Background
Left ventricular (LV) filling following atrial contraction generates LV myocardial stretch that propagates from base to apex with a speed proportional to myocardial elasticity. The aim of this study was to test the hypothesis that intrinsic velocity propagation of myocardial stretch (iVP) would be altered in patients with valvular disease and chronic LV pressure overload or volume overload, which may adversely affect mechanical properties of the LV tissue. A second aim was to compare iVP with flow propagation velocity in the chamber.
Methods
Sixty subjects were prospectively recruited: 20 with severe aortic stenosis (AS), 20 with severe degenerative mitral regurgitation (MR), and 20 normal control subjects. LV iVP was measured using ultrahigh–frame rate tissue Doppler (350–460 frames/sec) and flow propagation velocity by color flow M-mode imaging. Follow-up data (up to 2 years) were retrieved from medical records.
Results
iVP was highest in patients with AS (2.2 ± 0.7 m/sec), intermediate in those with MR (1.6 ± 0.5 m/sec), and lowest in control subjects (1.4 ± 0.2 m/sec; P < .0001). Fourteen patients with AS and eight with MR had iVP > 1.8 m/sec. Overall, iVP correlated with age, LV morphology, severity of aortic valve obstruction, and measures of LV preload and afterload. At follow-up, patients with high iVP had lower survival free of major adverse cardiac events ( P = .002). Flow propagation velocity was similar between groups and correlated poorly with iVP ( r = 0.26, P = .10).
Conclusions
A significant number of patients with severe AS and severe MR had rapid transmission of myocardial stretch, indicating increased myocardial stiffness. This information was not conveyed by measurement of flow propagation. Larger studies are needed to investigate the clinical utility of this novel measurement.
Highlights
- •
Noninvasive quantification of myocardial stiffness remains elusive.
- •
A novel approach to estimate myocardial elasticity is proposed.
- •
The mechanism relies on intrinsic cardiac waves and theory of wave propagation in elastic tubes.
- •
Some patients with valvular heart disease were found to have high diastolic myocardial stiffness.
- •
Intraventricular flow propagation was not able to convey this information.
Left ventricular (LV) filling generates longitudinal myocardial stretch first at the LV base, followed by the mid and apical levels. This progression can be thought of as a local disturbance propagating as a wave from base to apex. The time delay and wave speeds, both during early and late diastole, have been previously measured using strain rate imaging and proposed as evaluators of the diastolic function. However, the significance of these waves remains unknown.
More recently, our group has focused on the mechanism of the late diastolic stretch wave and shown it to be directly related to myocardial elasticity : the faster the wave speed, the higher the myocardial stiffness, as confirmed by an independent invasive method, the myocardial stress-strain relationship. In addition, it was shown that ultrahigh–frame rate Doppler tissue imaging (DTI), available on commercial scanners, is exquisitely sensitive for measuring this fast wave propagation in LV myocardium of healthy volunteers. This event occurs at a time when ventricular myocardium is quasi-relaxed and could be thought of as analogous to a mechanical vibration propagating along an elastic string in tension (i.e., the myocardial wall during diastole; Figure 1 ). The theory of wave propagation in a solid elastic medium predicts that wave speed is proportional to the elasticity of the medium. This concept is used in vascular biomechanics, in which pulse-wave velocity is a known measure of arterial stiffness. Thus, evaluation of intrinsic cardiac waves opens up a new approach to estimate myocardial stiffness noninvasively. This offers a great advantage because direct assessment of myocardial stiffness in patients has been elusive to achieve by noninvasive means and has been restricted to invasive pressure-volume analysis in the catheterization laboratory.

Almost nothing is known how these mechanical waves change with pathology. On the other hand, there are numerous studies addressing the mechanism of flow propagation in the chamber, particularly during early diastole, and showing that flow wave speed is reduced with impaired LV relaxation.
In this study, we evaluated patients with severe aortic stenosis (AS) and severe degenerative mitral regurgitation (MR) and compared them with normal subjects. These patients have chronic LV pressure overload or volume overload, respectively, resulting in concentric or eccentric LV hypertrophy, later progressing to subendocardial ischemia, necrosis and fibrosis, and culminating with overt LV dysfunction and heart failure. Considering these factors also change myocardial distensibility and stiffness, we hypothesized that some patients may have altered wave transmission in LV myocardium. A higher wave speed (and thus stiffness) would reflect the cumulative effects of the hemodynamic burden on the LV tissue, while a normal wave speed would indicate the presence of a compliant normal myocardium, despite the overloading condition.
The aims of this study were twofold. The first aim was to determine the range of values of this intrinsic velocity propagation of myocardial stretch (iVP) in the left ventricle in patients with severe AS and severe degenerative MR compared with normal subjects. The second aim was to evaluate whether there is a relationship between this wall-guided mechanical wave and flow wave propagation in the chamber.
Methods
The study was approved by the institutional review board, and written informed consent was obtained from each subject. The work complied with the Code of Ethics of the World Medical Association (Declaration of Helsinki) for experiments involving humans. Patients with severe AS (group 1) and those with severe degenerative MR (group 2) were prospectively recruited from patients referred for echocardiographic evaluation. Inclusion criteria were echocardiographically confirmed severe AS (typical restricted appearance of the aortic valve, with calculated aortic valve area < 1.0 cm 2 , mean gradient > 40 mm Hg, or peak aortic flow velocity > 4 m/sec) or severe degenerative MR (typical two-dimensional appearance of degenerative mitral valve disease, mitral effective regurgitant orifice > 0.4 cm 2 and regurgitant volume > 60 mL). Exclusion criteria were multivalvular disease (moderate or greater), infiltrative and hypertrophic cardiomyopathies, atrial or ventricular arrhythmias, complex congenital heart disease, moderate or greater LV dysfunction, and suboptimal echocardiographic image quality. All patients were in sinus rhythm. A control group (group 3) was selected from healthy volunteers or patients referred to the echocardiography laboratory and found to have normal LV size and function, no more than mild valvular disease, and no significant cardiovascular condition.
Echocardiography
All patients underwent comprehensive echocardiographic examinations as indicated per clinical practice. Data were acquired using commercial scanners (Vivid E9 [GE Healthcare, Little Chalfont, United Kingdom] or iE33 [Philips Healthcare, Best, The Netherlands]). Standard echocardiographic measurements were performed according to guidelines.
Global longitudinal systolic strain (GLS) was measured by speckle-tracking (50–60 frames/sec; Vivid E9 or Vivid Q) from three standard apical views (apical long-axis, four-chamber, and two-chamber).
Measures of LV Preload and Afterload
The ratio of early diastolic (E) mitral inflow velocity to average mitral annular e′ velocity was measured as an estimate of LV filling pressures (preload). Chamber stiffness was calculated as the ratio of E/e′ to LV end-diastolic volume. LV afterload was evaluated by total load (combined arterial and valvular load, equal to systolic blood pressure + mean aortic valve pressure gradient), valvuloarterial impedance ( Z va = total LV load/stroke volume index), and meridional end-systolic wall stress (0.334 PD / h [1 + h / D ], where P is pressure, D is chamber diameter, and h is wall thickness ).
Measurement of iVP
After the clinical echocardiographic examination, additional ultrahigh–frame rate DTI data (350–460 frames/sec) were acquired from three standard apical views using commercial scanners (Vivid E9 or Vivid Q). Each LV wall was scanned individually and carefully aligned with the ultrasound beams to minimize the Doppler angle. Data were analyzed offline (EchoPAC; GE Healthcare). Spatiotemporal maps of LV longitudinal tissue velocities during late diastole were reconstructed along a curved M-mode line passing through the wall and excluding the apical cap ( Figure 2 ). This cardiac phase is preferred because deformation during this phase depends mostly on tissue properties and loading, as opposed to early diastole, which is affected by concomitant LV relaxation, elastic recoil, untwisting, and filling, unpredictably interfering with a passive wave transmission. Velocity aliasing was used to facilitate identification of the onset of motion. All subjects showed a consistent wave front propagating from base to apex. The wave speed, called here iVP, was measured as the slope of the isovelocity wave front over a 3- to 5-cm segment. Values for two or three cardiac cycles were averaged, and a global value was obtained by averaging all walls. Strain rates were reconstructed to confirm the findings by DTI and rule out tethering effects (however, strain rate data were used as a quality test whenever possible, as these data were frequently noisier and unreliable for measuring wave propagation).

Elastic modulus was estimated from wave speed and LV geometry using the Moens-Korteweg equation as validated in animal studies : <SPAN role=presentation tabIndex=0 id=MathJax-Element-1-Frame class=MathJax style="POSITION: relative" data-mathml='c=Eh/ρD’>c=Eh/ρD−−−−−−√c=Eh/ρD
c = E h / ρ D
, where c is wave speed; h and D are wall thickness and diameter at end-diastole, respectively; and ρ is tissue density (1,060 kg/m 3 ).
Measurement of Flow Propagation Velocity
Color flow M-mode images were acquired in apical four-chamber view at 50 to 100 mm/sec sweep speed. An M-mode line was placed along the main direction of inflow as identified on two-dimensional color Doppler. Flow propagation velocity (FPV) was measured offline during early diastole and late diastole (FPV a ) from the slope of the isovelocity wave front, starting at the mitral leaflets to about 3 to 4 cm into the chamber. Values for two or three heart cycles were averaged. Baseline velocity was shifted to optimize the detection of flow propagation. FPV a was directly compared with myocardial iVP ( Figure 2 ).
Follow-Up
The presence or absence of valvular replacement or repair, functional status (LV ejection fraction [LVEF]) after intervention or with conservative treatment, and major adverse clinical events were obtained from available medical records. A combined clinical end point was created on the basis of the following adverse events: death, cardiac hospitalization, myocardial infarction, atrial fibrillation, and stroke.
Statistical Analysis
Analysis was performed using JMP version 10.0 (SAS Institute, Cary, NC). Normal distribution was verified using the Shapiro-Wilk statistic. Differences between groups were evaluated using analysis of variance. Pairwise comparisons were made with the Tukey’s t test. Univariate regression analysis was performed to evaluate the associations between iVP and relevant parameters. Multivariate regression analysis was subsequently used to identify the independent effects. Receiver operating characteristic analysis was used to differentiate patients with AS or MR from control subjects. Intra- and interobserver variability and agreement of iVP measurements were evaluated on 16 randomly selected data sets using Bland-Altman analysis. A test-retest analysis was performed on data from an additional 12 subjects scanned twice on two separate days. The impact on outcome was analyzed using Kaplan-Meyer and correlative analyses. Because this pilot study was not powered to evaluate the prognostic impact on patient outcomes, a limited statistical analysis was attempted for this purpose. Values are presented as mean ± SD or as number (percentage). Statistical significance was considered at P < .05.
Results
Sixty subjects were included in this pilot study: 20 patients with severe AS, 20 patients with severe degenerative MR, and 20 normal control subjects. Baseline characteristics are presented in Table 1 . Patients with AS and MR had typical morphologic and hemodynamic changes consistent with the presence of LV pressure overload or volume overload, respectively ( Table 1 ). Patients were on average older than control subjects; however, there were no differences in LVEF, cardiac output, and cardiac index. Most patients had normal LVEFs, except one patient with AS and three with MR who had moderately reduced LVEFs (<50% and <60%, respectively).
Variable | Patients with AS | Patients with MR | Control subjects |
---|---|---|---|
Age (y) | 73 ± 11 ∗ | 64 ± 12 ∗ | 58 ± 14 |
Men/women | 12/8 | 14/6 | 9/11 |
Heart rate (beats/min) | 64 ± 11 | 64 ± 13 | 61 ± 12 |
Systolic blood pressure (mm Hg) | 134 ± 18 ∗ | 125 ± 18 | 118 ± 14 |
Diastolic blood pressure (mm Hg) | 70 ± 12 | 71 ± 8 | 70 ± 11 |
NT-proBNP (pg/mL) | 855 ± 1,518 (410) | 943 ± 2,024 (218) | — |
Hypertension | 14 (70%) | 3 (15%) | 2 (10%) |
Coronary artery disease | 4 (20%) | 4 (20%) | 0 |
QRS duration (msec) | 103 ± 20 | 99 ± 12 | 89 ± 9 |
Bundle branch block | 5 (25%) | 1 (5%) | — |
β-blockers | 13 (65%) | 4 (20%) | 2 (10%) |
ACE inhibitors/ARBs | 8 (40%) | 4 (20%) | 1 (5%) |
Calcium channel blockers | 4 (20%) | 1 (5%) | — |
NYHA class I/II/II/IV | 3/8/7/2 | 6/9/4/1 | — |
Echocardiography | |||
LVEF (%) | 67 ± 6 | 65 ± 7 | 64 ± 5 |
Stroke volume index (mL/m 2 ) | 50 ± 13 | 47 ± 11 | 46 ± 10 |
Cardiac index (L/min/m 2 ) | 3.2 ± 1.1 | 2.9 ± 0.5 | 2.8 ± 0.5 |
Aortic valve area (cm 2 ) | 0.9 ± 0.2 ∗ † | 3.5 ± 0.8 | 3.4 ± 0.7 |
Mean transaortic pressure gradient (mm Hg) | 52 ± 14 | — | — |
Effective mitral regurgitant orifice (PISA) (cm 2 ) | — | 0.51 ± 0.21 | — |
Mitral regurgitant volume (mL) | — | 80 ± 26 | — |
LV mean wall thickness (mm) | 11.7 ± 1.4 ∗ † | 9.4 ± 1.1 | 9.4 ± 1.2 |
LV end-diastolic diameter (mm) | 48 ± 5 | 59 ± 4 ∗ | 47 ± 4 |
LVEDV (mL) | 111 ± 35 | 172 ± 39 ∗ | 113 ± 26 |
LV mass index (g/m 2 ) | 111 ± 30 ∗ | 116 ± 17 ∗ | 79 ± 21 |
LV geometry (normal/CR/EH/CH) | 4/5/3/8 | 7/0/13/0 | 16/2/2/0 |
GLS (%) | −18.2 ± 2.2 ∗ † | −20.5 ± 2.1 | −20.6 ± 1.3 |
Diastolic function | |||
Mitral E velocity (m/sec) | 0.8 ± 0.2 † | 1.1 ± 0.3 ∗ | 0.7 ± 0.1 |
Mitral A velocity (m/sec) | 1.0 ± 0.3 ∗ † | 0.7 ± 0.2 | 0.6 ± 0.1 |
Mitral E-wave deceleration time (msec) | 272 ± 74 ∗ † | 189 ± 57 | 207 ± 33 |
Mitral annular septal e′ velocity (cm/sec) | 5.3 ± 1.2 ∗ † | 7.8 ± 2.5 | 7.6 ± 2.3 |
E/e′ ratio (average) | 15.1 ± 5.2 ∗ | 13.7 ± 5.3 ∗ | 7.8 ± 1.7 |
(E/e′)/LVEDV (mL −1 ) | 0.15 ± 0.07 ∗ † | 0.09 ± 0.05 ∗ | 0.07 ± 0.03 |
Left atrial volume index (mL/m 2 ) | 41 ± 11 ∗ | 55 ± 28 ∗ | 28 ± 6 |
Right ventricular systolic pressure (mm Hg) | 33 ± 5 ∗ | 33 ± 12 ∗ | 24 ± 2 |
FPV, early diastole (m/sec) | 0.54 ± 0.17 | 0.51 ± 0.18 | 0.43 ± 0.15 |
FPV, late diastole (m/sec) | 0.50 ± 0.14 | 0.43 ± 0.16 | 0.53 ± 0.14 |
Pulmonary vein atrial flow reversal velocity (m/sec) | 0.27 ± 0.05 | 0.25 ± 0.08 | — |
LV afterload | |||
Total load (mm Hg) | 184 ± 21 ∗ | 125 ± 18 | 120 ± 15 |
Valvuloarterial impedance (mm Hg/mL/m 2 ) | 3.9 ± 0.9 ∗ † | 2.8 ± 0.8 ∗ | 2.7 ± 0.7 |
End-systolic wall stress (kdyne/cm 2 ) | 157 ± 23 ∗ † | 93 ± 16 ∗ | 72 ± 14 |
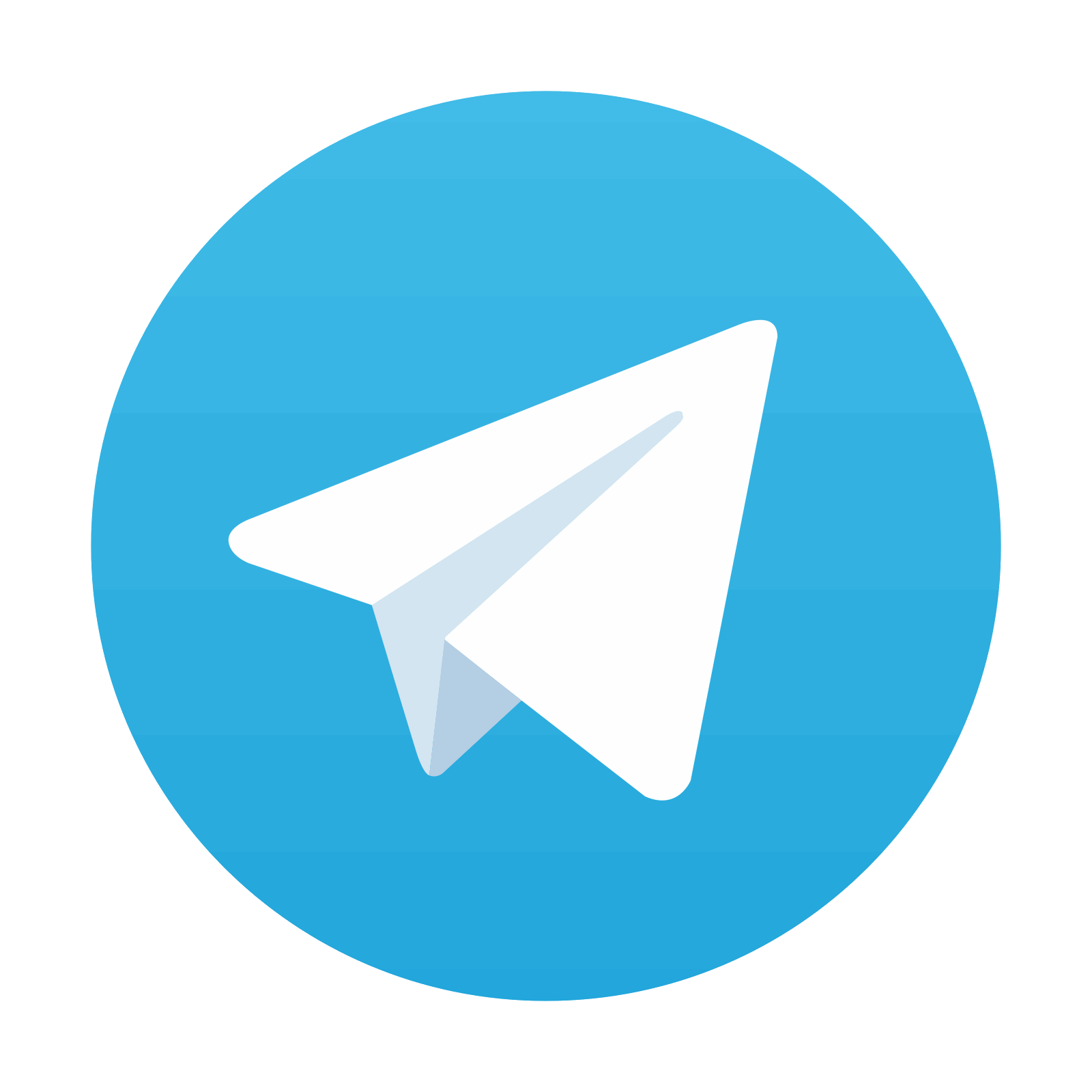
Stay updated, free articles. Join our Telegram channel

Full access? Get Clinical Tree
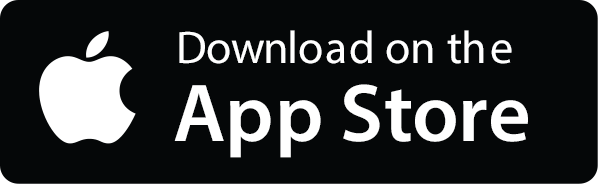
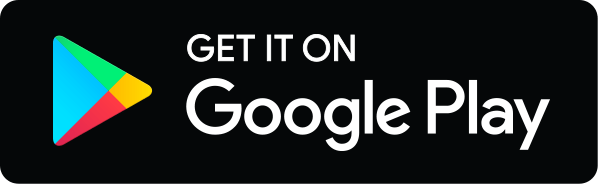
