Myocardial ischemia is defined as the sudden decrease or even stop of the flow of oxygenated blood that in normal conditions perfuse the myocardial tissue. The presence of myocardial ischemia initiates a cascade of events (Nesto and Kowaldruk 1987), as summarized in Figure 13.1, that ends with the appearance of symptoms—angina or the equivalent—and often evolves into myocardial infarction (MI). Recently, however, Kenigsberg et al. (2007) have demonstrated that delay of repolarization (QT lengthening and peaked and symmetric T wave) appears after a few seconds of coronary occlusion. This probably means that the electrical disturbances may be present at the same time or even before mechanical alterations. Frequently, arrhythmias appear and these may even lead to sudden death. The ECG alterations appear as a consequence of the electrophysiological and pathological changes that transient or long‐lasting ischemia induces in the myocardial cells. Here, we comment on the results obtained using different methodologies to occlude coronary arteries, and experiments induced by cooling (equivalent to ischemia) and warming different zones of the left ventricle wall. We consider that these experiments are very helpful in clarifying and understanding the mechanisms that explain ECG changes in human beings. In the 1940s, Bailey et al. (1944) conducted open chest experiments in dogs in which the left anterior descending (LAD) coronary artery was occluded, with the electrodes located in the pericardial sac. It was found (Figure 13.2A) that the first ECG change recorded in the area with induced ischemia was a negativity of the T wave. This was called an ECG pattern of ischemia. If the ischemia persisted, it was followed by an ST elevation that was called an ECG pattern of injury. Finally, a Q wave of necrosis appeared, which Bayley called an ECG pattern of necrosis (see Figure 13.2A). This terminology was accepted by several groups (Sodi and Calder 1956; Chou et al. 1974) and used in clinical practice. Therefore, it was considered that in patients with ischemic heart disease (IHD), the presence of a negative T wave represented a grade of acute ischemia that was less severe and/or persistent than an ST segment elevation. Later on, Lengyel et al. (1957) found that when the experiment to occlude the artery was performed in awake dogs with a closed chest, and the ECG was recorded with a 12‐lead surface ECG, the changes in the ECG (Figure 13.2B) were similar to those that occurred in coronary spasm or transmural MI in humans. This means that the negative T wave only appeared as a late manifestation, when the acute ischemia was already vanishing or had already disappeared. In Chapter 20, we will comment that clinically negative T wave never appears during the acute phase of ischemia (pain). It may be seen during the evolving phase of STEMI if the artery opens, and in the evolution of Q‐wave MI, as a deep negative T wave, or in some phases of NSTEMI as a mild negative T wave (less than 2 mm). Various studies (Khuri et al. 1975; Franz 1983) have recorded unipolar ECG and transmembrane action potentials (APs) during regional ischemia produced by temporary coronary occlusion in open chest dogs, both in subepicardial and in subendocardial zone (with the deepest intramyocardial electrode), and also in the vicinity of the ischemic area. In all ischemic areas, the AP shows a progressive decrease of its rate of rise and its amplitude (low‐quality AP). However, the TAP remains normal outside the cyanotic border (the ischemic area). The unipolar ECG taken in various ischemic areas records successively the positivity of the T wave followed by ST elevation and the appearance of Q with a negative T wave, but the AP changes record the local electrophysiological changes more precisely, which demonstrates that they express the changes induced by ischemia better than unipolar ECG recordings (Franz 1983). Figure 13.1 Ischemic cascade. The figure shows the sequence of events in asymptomatic period before angina starts (Modified from Nesto and Kowaldruk 1987) (see text). Figure 13.2 (A) Recordings in the case of experimental occlusion of left anterior descending (LAD) coronary artery in a dog with open heart. (1) Control. (2) ECG pattern of ischemia (negative T wave). (3) ECG pattern of injury (ST segment elevation). (4) Appearance of ECG pattern of necrosis (Q wave) (Based on Bailey et al. 1944). (B) Electrocardiographic–pathological correlations after the occlusion of a coronary artery in an experimental animal with its thorax closed. (1) Control. (2) It changes from a subendocardial ischemia pattern (tall and peaked T wave) to a pattern of an ST segment elevation when the acute clinical ischemia is more severe and transmural (3). Finally (4), the “q” wave of necrosis develops, accompanied as time passes by an increasingly evident pattern of negative T wave (see text) (Based on Lengyel et al. 1957). On the other hand, in experimental models or anesthetized dogs with the heart exposed through a sternetomy and with electrodes pulled over its surface, Burnes et al. (2001) induced a delay of repolarization of the AP of the epicardial zone submitted to cooling that overcame the duration of the AP of mid‐endocardium. This explains that the T wave becomes negative (see Figure 13.3A‐3). In this model, warming the same subepicardial zone produces a shortening of AP of this zone and a more positive and peaked T wave arises (Figure 13.3A‐2). The same difference in the duration of AP of the subepicardium (shorter), and mid‐subendocardium (longer), could theoretically also be obtained by cooling the subendocardial area. Thus, the longer AP of mid‐subendocardium may explain the wider and peaked T wave observed at the onset of subendocardial ischemia. In the experiment, the evaluation of 12‐lead ECGs during cooling and warming shows changes similar to those seen in epicardial electrograms (see Figure 13.3B and C). We will now summarize the correlation of these different types of ischemia or similar damage with electrophysiological and surface ECG changes. According to the importance and duration of ischemia or similar damage (cooling the area or the effect of some toxins), experimental studies (Coksey et al. 1960; Horan et al. 1971; Burnes et al. 2001) have demonstrated that the APs of myocardial cells present changes in both repolarization and depolarization: The classical ECG changes due to ischemia are the following: Figure 13.3 (A) Transmembrane action potential (AP): control (1) and after warming (2) and cooling (3) the epicardium. It is seen that after cooling (3), equivalent to ischemia, there is a delay of AP of subepicardium that explains the negativity of the T wave. After cooling the mid‐endocardium area, there is probably a delay of AP of this area that explains the existence of a voltage gradient similar to what would exist in the presence of warming the epicardium (2), which explains the appearance of a taller and wider T wave with longer QT interval. B and C show the ECG changes in surface ECG after cooling (B) (negative T wave) and warming (C) (positive T wave) the epicardium (1, 2, and 3 surface ECG leads) (Reproduced with permission from Burnes et al. 2001.) Figure 13.4 The different morphological features that appear after coronary occlusion (Reproduced with permission from Fallon 1996). These include the Q wave of necrosis and reciprocal patterns, and changes in the mid‐late part of QRS (fractionated QRS). Other changes include lengthening of QT interval (this is, in fact, the first change according to Kenigsberg et al. (2007)), QT dispersion, changes of P wave, increase in duration and distortion of QRS, active and passive arrhythmias, and sudden death (see Chapter 20 and Fiol‐Sala et al. 2020). The occlusion of a coronary artery leading to MI represents a pathological process that may be divided into four phases (Fallon 1996): (i) ischemic insult, (ii) coagulation necrosis, (iii) healing, and (iv) scarring. Figure 13.4 shows the most important morphological features of each phase. ECG changes appear very early and last throughout this process. This includes ST/T changes and the appearance of Q of necrosis. Figure 13.5 shows the sequence of appearance of these ECG patterns. First the peaked, symmetric, and usually taller T wave and after the shifts of ST (ST/T changes) corresponds to the phase of ischemic insult, and the appearance of pathologic Q waves with negative T waves indicates the phase of necrosis. The evolution of Q wave–MI corresponds to phases 3 and 4 of Fallon’s classification. Figure 13.5 Observe how different degrees of ischemia that appear sequentially after total coronary occlusion in a heart without previous ischemia (A) explain the ECG morphologies. (B) Ischemia predominant in the subendocardial area (T wave symmetric and usually taller than normal with longer QT interval). (C) In the presence of more severe ischemia evolving to transmural injury, ST segment elevation is present. (D) If the ischemia persists, transmural necrosis expressed as Q wave of necrosis and a negative T wave appears. We will comment throughout this chapter and in Chapter 20 on the correlations between the ECG changes, the site of the occluded artery, the area at risk (ST/T changes), and the final necrotic area (Q wave or equivalent). We will always have in mind the importance of the direct and mirror patterns (see Figures 13.13 and 13.35–13.39). All ECG changes induced by ischemia (T wave, ST segment, and Q wave) in the left ventricle are located in the four walls of the left ventricle that are in agreement with the statement of the Imaging Societies of the US (Cerqueira et al. 2002), and the statement of the International Society for Holter and Noninvasive Electrocardiology (ISHNE) (Bayés de Luna et al. 2006a; Fiol‐Sala et al. 2020), are named: anterior, septal, inferior, and lateral (see Chapter 3). The old posterior wall coincides with the inferobasal segment of the inferior wall (Thygesen et al. 2019). Furthermore, this segment rarely bends upward to become a true posterior position. Usually it remains flat and is the posterior part of the inferior wall but not a true posterior wall (see Figures 4.1–4.6). In this chapter, we will focus on the changes in T wave, ST segment, and QRS complex that are induced by experimental and clinical ischemia and their electrophysiologic mechanism in the presence of narrow and wide QRS complexes. Later, in Chapter 20, the ECG changes that appear during the evolution of different clinical settings of IHD, their diagnostic criteria, and the most important clinical and prognostic implications of QRS–ST–T ECG changes and other changes already mentioned will be discussed. The electrophysiologic explanation of the changes in the T wave caused by experimental ischemia or similar damage (cooling the area) that produces delay of repolarization in the affected zone (prolonged action potential ‐AP‐), without change in the shape of it may be explained by two theories (Sodi and Calder 1956; Cabrera 1958; Coksey et al. 1960; Franz 1983; Burnes et al. 2001; Fiol‐Sala et al. 2020). The first theory is based on AP summation. The same reasoning has also been used to explain the appearance of a normal ECG (see Chapter 5). Figure 13.6 shows how the sum of the APs of the subendocardium and subepicardium, when the delay of repolarization due to ischemia is in the subendocardium or in the subepicardium, respectively, may explain the appearance of a taller or wider T wave (ECG pattern named subendocardial ischemia) (Figure 13.6B) or a flat or negative T wave (ECG pattern named subepicardial ischemia) (Figure 13.6C,D). Both cases are caused by delay of repolarization in the affected area and present with a lengthening of the QT interval. The second theory is the theory of the ischemic vector. According to this theory, the area with delayed TAP (named the ischemic area), either in the subendocardium (Figure 13.7B) or in the subepicardium (Figure 13.7A), was not fully repolarized at the time when the other area was already repolarized. Therefore, it still presents with negative changes. As repolarization starts in the zone that is less ischemic, the sense of repolarization ( Figure 13.6 Explanation of how the sum of the transmembrane action potentials (APs) from the subepicardium and the subendocardium explain the ECG, both in the normal situation (A), in the case of experimental subendocardial ischemia (B) (tall and peaked T wave), and in mild (C) and severe (D) experimental subepicardial ischemia (flattened or negative T waves). This is the consequence of the repolarization delay in ischemic areas and the more prolonged AP. Clinically, ischemia is never exclusively subepicardial. Negative T wave related to ischemia may be seen in post Q‐wave MI (E), and also when the surface ECG records the gradient of voltage between the transmural involved area probably due to edema (Based on Migliore et al. 2011) (longer AP) and the rest of the left ventricular wall (F) (see text, Figure 13.7, and Chapter 20). The normal T wave (see Chapter 7) is asymmetric and is positive in all leads apart from aVR and often V1, and on occasion in III, aVF, and rarely II and even aVL in the case of vertical heart with rS or QS, and usually negative P wave. The normal negative T wave is of low voltage (except aVR) and asymmetric. Therefore, the appearance of a flattened or negative and symmetric T wave in other leads (V2–V6, I, II, and in general V2 and aVL) is abnormal, and also is a suspicious, positive, tall, and symmetric T wave, and its presence in V1 is also abnormal. Table 13.1 shows the current ECG criteria for T changes due to ischemia. However, in our opinion, T inversion usually is a sign of reperfusion and therefore is not due to acute ischemia (post‐ischemic change) (Birnbaum et al. 2020a, 2020b) (see later) and Chapter 20—ECG in NSTEMI). As a consequence of occlusion, the first effect is subendocardial involvement in the left ventricle (Figure 13.5B). As a result of the delay of repolarization in this area (prolonged AP without change of its shape) (Figure 13.6B and 13.7B), a peaked, wide, and usually tall T wave appears, accompanied by QT lengthening (T wave of subendocardial ischemia). It has been suggested that this tall T wave also reflects a release of K (Katz 2001). In fact, the subendocardium is more vulnerable to ischemic damage than the subepicardium. Different factors explain the susceptibility of the subendocardium to the development of ischemia. These include the characteristics of subendocardial coronary flow, the greater dependence of this region on diastolic perfusion, and the greater degree of shortening and therefore of energy expenditure of this area during systole (Bell and Fox 1974). Epicardial stenosis is associated with reduction in the subendocardial to subepicardial flow ratio. This first prolongs the AP of the zone (positive T wave) and if the reduction is greater, the AP changes its shape (ST depression) (Dunker et al. 1998; Figure 13.8). Thus, in the subendocardium after epicardial coronary occlusion, the wave front of ischemia starts (Reimer et al. 1981). If more important subendocardial ischemia exists, the AP of the area will present not only longer duration but also smaller area. The sum of two AP of the subendocardium and the subepicardium (Figure 13.5C) explains the ECG pattern with mild/moderate ST depression and tall T wave that is seen sometimes in the acute phase of STEMI due to LAD subocclusion (De Winter et al. 2008) (Figure 20.7). Also, it has been demonstrated with CV magnetic resonance (CE‐CMR) (Marholdt et al. 2005) that the first area where appears a wave front of necrosis starts in the subendocardium (Figure 13.9). Figure 13.7 (A) Subepicardial experimental ischemia. Subendocardial repolarization is complete, but the transmembrane action potential (AP) in the subepicardium still shows negative charges and is longer than normal (AP prolongation further beyond the dotted line) because the subepicardium is not completely repolarized yet. Thus, the vector of ischemia that is generated between the already polarized area in the subendocardium with positive charges and the subepicardial area still with an incomplete repolarization (with negative charges) due to the ischemia in that area, is directed from the subepicardium to the subendocardium, with the head of the vector coinciding with the positive charge of the dipole of repolarization. Therefore, the subendocardium is faced with the vector head (positive charge of the dipole), which explains why the T wave is negative in the subepicardium. (B) In subendocardial ischemia (experimental and clinical), a similar but inverse phenomenon occurs, which explains the development of peaked, longer, and positive T waves (see also Figure 13.6). If more important subendocardial ischemia exists, the AP of the area will present not only longer duration but also smaller area. The sum of two AP explains the ECG pattern with some ST depression and tall T wave (C) (see text). In D, the AP of involved transmural area due, at least sometimes, to edema (E transmural edema seen in CV magnetic resonance) is longer but with similar shape of the rest of LV. The recording electrode faces the tail of ischemic vector and records negativity (deep negative T wave sometimes seen in the evolving phase of STEMI) (D and E) (Migliore et al. 2011). Table 13.1 ECG manifestations of acute myocardial ischaemia (in the absence of left ventricular hypertrophy and left bundle branch block). From Thygesen et al. (2019). a When the magnitudes of J‐point elevation in leads V2 and V3 are registered from a prior electrocardiogram, new J‐point elevation ≥1 mm (as compared with the earlier electrocardiogram) should be considered an ischemic response. Figure 13.8 Cross‐section of the left ventricular wall in diastole and systole. Factors involved in the susceptibility of the subendocardium to the development of ischemia include the greater dependence of this region on diastolic perfusion and the greater degree of shortening—and therefore of energy expenditure—of this region during diastole (Reproduced with permission from Bell and Fox 1974). The positive T wave seen in the hyperacute phase of ischemia is symmetrical and peaked, and often presents with high voltage and is usually preceded by a clear ST segment, because the T wave arises in the second phase of repolarization (Figures 13.6 and 13.7). This is especially seen in case of LAD involvement (Figure 13.10C). The ST segment in V1–V2 to V4–V5 may be depressed (Figure 13.10E). However, often there is no ST depression, probably because it is already in the first stage of ongoing ST elevation (Figure 13.10B,D). It is not always easy to detect these changes of T wave (peaked T wave) because they are sometimes minor. The earliest change is that the T wave becomes symmetric. Figure 13.9 The typical pattern of ischemic heart disease can be explained by the pathophysiology of ischemia studied with contrast‐enhanced cardiovascular magnetic resonance (CE‐CMR). Little or no cellular necrosis is found until about 15 minutes after occlusion. After 15 minutes, a “wavefront” of necrosis begins subendocardially and grows toward the epicardium over the next few hours. During this period, the infarcted region within the ischemic zone increases continuously toward a transmural infarction (Reproduced with permission from Marholdt et al. 2005). Figure 13.10 A normal variant tall T wave. B to E morphologies of taller than normal T wave in patients with ischemic heart disease. (A) Normal variant. (B) T wave very tall not preceded by rectified ST segment: this morphology is frequently observed as a transitory form to ST elevation. (C) A tall T wave, very symmetric and with rectified ST segment completely abnormal in V2 lead, which may be frequently observed in a hyperacute phase of STEMI before ST segment elevation (see Figure 20.4). (D) V2 lead: T wave with very wide base and straight ascendant slope of T wave that can be seen in a patient with an acute myocardial infarction. This pattern is transitional between the typical pattern of tall T wave of subendocardial ischemia to ST elevation. (E) Very tall and peaked T wave preceded by mild but evident ST depression. In spite of the presence of mild ST depression, this is a pattern of evolutive ST segment elevation acute coronary syndrome (STEMI) (see Figure 20.7) (Based on Wackers et al. 1978). Usually this tall and peaked T wave pattern is very transient (see Figure 20.4B‐1). However, sometimes the peaked and symmetric T wave, with or without mild ST depression, persists for hours and remains unchanged until it evolves into Q‐wave MI, often with clear ST elevation (Sagie et al. 1989, De Winter et al. 2008) (Figures 20.4B‐2 and B‐3). This was clearly seen in the cases described by Dressler and Roesler (1947) (see Figure 20.7). According to Birnbaum et al. (1993b), the cases of persistent tall T wave correspond to grade I of ischemia, although in the series reported by De Winter et al. (2008), there is at least a moderate increase of biomarkers. These cases have tight or even total LAD occlusion, but without transmural involvement due to collaterals/preconditioning. The amount of necrosis at this time is probably at least moderate but we can presume that this pattern does not represent at this moment complete transmural involvement. However, if not treated, it will usually develop into transmural homogeneous involvement with ST elevation Q‐wave MI (Dressler and Roesler 1947; Sagie et al. 1989) (Figures 20.6 and 20.7) (see Chapter 20). As mentioned earlier, the results of studies on the experimental occlusion of coronary arteries in animals with closed chest (Lengyel et al. 1957) agree with the ECG changes that often appear in various clinical settings of transmural ischemia and that are a consequence of coronary occlusion, such as coronary spasm (see Figure 20.46), hyperacute phase of STE‐ACS (Figure 20.4B) and PCI (see Figures 20.13 and 20.14), and also occasionally during exercise test (Table 25.6). In many cases, after a short period of peaked T wave with QT prolongation, if ischemia persists, an evident ST segment elevation appears and, later on, the ECG pattern evolves to Q‐wave MI (Figure 13.32 and 20.4B1). In addition, a tall, positive, and symmetrical T wave is frequently seen in V1–V3 in the chronic phase of lateral or inferolateral Q‐wave MI. This is not an expression of the subendocardial ischemia, it is a mirror pattern of a negative T wave seen in opposed leads as a window effect of Wilson (Figures 3.6E and 13.13). In clinical setting, the presence of flat/negative T wave is never present; except in myopericarditis, only a subepicardial involvement. Therefore, the theories that explain the presence of flat/negative T waves in experimental ischemia (see Figures 13.3–13.7) as a result of anoxia or similar damage in subepicardial area have a limited value in the clinical setting. The mechanism that explains the presence of T wave may be different according to the clinical situation: in post‐Q‐wave MI (see Figure 13.6E) or in the evolving course of an STEMI without Q‐wave MI (Figure 13.6F and 13.7D,E), or in NSTEMI (see later). According to the new universal definition of MI (Thygesen et al. 2019) (see Table 13.1 and later), the presence of T inversion ≥0.1 mV in two contiguous leads with prominent R or R/S ratio >1 is considered to be an ECG manifestation of clinical acute myocardial ischemia. It is true that during acute episode of chest discomfort, the pseudonormalization of previous inverted T wave may indicate acute ischemia (Figure 13.11), but the presence of a persistent negative T wave is an expression of a post‐ischemic change more than of acute ischemia. The following observations are in favor of the suggestion that a negative T wave does not appear during acute ischemia and that it corresponds to a post‐ischemic change (Nikus et al. 2010; Fiol‐Sala et al. 2020). Figure 13.11 (A) ECG with a quite negative T wave in V1–V2 to V5, with extension to I and aVL in a patient with ACS, without chest pain, corresponding to a critical lesion in the proximal part of the left anterior descending (LAD) coronary artery that practically normalizes during a chest pain crisis (B). This corresponds to an atypical pattern of ST segment elevation acute coronary syndrome (STE‐ACS) (see Figure 20.4C). The normalization of this pattern is an intermediate situation between the negative T wave and the ST segment elevation that would appear if the chest pain were more intense and prolonged. It is quite important to bear this in mind and to perform sequential ECGs, as the normal ECG during the angina crisis can provide quite confusing and dangerous information. Figure 13.12 ECG of a 55‐year‐old man without chest pain and with non‐ST segment elevation acute coronary syndrome (NSTE‐ACS) (unstable angina) and ECG with symmetric and mild negative T wave from V1 to V3. The coronarography shows important left anterior descending (LAD) proximal subocclusion. Negative T waves are abnormal if present in at least two contiguous leads (Thygesen et al. 2019) and may be located in different areas of the left ventricle according to the previously mentioned established correlations with different leads. In V1–V2, a peaked T wave may be seen as an expression of a mirror pattern of the lateral wall (see above). Figure 13.13 ECG with a typical pattern of chronic negative T wave in the leads facing the inferior wall (negative T wave in II, III, and aVF) and the lateral wall (positive peaked T wave in V1–V2). There is a necrosis in inferior wall with QR complex in II, III, and aVF. CE‐CMR shows that the affected area of lateral wall was the basal zone. Due to that, there is no RS pattern in V1. The morphology of negative T wave is symmetric and shows a mirror pattern in the frontal plane leads (Figure 13.13). Usually a well‐defined isoelectric ST segment is observed and sometimes only the second part of the T wave is negative (±) (Figure 13.11) because the T wave originates in the second phase of the repolarization. In general, the depth of a negative T wave depends on the intensity of the previous ischemia and on the extension of transmural homogeneous involvement. Therefore, it is much deeper (>4–5 mm in some leads) in the resolution of STE‐ACS without Q‐wave MI (Figure 13.11) or after evolved Q‐wave MI, or even after fibrinolysis than in cases of NSTE‐ACS (<1–2 mm or even flat T wave) (Figure 13.12). However, its duration is in relation to the reversibility of the left ventricular damage. In the case of coronary spasm, the depth of the negative T wave that appears after the crisis is an expression of the severity of the ischemia (grade III ischemia), but it is very transient (non‐irreversible damage) and disappears very soon (ischemia‐reperfusion pattern) (Figure 20.45). In contrast, after Q‐wave MI, the T wave may be more or less deep in different leads according to the intensity of the damage, but the duration may last forever (Figure 13.13). The disappearance of a negative T wave in these cases is a sign of good prognosis (Figure 13.32). Nevertheless, do not forget that the sudden pseudonormalization of a negative T wave in the evolution of ACS is always abnormal and represents an intermediate transient situation to an evolving ST elevation (see Figures 13.11 and 20.4C). The association of negative T wave due to primary alteration of repolarization (ischemia, drugs, electrolyte disturbances, etc.) with secondary alterations due to left ventricular enlargement (LVE) with strain, and/or left bundle branch block (LBBB), produces a mixed pattern. In these cases, the resultant negative T wave frequently appears more symmetric (Figure 13.14) (see Chapter 7, abnormalities of repolarization, and Chapter 10, LVH strain). Figure 13.14 Symmetric negative T wave (see leads I and V5) in a patient with hypertension and intermittent complete left bundle branch block, who presents with symmetric T wave when the LBBB disappears after a ventricular extrasystole. This is a mixed pattern (ischemia + LVH). Also the T wave of complexes with LBBB shows more symmetric morphology than in cases of isolated LBBB. A long list of conditions, other than IHD, may present with changes in the T wave (symmetric, wide, and/or taller than normal) (Fiol‐Sala et al. 2020). Table 13.2 summarizes the most important conditions, and Figure 13.15 illustrates some striking examples. Table 13.3 lists the more frequent causes of flattened or negative T wave aside from IHD. Figure 13.16 shows some striking examples. The most negative T waves usually appear in some cases of hypertrophic cardiomyopathy (HCM) (see Figure 21.2) and in some strokes or metabolic shock with long QT. Chronic pericarditis is the most important condition for a clinical differential diagnosis because this disease also presents with pain in the acute phase and even may present mild increase of biomarkers. The negative T wave of chronic pericarditis (see Figure 13.17) is usually more extensive, does not present mirror pattern in the frontal plane, and is less intense (negative) than in cases of IHD, especially in post‐Q‐wave MI. However, especially when associated myocarditis exists, the pattern may be more striking than a case of IHD (Figure 13.18). In fact, some type of epicardial involvement often exists in pericarditis. In this case, the pattern of flat/negative T wave is a result of clear subepicardial involvement (Figures 13.6 and 13.7). It is also important to know that flat or mildly negative T waves may appear transiently after alcohol ingestion (see Figure 23.18) or even after the administration of some drugs (see Figure 23.13). Table 13.2 Causes of taller‐than‐normal T wave (aside from ischemic heart disease; Figure 13.15). Figure 13.15 T wave morphologies in conditions other than ischemic heart disease. Tall peaked T wave in case of (A) variant of normality (vagotonia with early repolarization); (B) alcoholism; (C) left ventricular enlargement; (D) stroke; (E) hyperkalemia; (F) congenital atrioventricular block. Table 13.3 Causes of negative or flattened T waves (aside from ischemic heart disease) (Figure 13.16). The area of the left ventricular myocardium subepicardium or subendocardium or the zone of tissue (wedge preparation of left ventricular muscle) or a single heart muscle cell close to or far from the recording electrodes with significant and persistent ischemia (called the area of injury, term that we believe not very lucky because it means transmural ischemia) present due to different grades of depolarization during systole and diastole, two currents generated as a consequence of this important ischemia—one occurring during systole and the other during diastole (Hellerstein and Katz 1948; Cabrera 1958; Janse 1982; Franz 1983; Coksey et al. 1960) (Figures 13.19 and 13.20) (for more information, consult Fiol‐Sala et al. 2020). However, the ischemic current generated during systole is the only one considered here because ECG equipment is adjusted by alternating current (AC) amplifiers to maintain a stable isoelectric baseline during diastole, despite the existence of diastolic depolarization. Without this adjusted mechanism, the changes in the ECG generated by the transmural ischemia would be the sum of TQ plus ST deviations (depression or elevation) depending on whether the ECG faces the ischemic area or is in the opposite wall (Figure 13.20). Currently, in the surface ECG, we only recognize the consequences of this systolic depolarization by the modification that, according to the membrane response curve (Singer and Ten Eick 1971) (Figure 13.21), is induced in the AP of the affected area, which is converted to a “low‐quality” AP (slower upstroke, lower voltage, smaller area, etc.) (systolic ischemic current). This change in AP expression of the systolic ischemic current is responsible for the ST changes found in the surface ECG. The ST segment changes (ST deviations) caused by this important and persistent experimentally induced ischemia that produces a “low‐quality” TAP in the involved area varies depending on the location of the ischemia. An ST segment depression is observed if the ischemia affects the subendocardium or area far from the recording electrode, whereas an ST segment elevation is recorded if the experimental transmural ischemia affects the subepicardium or area close to the exploring electrode. Figure 13.16 T wave morphologies in conditions other than ischemic heart disease. Some morphologies of flattened or negative T wave: (A,B) V1 and V2 of a healthy 1‐year‐old girl; (C,D) alcoholic cardiomyopathy; (E) myxedema; (F) negative T wave after paroxysmal tachycardia in a patient with initial phase of cardiomyopathy; (G) bimodal T with long QT frequently seen after long‐term amiodarone administration; (H) negative T wave with very wide base, sometimes observed in stroke; (I) negative T wave preceded by ST elevation in an apparently healthy tennis player rules out HCM; (J) very negative T wave in a case of apical cardiomyopathy; and (K) negative T wave in a case of intermittent left bundle branch block in a patient with no apparent heart disease. Figure 13.17 A patient with chronic constrictive pericarditis. The T wave is negative in many leads, but not quite deep, without the “mirror pattern” in the frontal plane. The T wave is only positive in aVR and V1 because, as this is a diffuse subepicardial ischemia, they are the only two leads in which the ischemic vector that is directed away from the ischemic area is approaching the exploring electrode. Two theories have been suggested to provide an electrophysiologic explanation of these injury patterns found in areas with low‐quality TAP due to experimental subepicardial or subendocardial injury (Coksey et al. 1960; Samson and Scher 1960; Fiol‐Sala et al. 2020): Figure 13.18 (A) ECG of a patient with chronic ischemic heart disease. (B) ECG of a patient with myopericarditis. The ECG does not aid in this case in establishing the differential diagnosis. Even, the patient with myopericarditis shows more negative and deeper T waves. Figure 13.19 Local DC extracellular ECGs from the left ventricular subepicardium of an isolated pig heart before (control) and 6 and 8, minutes after coronary artery occlusion. Horizontal lines indicate zero potential. Note decrease in resting potential (TQ segment depression in extracellular complex) and reduction in action potential upstroke velocity with the appearance of ST segment elevation. After 8 minutes of occlusion, the ECG shows monophasic morphology. (There is a TQ depression and huge ST segment elevation.) After an hour, the ischemic zone becomes permanently unexcitable. Characteristically, some time before the ischemic cells become unresponsive, they showed electrical alternance of amplitude and duration of action potential (Adapted from Janse 1982). Figure 13.20 The electrode located in the epicardium of anterior cardiac wall records a simultaneous ST segment depression and T–QRS elevation after subepicardial ischemia of the lateral wall (arrow) is produced (Source: Adapted with permission from Hellerstein and Katz 1948). In Chapter 7, we commented on the limits of ST segment elevation and depression found in a normal population (see Figures 7.12–7.17). In the clinical setting, the ECG manifestation of acute ischemia in the absence of left ventricle hypertrophy or left bundle branch block, in respect to ST segment (elevation or depression), according to the new universal definition of MI (Thygesen et al. 2019), is described in Table 13.1. We will refer to this throughout this chapter and also in Chapter 20. Figure 13.21 Note the relationship between the value of the transmembrane diastolic potential (TDP) in millivolts and the velocity of response (response dV/dt). Figure 13.22 How the respective patterns of subendocardial (B) and subepicardial (C) experimental injuries are generated according to the theory that the normal ECG pattern (A) is the result of the sum of subendocardial and subepicardial transmembrane action potentials (APs). (B) The example explains the pattern in the case of subendocardial experimental work and also for subendocardial clinical ischemic. (C) This shows the case of experimental subepicardial ischemia. In the clinical setting (D), the ischemic is transmural, never only subepicardial. Therefore, the ST elevation in the clinical setting may be explained by the sum of low‐quality APs of the ischemic zone + normal AP of rest of left ventricle. Table 20.2 summarizes the clinical, electrophysiological, coronarographic, and pathological findings that usually differentiate STE‐ACS from NSTE‐ACS (Sclarovsky 1999; Wellens et al. 2003; Fiol‐Sala et al. 2020). ST segment depression in clinical practice usually appears because the subendocardium is more vulnerable to ischemic damage than the rest of the ventricular wall, and thus, it may be affected without transmural involvement if the occlusion is subtotal although the ischemia usually extends beyond the limits of anatomic subendocardium (Figure 13.23A‐2). When the subendocardial/subepicardial ratio is reduced to approximately 40%, the flow ratio decreases dramatically. This occurs during exercise and mental stress and also in other situations with increased left ventricular end‐diastolic pressure (Nikus et al. 2010). In these cases, the AP of the subendocardium due to extensive and already usually existent ischemia shows a fundamental change in its shape (see above T wave: experimental electrophysiologic mechanism) and because of that an ST depression appears as a consequence of the summation of the AP of subepicardium and subendocardium (Figure 13.22B) and in the leads facing the tail of the ischemic vector (Figure 13.23A‐2). When the number of involved leads is important, there are some leads with a mirror pattern (see Figures 13.28 and 13.29) (see Chapter 20). Figure 13.23 (A) In significant experimental subendocardial ischemia (1) (ischemic zone), the ischemic vector is directed from the non‐injured zone (with more negative electric charges) toward the ischemic zone (with fewer electric negative charges, being relatively positive in comparison with the non‐injured area). The ischemic vector faces the ischemic zone, while the ischemia and necrosis vectors are directed away from the involved area. Therefore, when significant experimental ischemia (injury) is located in the subendocardium (A‐1), the vector is directed from the subepicardium to the subendocardium and generates ST segment depression in the ECG leads facing the subepicardium. In clinical subendocardial ischemia, this is predominantly but not exclusively subendocardial (A‐2) and the ST depression may be explained by the same mechanism. (B) In the case of significant experimental subepicardial ischemia (ischemic zone (B‐1), the ischemic vector is directed toward the subepicardium because, in this case, the current flow runs from the subendocardium to subepicardium because the subepicardium shows less negative changes, and is relatively positive. This generates ST segment elevation in the ECG leads facing the subepicardium. In clinical practice, exclusive subepicardial ischemia does not exist. The ST depression appears in relation to the following mechanisms: The ischemia that first is subendocardial, soon becomes transmural. In this case, the surface ECG records the pattern as ST elevation (B‐2) because all the left ventricular transmurally ischemic walls are relatively less negative than the other zones of LV farthest from the electrode (see text). Figure 13.24 Observe how an amplified ECG (4×) of lead V1 allows the proper assessment of ST segment deviation. The patient had a previous anterior myocardial infarction, and there is mild ST segment elevation in the right precordial leads. Figure 13.25 Evolution of non‐Q‐wave myocardial infarction with important ST segment depression at the beginning which normalizes in few weeks (A–D). In the surface ECG, new ≥0.5 mm of ST depression horizontal or downsloping found in two consecutive leads measured at J point are sufficient to be considered pathological in patients with possible NSTE‐ACS (chest pain) (Table 13.1). It is important to record an amplified (×4) ECG to better assess these small ST changes (Figure 13.24). The morphology is usually flat or downsloping, and not quickly upsloping (Figures 13.25–13.30). The ST segment depression tends to normalize from the acute to subacute phase (Figure 13.25). If the ST depression is small, it is most probably a case of unstable angina. However, the distinction between unstable angina and non‐Q‐wave MI is shown by the biomarkers. We comment these aspects more extensively in Chapter 20 (see Table 20.4). Patients with NSTE‐ACS can be divided in two groups according to the number of leads with ST segment depression: Figure 13.26 Various types of subendocardial ischemia patterns may appear in the course of an exercise test: (A) horizontal displacement of the ST segment, (B) descendant displacement of the ST segment, (C) concave displacement, and (D) ST segment depression from J point with ascendant morphology and rapid upslope. This usually is seen in normal cases. The coronary angiography was abnormal in A, B, and C, and normal in D. These changes are especially visible in leads with dominant R wave, especially V3–V4 to V5–V6, I, and aVL, and/or inferior leads present with dominant R wave. Figure 13.27 Changes of the ST segment in a patient with exercise angina. (A) The trend of ST segment changes and heart rate; (B) the different morphologies of ST. Figure 13.28 (A) In the case of diffuse subendocardial circumferential injury due to tight subocclusion of the left main trunk (LMT) in a heart with previous important subendocardial ischemia, the ischemic vector that points to the circumferential subendocardial area is directed from the apex toward the base, from forward to backward and from left to right. This explains the typical morphology of ST segment depression in all the leads except aVR and V1, with maximal ST segment depression in V3–V5. As the ischemic vector faces more aVR than V1, the ST segment elevation is aVR > V1. (B) Typical ECG of LMT critical subocclusion. The ST segment depression is higher than 6 mm in V3–V5 and there is no final positive T wave in V4–V5. We comment on the ECG usually recorded during anginal pain. In around 50%, in the absence of pain, the ECG is normal/near normal. The more striking ST depression is found in V3–V5, V6 with or without final positive T wave and usually with evident positive ST elevation in aVR as a mirror pattern (Figures 13.28 and 13.29, 20.17, 20.19, and 20.20) (see legends, and Chapter 20). Figure 13.29 (A) Normal control ECG of a patient with chronic ischemic heart disease. (B) During non‐ST segment elevation ACS (NSTE‐ACS), diffuse and mild ST segment depression is seen in many leads, especially in I, V5, and V6 with small ST segment elevation seen in III, VR, and V1. The coronary angiography shows three‐vessel disease with important proximal obstruction in the left anterior descending (LAD) and left circumflex artery (LCX). Figure 13.30 Top panel. This ECG was recorded during an episode of chest pain in a patient with single‐vessel coronary artery disease. On angiography, there was a 95% stenosis of the midpart of the left circumflex artery (LCX). The ST segment is depressed in leads I, II, aVF, aVL, and V2–V6. These leads with ST depression show positive T waves. The ST segment is elevated in leads aVR and V1. Bottom panel. A second ECG recorded when the patient was pain free showed no significant ST changes. This abnormal ECG pattern is recorded in patients with left main coronary artery subocclusion or equivalent (2–3 proximal vessels disease), and also in cases of very proximal subocclusion of long LAD, even without important compromise of other arteries, or rarely in proximal subocclusion of dominant LCX. To identify, with some limitation, the involved (culprit) artery, we have to look especially to aVR (check ST elevation) (remember that aVR = I + II/2) (Chapter 7), and mid precordials leads (Table 20.4 and Chapter 20). In cases of severe 2/3 vessel disease, the ECG pattern may be similar but frequently either the ST depression is less deep or the final T wave is more positive (Figure 20.19). In tight LAD subocclusion, the ECG pattern presents less ST depression and taller T wave. Furthermore, in aVR, the ST elevation is usually <1 mm (Figure 20.21). Figure 13.31 Patient with subocclusion of the LMT. The figure shows the sequence of progressive changes of the ST segment and the T wave obtained through monitoring with the MIDA system (Ortivus Medical AB, Sweden), pending coronary angiography. When the patient experienced anginal pain, an increase of the ST segment depressions was seen in I, aVL, and from V2 to V6 with a final negative T wave in V5–V6. There is also mild ST segment elevation in III during almost the entire sequence (somewhat less when the patient was pain free, row 4). At the time of maximal pain, the ST of aVR was higher than in V1. The first and second rows show a pattern of circumferential subendocardial ischemia. Rows 3–7 show some resolution with less ST segment depression and positivization of the terminal part of the T waves, but after that the situation worsened. Finally, in rare cases, a tight proximal subocclusion of long LCX shows an ECG pattern usually with prominent R wave and ST depression is usually not very deep (Figure 20.21). In Chapter 20, Table 20.4 lists the different groups of ECG pattern that may be found. Although it is, in general, difficult to identify which is the culprit artery, may be useful to remember the following: It should be remembered that ST depression in V1, V3–V4 may be an expression of true ST elevation myocardial infarction (STEMI equivalent) (mirror pattern) in the case of LCX total occlusion with transmural involvement (see above) (see Figure 20.5). These patients present with transmural involvement but usually do not have a previous important ischemia. The surface electrodes record an ECG pattern of ST elevation (Figures 13.22D and 13.23B‐2). The mechanisms that explain the ECG pattern of subepicardial injury in clinical setting are based on the theory of summation of AP of transmural affected zone and the rest of the LV and, in theory, of injury vector which have been explained before (see Figures 13.22D and 13.23B‐2). To explain the presence of a tall T wave in the early phase (see above) (Figures 13.3, 13.6, 13.7, 13.10, and 20.4B), we have to consider that before the injured zone is transmural, the effect of ischemia is exclusively or predominantly subendocardial. This explains the presence of a tall, usually transient, T wave, which in a few number of cases (1–2% of ACS) is more or less persistent (Figures 20.7 and 20.9). Because the ischemic patterns develop at the end of the depolarization, which corresponds to the end of QRS at the beginning of repolarization, the ECG expression of injury starts during the first part of the ST segment. In contrast, the ischemia pattern appears in the second part of repolarization, after a clear ST segment. Therefore, in cases of relevant ischemia, the final part of QRS (S wave) is pulled up, which is followed by striking ST segment elevation (Figures 13.34 and 20.3C) (see later “Distortion of Q wave” and Chapter 20). Figure 13.32 A flow chart drawing of the ECG changes in case of Q‐wave anterior myocardial infarction: records in V1–V2 from the very onset (rectified ST and positive T wave) until the different evolution patterns that may be present throughout a year in the case where no treatment has been administered. An ST segment elevation may also be recorded in other clinical settings of ischemia that are not related to atherothrombosis, such as isolated coronary spasm (see Figure 20.47) and Tako–Tsubo syndrome (Figure 20.44) (see Chapter 20). The ST segment elevation that appears in the course of ACS with ST elevation will evolve to Q‐wave MI if it is not aborted (see Chapter 20). Before the ST elevation, a tall and wide T wave usually exists for a short period. The ST segment elevation tends to normalize from the acute to the chronic phase (Q‐wave MI) if the MI is not aborted with treatment (Figures 13.32–13.34 and 20.36) (see Chapter 20, Acute coronary syndrome with narrow QRS). In these cases, it is very important to note the presence of mirror patterns (Figures 13.35–13.37). The leads that face the head of the vector of ischemia in transmural acute ischemia record an ST segment elevation, whereas the leads facing the tail of the ischemic vector record an ST segment depression. The global study of direct and mirror images of the ST deviations in different leads is crucial to correctly diagnose the location of the ischemia in the different walls of the heart (see later) and to understand the corresponding prognostic implications (Chapter 20). Figure 13.33 Different types of tissues (normal, ischemic, injured, and necrotic—from 1 to 4) (A) and (B). In each of them the corresponding electrical charges are shown—decreasing in steady fashion. Levels of Ki+/Ke+ (C), transmembrane action potential (AP) morphologies and the level of the transmembrane diastolic potential (DP) (D), the subendocardial and subepicardial AP (E), the corresponding patterns that are recorded in the ECG (F), considering that it is a transmural involvement and the pathological findings that are found (G). Note that necrotic tissue is non‐excitable (does not generate an AP) due to the marked diastolic depolarization that it shows. Figure 13.34 Acute infarction of anteroseptal zone with ST segment elevation in the pre‐fibrinolytic era. Evolutionary phases: (A) at 30 minutes, (B) 1 day later, (C) 1 week later, and (D) 2 weeks later. Different authors (Birnbaum et al. 1993a; Engelen et al. 1999; Chia et al. 2000) have described the value of ECG in localizing the occlusion site in STE‐ACS. Figure 13.38 shows an algorithm that allows us to predict the site of the LAD occlusion in the case of ACS with ST elevation in the precordial leads (Fiol et al. 2009) and Figure 13.39 shows an algorithm that we follow in the case of ACS with ST elevation in the inferior leads to distinguish between RCA or RCX occlusion (Fiol et al. 2004a, 2004b). Other algorithms, including one with “allcorners” with STEMI have also been published with good results (Tierala et al. 2009). The statistical power of these criteria in our experience (Fiol‐Sala et al. 2020) and other studies (Birnbaum et al. 1993a; Engelen et al. 1999; Tierala et al. 2009) is very high (see Figures 13.38 and 13.39). In the case of RCA occlusion, there are increased chances of a proximal occlusion and right ventricle involvement if an ST elevation in the right precordial leads V3R–V4R is present (Wellens 1999). In our experience, we found that the presence of isoelectric or elevated ST in V1 is also useful (Fiol et al. 2004a, 2004b). Furthermore, in the case of occlusion of very long RCA occlusion (extra‐large RCA), an ST elevation in V5–V6 is usually seen. ST elevation may even be seen in proximal occlusion of the RCA (ST elevation in V1–V3) due to proximal occlusion and in V4–V6 due to very long RCA (Eskola et al. 2004). In small number of these cases, the ST elevation may be seen in all the precordial leads (Garcia Niebla et al. 2009) (Figure 13.40). Figure 13.35 In an acute coronary syndrome with ST segment elevation in V1–V2 to V4–V6 as the most striking pattern, the occluded artery is the left anterior descending coronary artery (LAD). The correlation of the ST segment elevation in V1–V2 to V4–V5 with the ST morphology in II, III, and aVF allows us to know whether it is an occlusion proximal or distal to 1st diagonal. If it is proximal, the involved muscular mass in the anterior wall is large and the ischemic vector is directed not only forward but also upward, even though there can be a certain inferior wall compromise because of long left anterior descending (LAD). This explains the negativity recorded in II, III, and aVF. In contrast, when the involved myocardial mass in the anterior wall is smaller, because the occlusion is distal to 1st diagonal, if the LAD is long, as usually occurs, the ischemic vector in this U‐shaped infarction (inferoanterior) is of course directed forward, but often somewhat downward instead of upward, and so it generally produces a slight ST segment elevation in II, III, and aVF (see Figure 13.38). Figure 13.36 In an acute coronary syndrome with ST segment elevation in II, III, and aVF as the most striking abnormality, the study of the ST segment elevation and depression in different leads will allow us to determine whether the occluded artery is the right coronary artery (RCA) or the left circumflex artery (LCX) and even the site of the occlusion and its anatomical characteristics (dominance, etc.). This figure shows that the presence of ST segment depression in lead I means that this lead is facing the ischemic vector tail that is directed to the right and, therefore, the occlusion is located in the RCA. In contrast, when the occlusion is located in the LCX, lead I faces the ischemic vector head and, in this case, it is directed somewhat to the left and will be recorded as an ST segment elevation in lead I. To check the type of ST segment deviation in lead I is the first step of the algorithm for identification of the occluded artery (RCA or LCX) in the case of acute coronary syndrome with ST segment elevation predominantly in inferior leads (see Figure 13.39). Figure 13.37 In the case of high septal involvement due to left anterior descending (LAD) occlusion proximal to S1 branch, the ischemic area produces a vector of ischemia directed upward, to the right and forward. Vector of ischemia in the horizontal plane (A) and frontal plane (B). This explains the presence of ST segment elevation in aVR and V1 and ST segment depression in II, III, aVF, and V6 (see text). Figure 13.38 Algorithm to precisely locate the left anterior descending (LAD) occlusion in the case of an evolving myocardial infarction with ST elevation in precordial leads. See inside the figure the sensitivity, specificity, and positive predictive value (PPV) of all these criteria (Based on Fiol et al. 2004b). Figure 13.39 Algorithm to predict the culprit artery (right coronary artery (RCA) or left circumflex artery (LCX)) in the case of evolving myocardial infarction with ST elevation in inferior leads (see the text for details). See inside the figure the sensitivity, specificity, and positive predictive value (PPV) of all these criteria (Based on Fiol et al. 2004b). Cases of complete occlusion of the left main trunk are very infrequent, and usually present as cardiogenic shock or cardiac arrest due to ventricular fibrillation. However, the survivors present an ECG with ST elevation similar to cases of proximal LAD occlusion but without ST elevation in V1 because this is balanced by the ST depression due to LCX involvement. The ECG often presents also RBBB and/or SA hemiblock (occlusion before first septal branch) (see Figure 20.3). The correlation between the precordial leads with ST elevation and site of occlusion has some limitations (Huang et al. 2011, 2016). This may be for several reasons, such as differences in the location of the precordial leads, different terminologies used, cancellation of vectors, presence or not of small or large conal branch of the RCA, which, if large, may supply alternative blood to the high septum during occlusion of LAD (Ben‐Gal et al. 1998), different lengths of coronary artery (Zhong‐Qu et al. 2009), rapid changes from one to another ECG pattern in relation to the sudden change of the location and intensity of the occlusion (see Figure 20.9), that we comment with some extension in Chapter 20 (see ECG patterns in STEMI. Location of occlusion). Figure 13.40 ECG and coronarography of a patient with ST elevation myocardial infarction (STEMI) due to proximal occlusion of dominant the right coronary artery (RCA). See ST depression in I and aVL (aVL > 1) and ST elevation in II, III, aVF (III > II), and all precordials (V1–V3–V4 for right ventricular infarction and V5–V6 for dominant RCA). Nevertheless, taking all these factors into consideration, we believe that the correlation we have shown by just taking a glance the surface ECG has an extraordinary value to stratify risk and take the best decision in many cases of STE‐ACS (see Chapter 20). Figures 13.41–13.52 illustrate some examples of how the changes of ST segment correlate with the area at risk, the coronary artery involved, and the site of occlusion in the presence of STE‐ACS, with the limitations just explained (see legends for explanation) and Figures 13.38 and 13.39. We do not show coronariography for limitation of space (for more details including coronariography of all these cases, consult Fiol‐Sala et al. 2020). In cases of left ventricular hypertrophy (LVH) with strain pattern and/or wide QRS complex, the ECG diagnosis of transmural ischemia pattern is frequently more difficult, especially in the presence of an LBBB or pacemaker. However, in the course of ACS, especially those secondary to the total proximal occlusion of an epicardial coronary artery, ST segment elevation are clearly seen in the presence of complete RBBB (Figure 13.53). Also, the presence of complete LBBB or pacemaker in the case of STE‐ACS often allows us to visualize the ST segment elevation clearly (Figures 13.54–13.56). Sgarbossa et al. (1996a) have reported that in cases compatible with acute MI, a diagnosis of evolving infarction associated with a complete LBBB is supported by the following criteria (Figures 13.54 and 13.55): (i) ST elevation >1 mm concordant with the QRS complex; (ii) ST depression >1 mm concordant with the QRS complex; (iii) ST elevation >5 mm non‐concordant with the QRS complex (e.g. in V1–V2). This last criterion is of less value when the QRS complex has an increased voltage (Madias et al. 2001). Concordant ST elevation has proved to be the single‐most specific criterion for the diagnosis of acute MI in the presence of LBBB, and improves the detection of this “STEMI equivalent” (Lopes et al. 2011).
Chapter 13
Ischemia and Necrosis
Concept
Experimental mechanisms of ischemia
ECG changes after experimental occlusion of a coronary artery
Experimental cooling and warming the epicardial zone
Correlation with electrophysiological and ECG changes
Changes in transmembrane action potential
Changes in the surface ECG (Fiol‐Sala et al. 2020; Nikus et al. 2010) (see later)
Changes of repolarization
Changes of depolarization
Other changes
Correlation with pathologic changes
Correlation between ECG changes, the coronary artery involved, and the affected left ventricular walls
Changes of repolarization: T wave
Experimental electrophysiological mechanisms
) will face the more ischemic zone. However, as this zone still has negative changes, a flow of current having a vectorial expression is generated going from more ischemic to less ischemic area. Therefore, the ischemic vector directed away from the ischemic area produces a more positive T wave in experimental subendocardial ischemia and a flat or negative T wave in experimental subepicardial ischemia. Note that the vector of necrosis is also directed away from the necrosis area (see Figure 13.62), but in contrast, the vector of ischemia is directed toward the injured area (Figure 13.24).
Changes of T wave in patients with ischemic heart disease (see Table 13.1 and Chapter 20)
Peaked, symmetrical, wider, and/or taller T wave (Figure 13.10)
Mechanism
ST elevation
New ST elevation at the J‐point in two contiguous leads with the cut‐point: ≥1 mm in all leads other than leads V2–V3 where the following cut‐points apply: ≥2 mm in men ≥40 years; ≥2.5 mm in men <40 years, or ≥1.5 mm in women regardless of agea
ST depression andT‐wave changes
New horizontal or downsloping ST depression ≥0.05 mV in two contiguous leads; and/or T inversion ≥0.1 mV in two contiguous leads with prominent R wave or R/S ratio >1
Morphology and duration
Flat or negative T wave
Mechanism
Morphology and duration
Diagnosis of negative primary T wave due to ischemia or other causes in patients with left ventricular hypertrophy and/or wide QRS
T wave changes in other heart diseases and situations
Differential diagnosis
Taller T wave
Flat or negative T wave
May sometimes be diffuse (global T wave inversion of unknown origin). More frequently observed in women
Changes of repolarization: ST segment
Experimental electrophysiologic mechanisms
ST segment deviations in patients with ischemic heart disease (see Tables 13.1 and 20.2)
ST segment depression (Figures 13.22–13.30)
Mechanism
Morphology and duration
The number of leads with ST depression and the coronary artery involved in patients with NSTE‐ACS
ST depression in seven or more leads (extensive involvement)
ST depression in fewer than seven leads (regional involvement) (Figure 13.30)
ST segment elevation: evolving STEMI (Figures 13.32–13.52) (see Chapter 20)
Mechanism (Figures 13.22, 13.23, 13.32, and 13.33)
Morphology and duration
Location of occlusion in STE‐ACS
Diagnosis of ST segment changes as a result of ischemia in patients with left ventricular enlargement and/or wide QRS
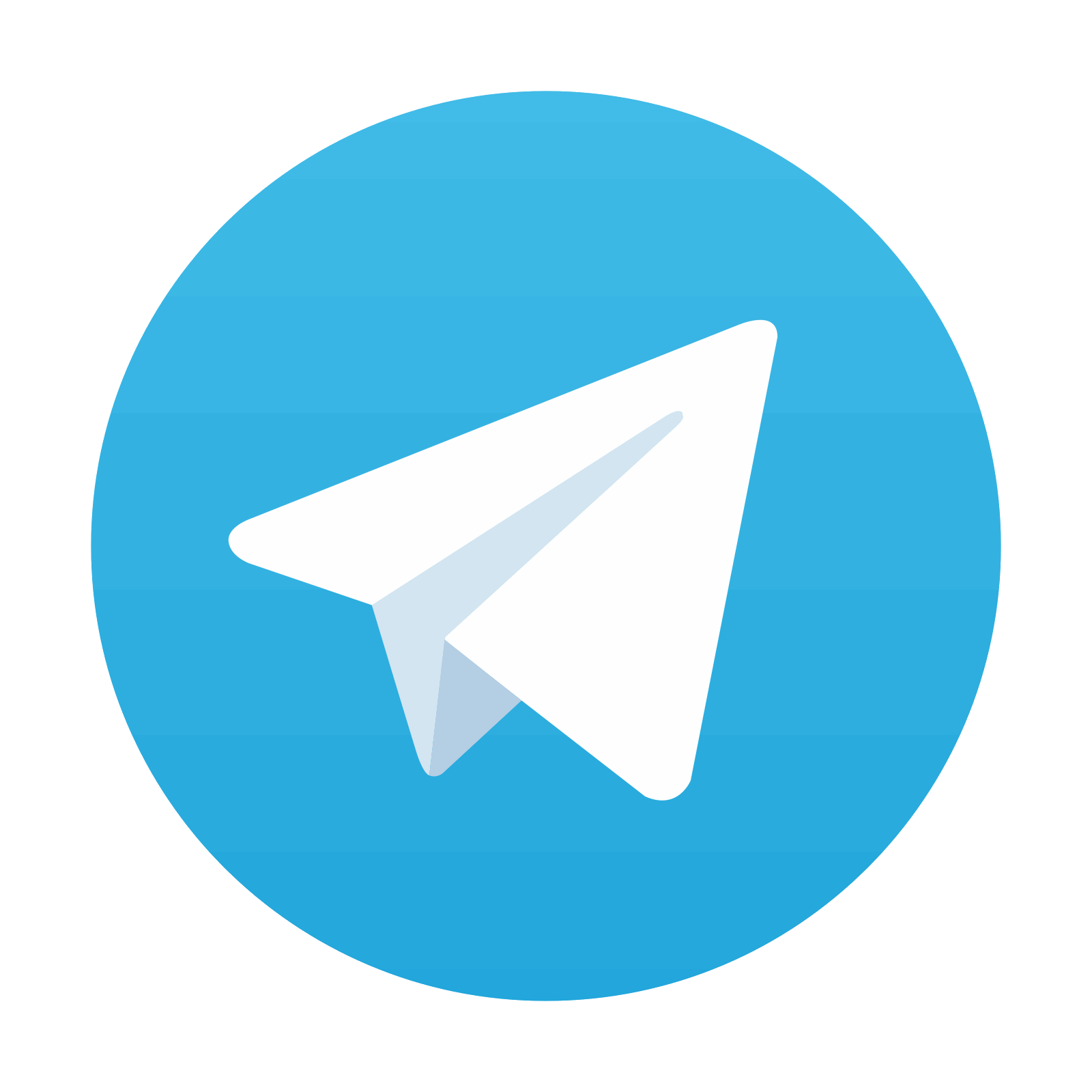
Stay updated, free articles. Join our Telegram channel

Full access? Get Clinical Tree
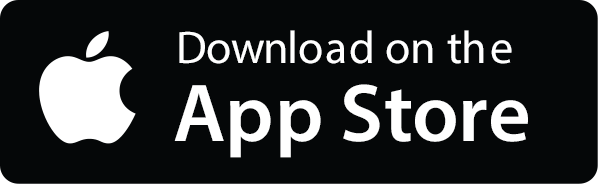
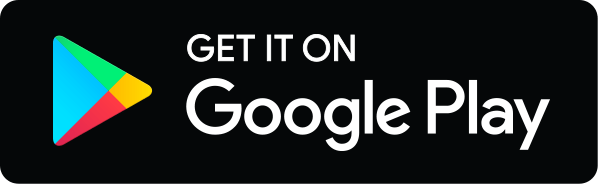