Abstract
In animal experiments, cooled ablation overcomes the limitations of standard radiofrequency (RF) delivery by cooling the catheter tip and preventing an impedance rise, thus allowing higher power delivery and resulting in deeper and larger lesions. In the clinical setting, efficacy of cooled-tip RF ablation is comparable to or better than conventional RF ablation for the catheter-based treatment of recurrent atrioventricular reentrant arrhythmias, reentrant atrial flutters, atrial fibrillation, and ventricular tachycardias. Temperature monitoring is less reliable for cooled-tip than noncooled-tip RF ablation. Other monitoring parameters, such as impedance, power, and contact force, during RF ablation are important. The safety profile of cooled-tip RF ablation is comparable to conventional RF ablation. Further developments in catheter design are targeting real-time assessment of ablation lesion formation and effectiveness.
Keywords
arrhythmias, cooled, impedance, irrigated tip, power, radiofrequency ablation
Key Points
- •
In animal experiments, cooled ablation overcomes the limitations of standard radiofrequency (RF) delivery by cooling the catheter tip and preventing an impedance rise, thus allowing higher power delivery and resulting in deeper and larger lesions.
- •
In the clinical setting, efficacy of cooled-tip RF ablation is comparable to or better than conventional RF ablation for the catheter-based treatment of recurrent atrioventricular reentrant arrhythmias, reentrant atrial flutters, atrial fibrillation, and ventricular tachycardias.
- •
Temperature monitoring is less reliable for cooled-tip than noncooled-tip RF ablation. Other monitoring parameters, such as impedance, power, and contact force, during RF ablation are important.
- •
The safety profile of cooled-tip RF ablation is comparable to conventional RF ablation.
- •
Further developments in catheter design are targeting real-time assessment of ablation lesion formation and effectiveness. There will be more widespread use of irrigated tip and contact force sensor to enhance efficacy and safety of RF ablation.
Radiofrequency (RF) ablation has become the standard of therapy for supraventricular tachycardia (SVT), including atrial fibrillation (AF), and ventricular tachycardias (VTs). More recently, RF ablation has also been used increasingly for the treatment of more complicated arrhythmias, particularly VT associated with structural heart disease. Although the results are promising, RF current delivered through a standard 7 F, 4-mm-tip electrode catheter is limited to ablation of arrhythmogenic tissue located within a few millimeters of the ablation electrode. In 1% to 10% of patients with accessory pathways and in 30% to 50% of patients with nonidiopathic VT, the arrhythmogenic tissue cannot be destroyed using a conventional ablation catheter. The overall success rate in these cases may be improved using alternative technologies for RF application that increase lesion size and depth.
Reducing the temperature of the ablation catheter tip has proven to be a solution for increasing the duration and power of RF application, decreasing the impedance rise and coagulum formation, and thereby developing a larger and deeper lesion. With the U.S. Food and Drug Administration (FDA) approving the use of several irrigated-tip ablation catheters in the past few years, there have been significantly increasing uses of this kind of catheter to ablate various supraventricular and ventricular arrhythmias. The aim of this chapter is to review current understanding of the mechanism of irrigated-tip and cooled-tip catheter ablation, as well as the results of animal studies and clinical trials that have used this technology.
Biophysics of Cool Radiofrequency Ablation
During RF application, delivery of RF current through the catheter tip results in a shell of resistive heating, which serves as a heat source that conducts heat to the myocardium ( Fig. 3.1 ). The shell of resistive heating is thin and within a few millimeters of diameter and is only somewhat greater than the diameter of the electrode tip. Conductive heat is thought to be responsible for thermal injury several millimeters away. For any given electrode size and tissue contact area, RF lesion size is a function of RF power level and exposure time. At higher power, however, the exposure time is frequently limited by an impedance rise that occurs when the temperature at the electrode–tissue interface reaches 100°C, because tissue desiccation and steam and coagulum formation occur at this temperature. The impedance rise limits the duration of RF current delivery, the total amount of energy delivered, and the size of the lesion generated. Please refer to Chapters 1 and 2 for details.

Although currently used temperature-controlled RF delivery systems are able to minimize the incidence of coagulum formation and impedance rise, the power applied is usually decreased and the lesion size is limited. During temperature-controlled RF ablation, the tip temperature, tissue temperature, and lesion size are affected by the electrode–tissue contact in addition to the cooling effects resulting from blood flow. With good contact between catheter tip and tissue and low cooling of the catheter tip, the target temperature can be reached with little power, resulting in fairly small lesions even though a high tip temperature is being measured. By contrast, a low tip temperature can be caused by a high level of convective cooling, which results in higher power consumption to reach the target temperature, yielding a relatively larger lesion.
Two methods have been used to cool the catheter tip, prevent the impedance rise, and maximize power delivery. In one approach, larger ablation electrodes (8 F, 8–10 mm in length) are used. The larger electrode–tissue contact area results in a greater volume of direct resistive heating. In addition, the larger electrode surface area exposed to blood results in greater convective cooling of the electrode by the blood. This cooling effect helps to prevent an impedance rise, allowing longer application of RF current at higher power, which produces a larger and deeper lesion.
An alternative approach described by Wittkampf and coworkers is to irrigate the ablation electrode with saline to cool down the electrode–tissue interface temperature and prevent an impedance rise. This approach allows cooler saline to bathe the ablation electrode internally or externally, dissipating heat generated during RF application ( Fig. 3.2 ). It decreases the electrode–myocardial interface temperature and allows for a larger amount of RF current to be passed before heating of tissue that results in the development of impedance rises and pops. Compared with conventional RF application, cooled ablation allows passage of both higher powers and longer durations of RF current with less likelihood of impedance rises. In addition, because convective cooling from the bloodstream is not required, an irrigated electrode may be capable of delivering higher RF power at sites of low blood flow, such as within the ventricular trabecular crevasse.

During cooled ablation, as the RF current is passed through the electrode to the myocardium, resistive heating still occurs at the electrode–myocardial interface. However, unlike with standard RF application, the area of maximum temperature with cooled ablation is within the myocardium, rather than at the electrode–myocardial interface. Nakagawa et al. demonstrated that the maximum temperature generated by cooled RF application will be several millimeters away from the electrode–myocardial interface as a result of active electrode cooling. In a study by Dorwarth and coworkers, the hottest point extended from the electrode surface to 3.2 to 3.6 mm within the myocardium, from the electrode–tissue interface for cooled ablation modeled with a catheter and cooled by internal perfusion of saline. Therefore tissue temperature generated during cooled RF ablation increases from the electrode tip to a maximum temperature a couple of millimeters within the myocardium. The current density and the width of the shell of resistive heating are increased around the electrode–myocardial interface, resulting in a larger effective radiant surface diameter and larger lesion depth, width, and volume.
Because the catheter tip is cooled actively, the temperature at the tip–tissue interface during cooled RF application is unreliable as a marker for determining the duration of RF application. Limiting tip temperature to less than 100°C prevents almost all impedance rises with conventional RF application. However, because the maximum tissue temperature is several millimeters away from the catheter tip during cooled ablation, the maximum tissue temperature may not be accurately monitored by a tip thermistor or thermocouple. Although RF current is increased with cooled RF application, intramyocardial tissues could be heated to 100°C, which would result in intramyocardial steam and crater formation, possibly associated with dissection, perforation, and thrombus formation. The maximum temperature may now be intramyocardial and surrounded by cooler areas of tissue. Some animal studies suggest that the optimal power required to avoid large craters are no greater than 50 W for an internally cooled catheter, or 20 W for an irrigated catheter. Wharton and coworkers demonstrated that impedance rises may be minimized to less than 6.3% if tip temperatures are maintained at less than 45°C. Nibley and coworkers also showed that a constantly maintained power of 50 W for the internally cooled catheter tip may deliver the maximum energy. Further studies are needed to expand these observations over a range of catheter types and clinical conditions to better understand how to limit power in cooled RF ablation in humans to prevent crater formation.
Design of Irrigated- and Cool-Tip Radiofrequency Catheters
Cooling of the catheter tip during RF ablation is achieved by circulating saline through or around the tip of the ablation catheter while RF current is being delivered. In general, there are two types of irrigation catheters. The first type is the closed-loop irrigation catheter, which has an internal thermocouple and continuously circulates saline within the electrode tip, internally cooling the electrode tip. The second type is the open-irrigation catheter (OIC), which has an internal thermocouple and multiple irrigation holes located around the electrode, through which the saline is continuously flushed, providing both internal and external cooling. Four different cooled catheters have been designed as shown in Fig. 3.3 . The internally cooled catheter (Boston Scientific Electrophysiology, San Jose, CA) has an internally cooled (or chilled) tip electrode that is perfused with room-temperature saline ( Fig. 3.4A ). With this closed-loop system, saline perfuses the tip of the catheter through a conduit in the catheter shaft and returns back through a second conduit in the catheter. Saline is not infused into the body (see Fig. 3.4 ).


One of the other designs investigated in vivo and in vitro is a screw-tip needle electrode, through which saline and contrast material could be infused during RF ablation. This specially designed electrode tip has been demonstrated to create a larger lesion in canines. A newly developed long irrigated ablation catheter (tripolar; 7 F; length of each electrode, 22 mm; interelectrode distance, 2 mm; helix radius, 9 and 10 mm) covered by a porous membrane to provide continuous irrigation could create longer and deeper lesions in vivo.
The Chilli cooled RF ablation system (Boston Scientific Electrophysiology; see Fig. 3.4 ) is approved by the FDA for use in patients with nonidiopathic VT. In clinical applications, cooling is achieved by pumping saline (0.6 mL per second) to the tip of the catheter during RF application. RF energy is titrated to achieve an electrode temperature between 40°C and 50°C, to a maximum of 50 W.
The other cooled RF ablation systems that are available are showerhead-type irrigated-tip catheter (Biosense Webster, Diamond Bar, CA and St. Jude Medical, St. Paul, MN). The ThermoCool ablation catheter (Biosense Webster; Fig. 3.5 ) is also approved by the FDA for AF ablation. Cooling is achieved with saline infused at a rate of 17 or 34 mL per minute during RF application and 2 mL per minute during all other times. A new addition is the Therapy Cool Path ablation catheter from St. Jude Medical, which is a 4-mm externally irrigated ablation catheter with six equidistant ports with a nominal flow rate of 2 and 13 mL per minute during ablation ( Fig. 3.6A ). The maximum power setting is 50 W, and it has thermocouple temperature monitoring at the maximum set temperature of 50°C. The Therapy Cool Path Duo (St. Jude Medical) irrigated-tip ablation catheter will be introduced soon with two sets of six ports evenly distributed on the distal and proximal portion of the tip electrode ( Fig. 3.6B ).


Yokoyama and coworkers found that open-irrigation systems resulted in greater interface cooling with lower interface temperatures and lower incidences of both thrombus formation and steam pops than seen with closed-loop irrigated cooled-tip catheters.
Animal Studies
Several authors have compared cooled RF catheter ablation with conventional ablation using animal models.
Nakagawa and coworkers evaluated cooled ablation. They compared conventional RF current delivery without irrigation with saline irrigation through the catheter lumen and ablation electrode at 20 mL per minute in a canine thigh muscle preparation. In the saline irrigation group despite the tip-electrode temperature not exceeding 48°C and electrode–tissue interface temperature not exceeding 80°C, the largest and deepest lesions (9.9 and 14.3 mm, respectively) were noted. They also demonstrated that the maximum tissue temperature during cooled ablation of 94.7°C occurred 3.5 mm from the tip of the electrode as opposed to conventional ablation where maximum temperatures were recorded at the electrode–tissue interface ( Fig. 3.7 ). Mittleman and coworkers also demonstrated that the use of a saline-irrigated luminal electrode with an end hole and two side holes (Bard Electrophysiology, Haverhill, MA) in canine myocardium in vivo at 10 to 20 W produced significantly larger lesions than a standard catheter ( Figs. 3.8 and 3.9 ). Dorwarth and coworkers compared three different actively cooled systems (showerhead electrode tip, porous metal tip, and internally cooled system) with standard 4- and 8-mm ablation catheters in isolated porcine myocardium. They found that the externally cooled systems had the largest lesion depth and diameter followed by the internally cooled system, which had a similar lesion depth with a slightly smaller diameter. The 8-mm tip had a similar lesion diameter with smaller depth. However, there were no differences in lesion volumes between the three cooled and the 8-mm ablation catheters. Maximum lesion volume was induced at a power setting of 30 W for the two open-irrigated systems and 20 W for the internally cooled catheter.



Larger electrode diameter or length during conventional RF application may generate larger lesions. However, Nakagawa and coworkers demonstrated an inverse relationship between electrode size and lesion size during RF application with actively cooling electrode perpendicular to the tissue. A 2-mm electrode delivered 49% more heating power than a 5-mm electrode; the latter lost more current to the surrounding blood, decreasing the effectiveness of the RF current to the targeted region. In the perpendicular electrode–tissue orientation, RF applications at 50 W resulted in lower power with the 2-mm electrode compared with the 5-mm electrode (26 vs. 36 W, respectively), but higher tissue temperature, larger lesion depth (8.0 vs. 5.4 mm), and greater diameter (12.4 vs. 8.4 mm). With the electrode parallel to the tissue, the overall power was lower with the 2-mm electrode (25 vs. 33 W), but tissue temperatures were higher and lesions were deeper (7.3 vs. 6.9 mm) with similar lesion diameters for both electrodes. Therefore if the cooling is adequate, the smaller actively cooled electrode transmitted a greater fraction of the RF power to the tissue and resulted in higher tissue temperatures and larger lesions.
Flow rates of saline infusion may also affect the size of a lesion created by cooled ablation. A higher flow rate might have a greater cooling effect on the catheter tip, which could potentially generate a larger lesion, while wasting more RF current as a result of overcooling. By contrast, a lower flow rate might result in a lesion size approaching that of conventional RF ablation. Weiss and coworkers compared three flow rates (5, 10, and 20 mL per minute) on sheep thigh muscle preparations ( Table 3.1 ). There were no differences in tip temperature or thrombus formation or power delivery to deeper tissues. The higher flow rate (20 mL per minute), however, did result in a smaller surface diameter lesion.
Parameters of Radiofrequency Application | Irrigation Flow Rate (mL per minute) | ||
---|---|---|---|
5 ( n = 15) | 10 ( n = 14) | 20 ( n = 14) | |
Total power | 929 ± 12 | 939 ± 12 | 935 ± 5 |
Maximum impedance (W) | 133 ± 13 | 125 ± 12 | 113 ± 12 |
Maximum catheter-tip temperature (°C) | 43 ± 3 | 39 ± 3 | 37 ± 3 |
Maximum tissue temperature (°C) | |||
| 79 ± 8 a | 67 ± 5 | 57 ± 4 |
| 57 ± 4 | 67 ± 5 | 58 ± 6 |
Audible pops | 0 | 0 | 0 |
Thrombus formation | 0 | 0 | 0 |
a P < .01 versus 10 and 20 mL per minute. All radiofrequency applications were achieved with a 30 W power output and a 30 second pulse duration.
Temperature monitoring during cooled RF application may be an unreliable marker because the actual surface temperature is underestimated. In the design of a longer catheter tip (6–10 mm) for increased convective cooling of the catheter tip, Petersen and coworkers found a negative correlation between tip temperature reached and lesion volume for applications in which maximum generator output was not achieved, whereas the delivered power and lesion volume correlated positively. They also directly examined the tissue temperature and lesion volumes formed by a showerhead-type cooled tip in the setting of either temperature control or power control. Power-controlled RF ablation at 40 W generated lesions that were similar to those achieved with temperature control at both 80°C and 70°C, as opposed to 60°C at which the lesions were significantly smaller. Importantly, positive correlations between lesion volume and real tissue temperature did not appear at the peak electrode-tip temperature.
For monitoring internal tissue temperatures, Thiagalingam and coworkers designed an intramural needle ablation catheter with an internally cooled 1.1-mm diameter straight needle that could be advanced up to 12 mm into the myocardium. The catheter could create significantly deeper and more transmural ablation lesions than a conventional irrigated-tip catheter (5-mm electrode; ThermoCool D curve system, Biosense Webster; Table 3.2 ).
Standard Catheter | Irrigated Catheter | Large-Tip Catheter | |
---|---|---|---|
Electrode length | 4–5 mm | 3.5–4 mm | 6–10 mm |
Power delivery | Up to 50 W | Up to 50 W | Up to 150 W |
Power titration | Temperature control | Power control monitoring temperature and impedance | Usually temperature control monitoring impedance ± microbubble formation |
Lesion size limited by | Electrode temperature and impedance rise | Power setting | Electrode temperature, current shunting, impedance rise |
Coagulum risk | Present | Low (especially open irrigation) | Present (possibly highest) |
Steam pop risk | Low | Present | Present |
Typical uses | Atrioventricular node reentry Accessory pathways Atrial tachycardias Ventricular tachycardia with normal heart Atrioventricular junctional ablation | Ventricular tachycardias with structural heart disease Atrial flutter Atrial fibrillation Coronary sinus ablation Epicardial ablation | Atrial flutter Possibly atrial fibrillation Possibly epicardial ablation |
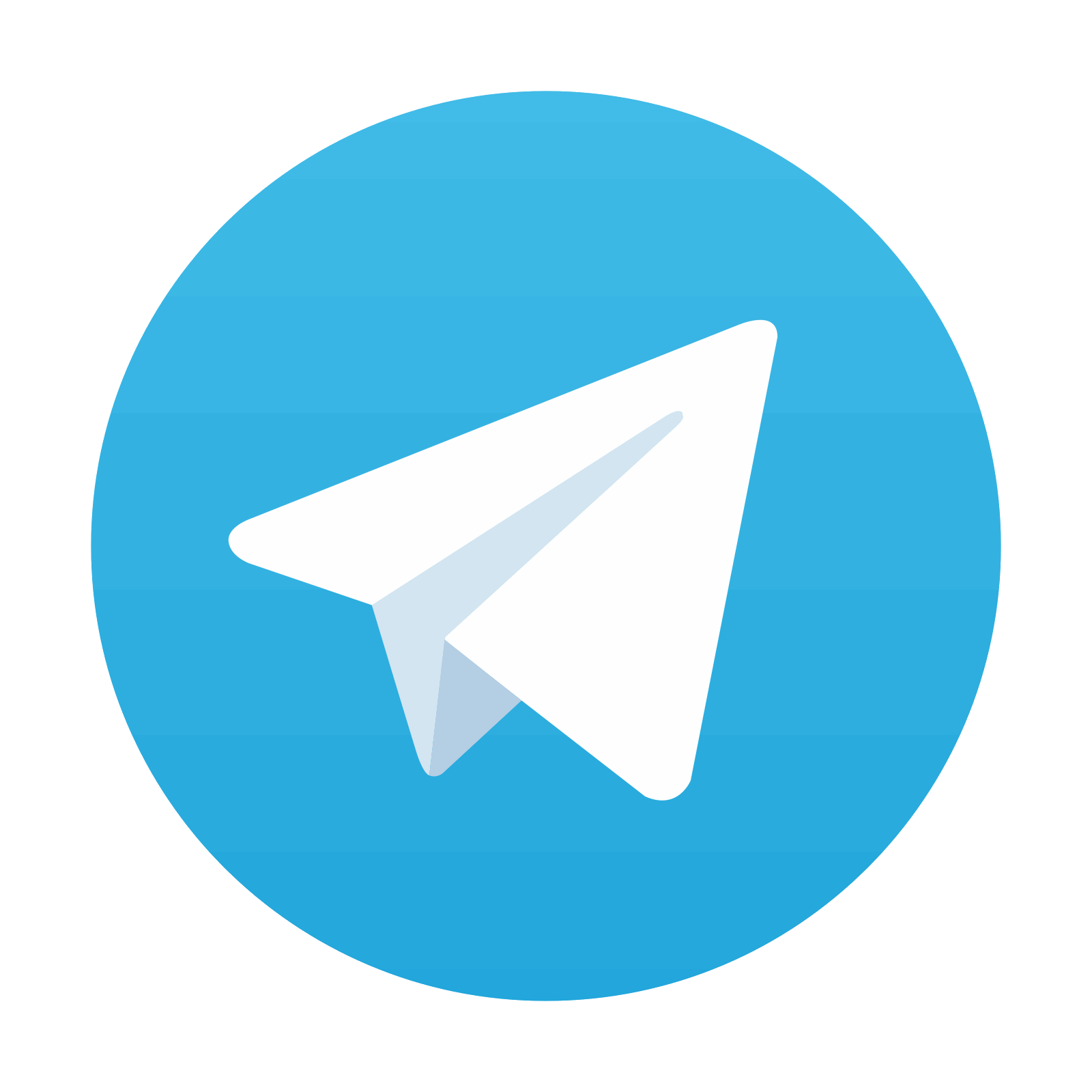
Stay updated, free articles. Join our Telegram channel

Full access? Get Clinical Tree
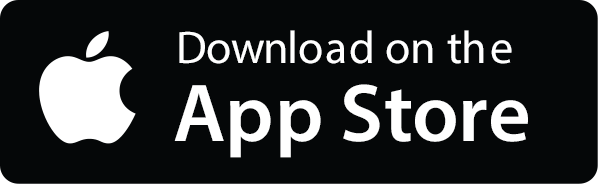
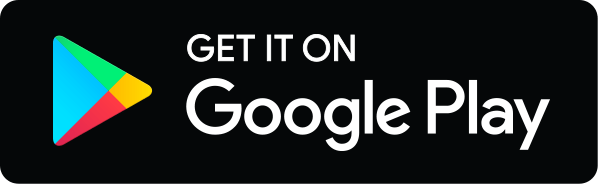
