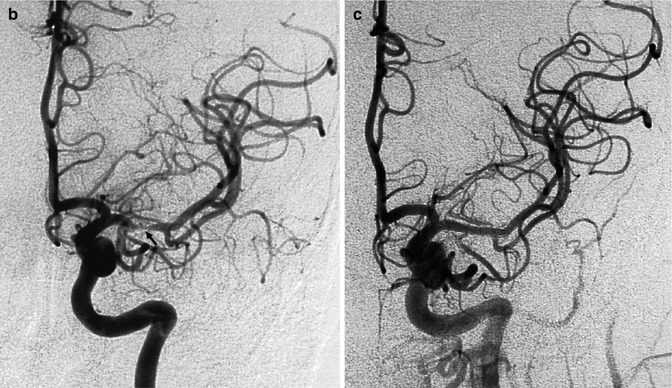
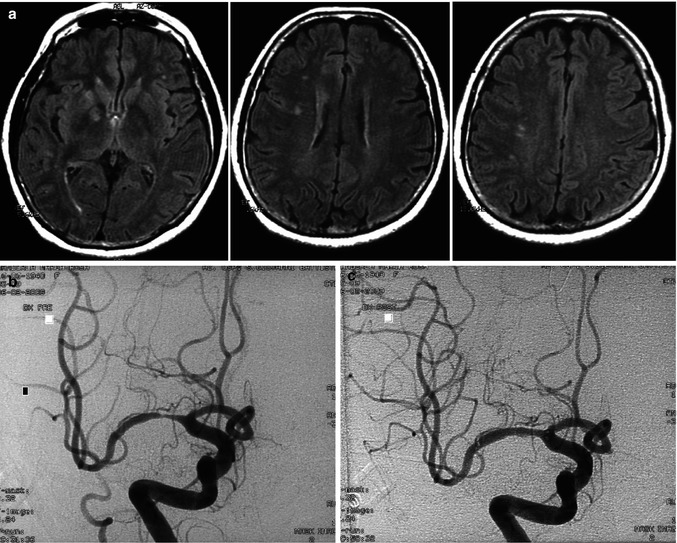
Fig. 15.12
Middle-aged patient with mild neurological deficit indicating impairment of the right ICA vascular territory. MRI (a) showed an ischemic lesion in the right basal ganglia and white matter. A few lesions were also present on the left. Angiogram (b) disclosed severe stenosis of the M1 with normal distal perforators. Endovascular treatment (angioplasty and stent) was performed (c). It is conceivable that the proximal perforators were occluded by the microatheroma while the distal perforator and medullary artery were involved by hypoperfusion
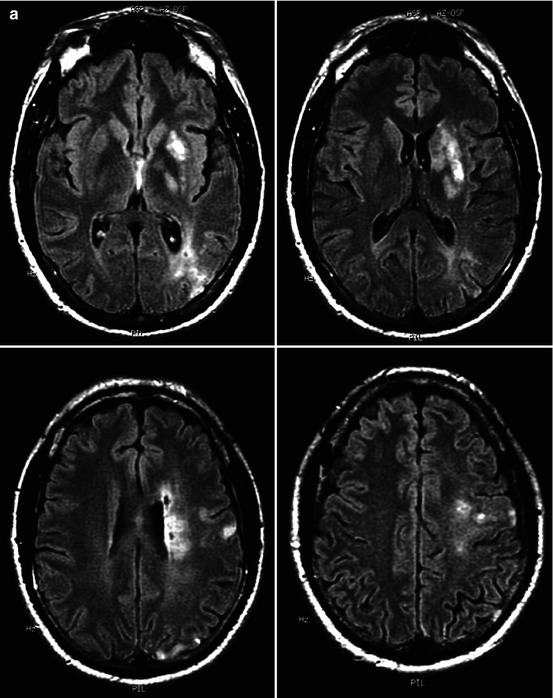
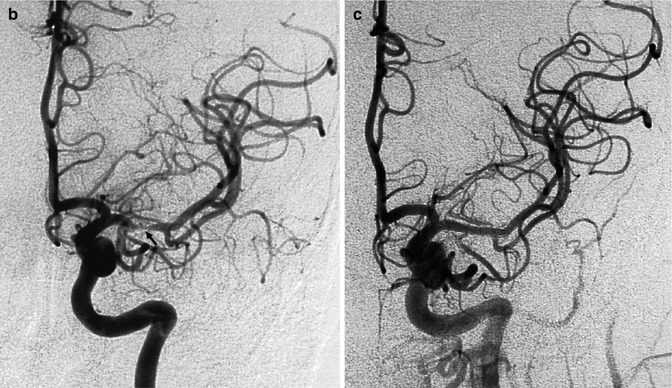
Fig. 15.13
Acute ischemia in a middle-aged patient involving the left basal ganglia, white matter, and cortex as demonstrated on MRI (a). Angiography (b) disclosed severe stenosis of the M1 (arrow). Some proximal perforators are occluded at their origin. Ischemia in the basal ganglia was due partially to direct occlusion of the proximal perforators at their origin and hypotension in the more distal branches. Ischemia in the white matter and in the temporo-occipital region was probably due to hypoperfusion, involving the medullary and distal cortical branches, respectively. Small ischemic cortical lesions in the frontoparietal area were embolic. Three weeks later, endovascular treatment (angioplasty plus stent) was performed (c). The patient recovered completely
15.4.3 Anterior Choroidal Artery
Infarcts in the territory of the anterior choroidal artery (AchA) are frequently associated with ischemia in other vascular territories, especially the MCA and PCA. The pathogenesis is carotid or cardiac embolism, or atheroma in the terminal ICA (Helgason et al. 1986; Boiten and Lodder 1991; Hupperts et al. 1994; Levy et al. 1995). Involvement of the AchA in T Occlusion has already been described (Sect. 15.4.1). Finally, also isolated embolization (Fig. 15.14), as well of cases of ischemia due to an inadvertent occlusion of the artery (Friedman et al. 2001) in surgical or endovascular treatment of aneurysm in this area, can occur. As already described, the vascular territories of the AchA can be replaced by other arteries. Furthermore, there are many anastomoses with branches of the PCA, and this explains the irregular presence of ischemic lesions in cases of occlusion of the AchA.
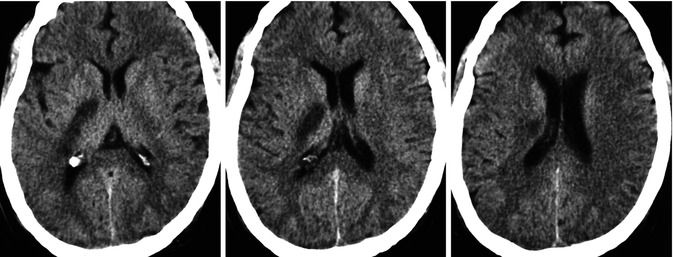
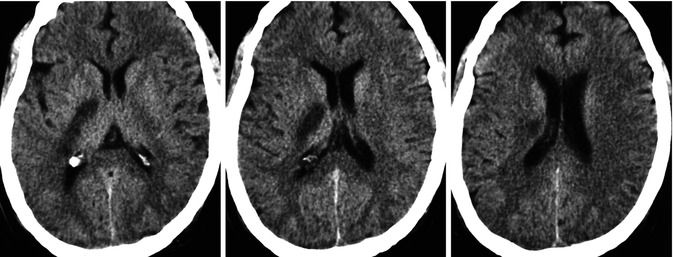
Fig. 15.14
Middle-aged patient with cardiac dysrhythmia presenting with stroke due to occlusion, probably embolic, of the anterior choroidal artery. CT showing ischemia in the corresponding vascular territory
15.4.4 Anterior Cerebral Artery
Infarcts in the territory of the ACA are commonly associated with ischemia in other vascular territories after carotid or cardiac embolism (Figs. 15.15 and 18.2). Embolization is not infrequently associated with variants in the anterior portion of the circle of Willis, which favors the passage in the ACA (Kazui et al. 1993). The vascular territories of perforators and/or peripheral branches in varying combinations can be involved depending on the proximal or more distal location of the occlusion.
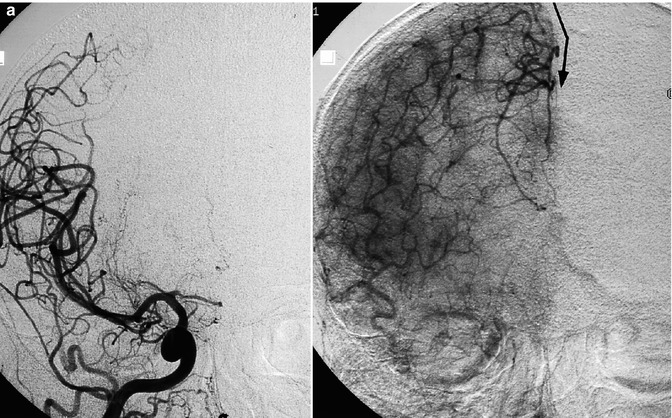
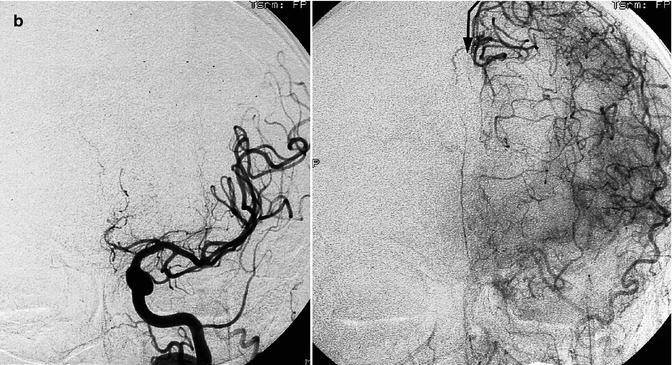
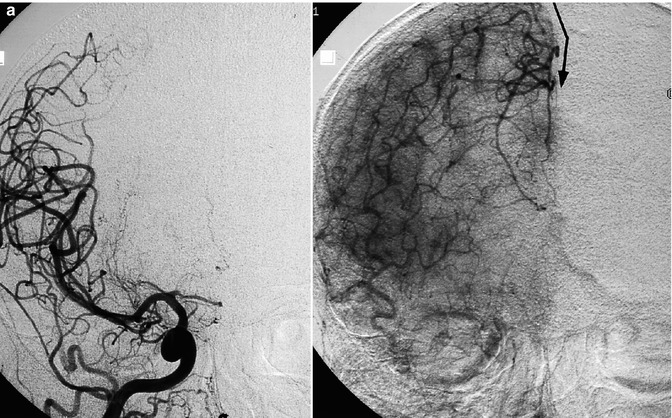
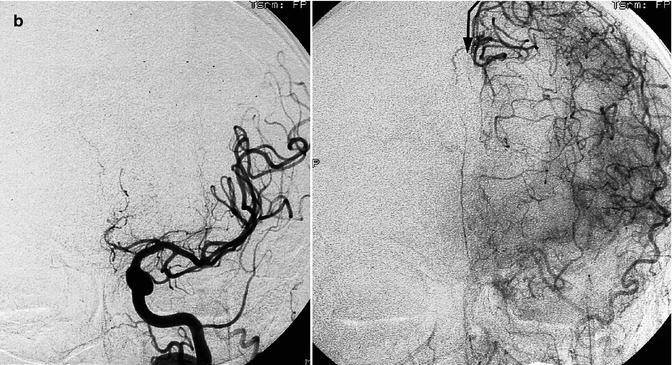
Fig. 15.15
Old female patient with stroke due to bilateral occlusion of the ACA. (a) Right carotid angiogram showing the occlusion at the passage of the A1–A2 segments. In the late phase, the beginning collateral circulation (arrow) through opening of anastomoses with distal branches of the MCA. (b) Left carotid angiogram showing a similar finding
Considering especially the artery of Heubner, its involvement, also in proximal occlusion, can vary due to its possible different site of origin (see also Chap. 4). Also, the inadvertent occlusion of the artery of Heubner in treating aneurysm of the ACA can lead to ischemia.
Microatheroma typically located in the pericallosal segment can be another rare cause of ischemia (Fig. 15.16).
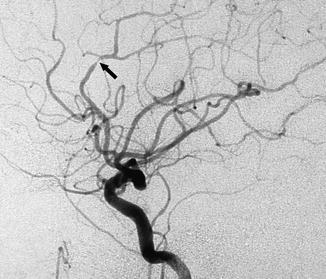
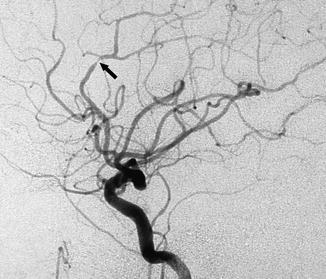
Fig. 15.16
Typical location of a microatheroma leading to stenosis of the pericallosal artery (arrow) in an asymptomatic patient
15.4.5 Lacunar Infarcts in the Anterior Circulation
These infarcts are due to involvement of the deep perforators supplying the basal ganglia and capsula interna and perforators supplying the superficial and deep white matter, which are called medullary arteries. As already described, these arteries do not anastomose to each other, and neither are connections between the deep perforators and medullary arteries present (De Reuck 1972; Moody et al. 1988, 1990, 1991). Both vascular territories or only one can be involved. This pathological condition, also called small vessel disease, presents with clinical symptoms which depend on the site and extension of the damaged parenchyma. Isolated lesions can be asymptomatic. Strokes can occur followed by improvement. Multiple lesions can develop presenting with or without strokes leading to a progressive decline of different neurological function with cognitive impairment and finally to dementia.
A frequent pathogenesis is a special form of atherosclerosis occurring particularly, but not always, in patients with hypertension and diabetes called lipohyalinosis, characterized by fibrinoid changes followed by collagenous fibrosis. This leads to stenosis or occlusion of the artery causing ischemia and sometimes to rupture of the wall and hemorrhage. The lesions can be very numerous and disseminated in both hemispheres. MR demonstrates on T2-weighted images the hyperintense ischemic lesions not rarely associated with small hypointense foci due to old microhemorrhage well defined with T2*-weighted gradient-echo and SW-weighted images. Pathological examinations disclose infarcts, commonly small (less than 20 mm in diameter) in the basal ganglia and white matter. In this latter, also areas of rarefaction due to destruction of fibers and area of gliosis have been demonstrated (Pantoni et al. 1996). No pathological changes of the arteries can be detected on the angiogram in many of these patients.
Another cause of ischemia is severe stenosis or occlusion of the ICA leading to hypoperfusion (Weiller et al. 1991; Waterston et al. 1990; Bogousslavsky et al. 1991; Boiten and Lodder 1991; Bogousslavsky and Regli 1992; Bladin and Chambers 1993; Horowitz and Tuhrim 1997; Boiten et al. 1997; Read et al. 1998; Bradac et al. 2008b). In this condition, the vascular territory of the medullary arteries is commonly more affected than that of the deep perforators (Fig. 15.8).
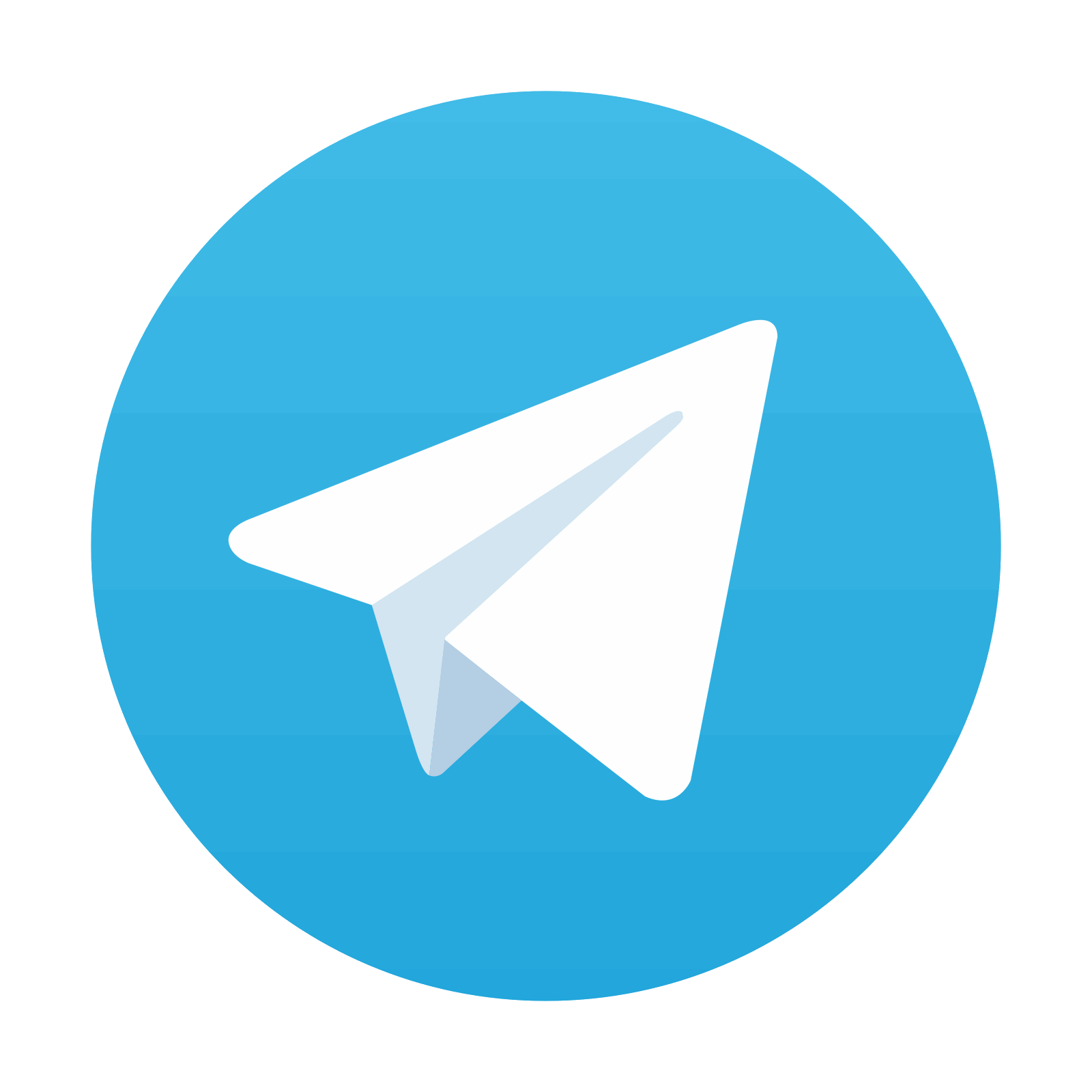
Stay updated, free articles. Join our Telegram channel

Full access? Get Clinical Tree
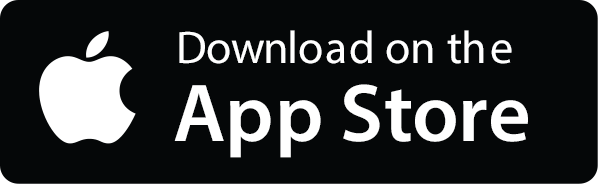
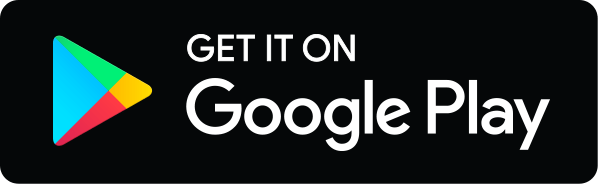