Intraventricular Conduction Disturbances
Intraventricular conduction disturbances are the result of abnormal activation of the ventricles. Normal ventricular activation requires the synchronized participation of the distal components of the atrioventricular (A-V) conducting system, that is, the main bundle branches and their ramifications. In addition, abnormalities of local myocardial activation can further alter the specific pattern of activation in that ventricle. In the last chapter, I discussed the entire infra-His system as a unit as a site of prolonged, intermittent, or failed conduction. In this chapter, I address the consequences of impaired conduction in the individual fascicles. I will not detail the influences of pathologic processes, such as infarction, on ventricular activation. However, I will discuss the effects of infarction on the characteristic patterns of “bundle branch block.”
Because it is often difficult, if not impossible, to measure conduction times and refractory periods directly from the individual fascicles via catheter, identification of specific conduction defects usually depends on recognition of an altered pattern of ventricular activation on the surface ECG. Measurement of activation times at different areas of the ventricles has been suggested as a means of indirectly assessing conduction properties of the right bundle branch (RBB) and the multiple divisions of the left bundle branch (LBB). This technique may be useful in evaluating conduction over the RBB, particularly if simultaneous measurements from the LBB are also obtained from the catheter and/or if the entire pattern of right ventricular activation is determined intraoperatively. It is possible because the anatomy of the RBB is generally uniform. In humans the RBB has its major route from the His bundle through the musculature of the ventricular septum. The septal band gives rise to the moderator band, which extends to the anterior papillary muscle at the apical third of the right ventricular free wall. Purkinje fibers extend from the moderator band to the remainder of the RV. In Tawara’s text a branch from the RBB in the moderator band gives rise to Purkinje fibers that subsequently activate the septum (Fig. 5-1). In some patients there appears to be a small continuation of the RBB distal to the moderator band toward the apical septum, which is also supplied by extensions of the moderator band and Purkinje fibers. Mapping studies of the RBB by Durrer et al.1 and Horowitz et al. showed that most individuals have the moderator band as the main source of right ventricular activation. Durrer et al.1 also noted that one in four had relatively early activation of the right ventricular apical septum, but activation of the septum never occurred prior to activation of the right ventricular free wall.
However, there are many objections to its use in evaluating the LBB system. The major objection stems from the fact that the LBB does not divide into two discrete fascicles, but it divides into two or three broad fascicle, then rapidly fans out over the entire left ventricle. The size of the fascicles is highly variable as described by Tawara2 more than 100 years ago (Figs. 5-2 and 5-3). Thus, a detailed assessment of infra-His conduction in humans can be made only intraoperatively, where direct mapping of the entire subendocardial conducting system can be performed. Use of mathematically derived electrograms from an intracoronary probe (Endocardial Solutions, Inc.) may provide assessment of His–Purkinje activation, particularly on the septum, but the use of this probe is complicated and expensive. Moreover, the value of data obtained from this probe has not been assessed. Therefore, for practical reasons, clinical evaluation has primarily involved observations of the surface ECG pattern and on the H-V interval. Recordings of potentials of the RBB and/or from the proximal and distal His bundle are of great help in determining sites of conduction delay or block associated with these specific electrocardiographic patterns and should be performed whenever possible.
Traditionally, in clinical electrocardiography, three major fascicles are considered to be operative in normal persons, even though a third fascicle of the LBB (septal fascicle) is usually responsible for the initiation of intraventricular septal depolarization (see Fig. 2-18).3 Electrocardiographically the three major fascicles are (a) the RBB, which is an anatomically compact unit that travels as the extension of the His bundle after the origin of the LBB; (b) the anterior (superior) division of the left bundle branch block (LBBB); and (c) the posterior (inferior) division of the LBB. The LBB and its divisions are, unlike the RBB, diffuse structures that fan out just beyond their origin (some investigators have denied their distinct anatomic existence).4,5 Nevertheless, specific patterns of altered ventricular activation as reflected in the QRS complex have been correlated experimentally, clinically, and anatomically with altered conduction in each of the fascicles. The contribution of the septal fascicle to the ECG is less well appreciated but is responsible for the initial left to right septal forces giving rise to a small r wave in lead V1 and a small q wave in lead I and/or aVL.
Definitions
Right bundle branch block (RBBB). QRS interval prolongation >120 msec with normal activation during the first half (approximately 60 msec) of the QRS complex, delayed intrinsicoid deflection in lead V1, and abnormal anterior and rightward forces during the terminal portion of the QRS complex.
Left bundle branch block. QRS interval prolongation >120 msec or (usually >140 msec) delayed intrinsicoid deflection in lead V6 (>0.075 seconds); absent Q waves and slurred broad R waves in leads I, aVL, and V6; rS or QS deflection in leads V1 and V2 (and often to V4); and an ST- and T-wave vector 180 degrees discordant to the QRS vector.
Left anterior hemiblock. QRS interval of 100 msec or shorter (unless complicated by bundle branch block, hypertrophy, etc.), with a frontal plane QRS axis to the left of −30 degrees with a counterclockwise loop, and initial activation in a rightward and inferior direction, with a small q wave in lead aVL and a small r wave in lead III, followed by leftward and superior forces with tall R waves in lead I and aVL and deep S waves in leads II, III, and aVF.
Left posterior hemiblock. Right ventricular hypertrophy, chronic lung disease, and extensive lateral wall myocardial infarction must be excluded. QRS interval of 100 msec or shorter (unless complicated by RBBB), a frontal plane QRS axis to the right of +110 degrees, with a clockwise loop, a small initial r wave in lead aVL, a small q wave in lead aVF, and a subsequently large terminal S wave in lead aVL and an R wave in aVF.
Intraventricular conduction defect (IVCD). QRS interval prolongation >120 msec with a pattern not conforming to LBBB or RBBB. An intraventricular conduction delay may be further classified as left or right ventricular, depending on the site of delayed intrinsicoid deflection and the direction of the terminal QRS forces.
Although the term bundle branch block is standard nomenclature, the pathophysiology of this electrocardiographic pattern should be thought of in terms of relative conduction delay (of varying degree and including failure of conduction) producing asynchronous ventricular activation without necessarily implying complete transmission failure. Thus, a typical bundle branch block appearance may be due to marked conduction delay in the bundle branch and not failure of conduction. The point is demonstrated by (a) varying degrees of “complete” bundle branch or fascicular block manifested by further axis shift or QRS widening either spontaneously or following premature extrastimuli6 and (b) spontaneous or induced alternating “complete” bundle branch block (Figs. 5-1 and 5-2).7 Obviously, in the latter instance, one could not have complete block in one bundle branch and then develop complete block in the other bundle branch without total failure of A-V conduction.
The marked H-V prolongation during LBBB in Figure 5-1 and during RBBB in Figure 5-2 reflects slowed conduction in the contralateral bundle branch to the one demonstrating “block” on the surface electrocardiogram. The difference in conduction time in the bundle branches allows the ventricles to be activated asynchronously, producing the typical QRS patterns of bundle branch block. Thus when alternating bundle branch “block” is observed, one assumes that the bundle branch block pattern associated with the long H-V is blocked, while the contralateral bundle is slowly conducting.
Site of “Block” or Conduction Delay During Bundle Branch Block
Interest in the site of block and/or conduction delay in the fascicles stems from the recognition that bifascicular block, especially RBBB with left anterior hemiblock, is the most common ECG pattern preceding the development of A-V block. Because these ECG patterns are common, we need to elucidate factors that can predict who will develop A-V block. Determining the site of bundle branch block is the first step in this process.
Despite the ECG-anatomic correlates that have been made, the exact site of block or conduction delay producing bundle branch block patterns is not certain in all cases. Longitudinal dissociation with asynchronous conduction in the His bundle may give rise to abnormal patterns of ventricular activation;8 hence, the conduction problem may not necessarily lie in the individual bundle branch. This phenomenon has been substantiated in the case of both LBBB and left anterior hemiblock by normalization of the QRS complex, H-V interval, and ventricular activation times during His bundle stimulation (Fig. 5-3).9,10 These findings suggest that fibers to the left and right ventricles are already predestined within the His bundle and that lesions in the His bundle may produce characteristic bundle branch block patterns. Moreover, it is not uncommon to observe intra-His disease (widened or split
His potentials) accompanying bundle branch block, particularly LBBB.
His potentials) accompanying bundle branch block, particularly LBBB.
The frequency with which conduction disturbances in the His bundle are responsible for the fascicular and bundle branch blocks is not known. The use of multipolar catheters to record distal, mid-, and proximal His bundle potentials or proximal right bundle and proximal His bundle potentials are of great use in delineating how frequently very proximal lesions result in a particular bundle branch block. It is theoretically appealing to postulate that such longitudinal dissociation in the His bundle causes the conduction abnormalities that ultimately result in complete A-V block in either the setting of acute anteroseptal infarction or sclerodegenerative diseases of the conducting system. The sudden simultaneous failure of conduction through all peripheral fascicles would appear much less likely than failure at a proximal site in the His bundle or at the truncal bifurcation. An example supporting this concept is shown in Figure 5-4, in which atrial pacing in a patient with LBBB and split His potentials produces progressive prolongation of conduction and ultimate block between the two His potentials, that is, in the His bundle.
The site of transient bundle branch block may differ from that of chronic or permanent bundle branch block. Studies in the clinical electrophysiology laboratory in which distal His bundle or proximal RBB recordings are used along with right and left ventricular endocardial mapping and/or intraoperative studies have both proved useful in better defining the sites of block in patients with either chronic or transient bundle branch block (i.e., aberration). Because the likelihood of developing complete A-V block may depend on the site of conduction or block in individual fascicles, obtaining such data is critical to predicting risk of A-V block. Failure to do so may explain variability of published data in predicting progression of bifascicular block to complete A-V block. (See section on predicting risk of heart block.) Results of activation studies during bundle branch block are described in the following paragraphs.
Chronic Right Bundle Branch Block
Intraoperative studies have clearly shown that the electrocardiographic pattern of RBBB can result from lesions at different levels of the conducting system in the right ventricle.11,12 These studies were performed during operative procedures for congenital heart disease, the most common of which was repair of tetralogy of Fallot. Endocardial mapping was performed from the His bundle along the length of the RBB, including the base of the moderator band, where it separated into the septal divisions, the anterior free wall, and the outflow tract. Epicardial mapping was also performed. The use of both endocardial and epicardial mapping clearly demonstrated three potential levels of block in the right ventricular conduction system that could lead to the electrocardiographic pattern of RBBB: (a) Proximal RBBB, (b) distal RBBB at the level of the moderator band, and (c) terminal RBBB involving the distal conducting system of the RBB or, more likely, the muscle itself.12
Proximal RBBB was the most common form noted after transatrial repair of tetralogy of Fallot,11,12 and similar studies in adults suggest it is also the most common spontaneously occurring type of chronic RBBB.13,14,15 In proximal RBBB, loss of the right bundle potential occurs where it is typically recorded (Fig. 5-5). Activation at these right ventricular septal sites is via transseptal spread from the left ventricle. This results in
delayed activation of the mid- and apical right ventricular septum, the right ventricular anterior wall at the insertion of the moderator band, and the right ventricular outflow tract (RVOT) (Fig. 5-6). Epicardial mapping in patients preoperatively and postoperatively shows a change from the normal right ventricular breakthrough at the midanterior wall with concentric spread thereafter, to a pattern showing no distinct right ventricular breakthrough but right ventricular activation occurs via transseptal spread following left ventricular activation. In Figure 5-7, this transseptal spread begins at the apex and then sequentially activates the midanterior wall and base of the heart. Left ventricular activation remains normal. In cases of proximal RBBB, the mid- and apical septa are activated at least 30 msec after the onset of the QRS.
delayed activation of the mid- and apical right ventricular septum, the right ventricular anterior wall at the insertion of the moderator band, and the right ventricular outflow tract (RVOT) (Fig. 5-6). Epicardial mapping in patients preoperatively and postoperatively shows a change from the normal right ventricular breakthrough at the midanterior wall with concentric spread thereafter, to a pattern showing no distinct right ventricular breakthrough but right ventricular activation occurs via transseptal spread following left ventricular activation. In Figure 5-7, this transseptal spread begins at the apex and then sequentially activates the midanterior wall and base of the heart. Left ventricular activation remains normal. In cases of proximal RBBB, the mid- and apical septa are activated at least 30 msec after the onset of the QRS.
![]() FIGURE 5-6 Selected epicardial electrograms in proximal right bundle branch block. ECG leads 1, 2, and 3 are shown with a reference electrogram recorded in the left ventricle (LV) and mapping electrograms recorded at the right ventricular apex (RVA), right ventricular anterior wall (RVAW), and right ventricular outflow tract (RVOT) before and after transatrial repair of tetralogy of Fallot. The vertical lines indicate the onset of the QRS, and the numbers indicate time (in milliseconds) from the vertical line to the local electrogram. Before repair, the RVAW was the earliest site in the right ventricle. After repair, the earliest right ventricular site was the apex, which was activated 25 msec later than before repair. The latest ventricular epicardial activation occurred on the RVOT at 144 msec (cf. Fig. 5-7). (From Horowitz LN, Alexander JA, Edmunds LH Jr. Postoperative right bundle branch block: identification of three levels of block. Circulation 1980;62:319.) |
In distal RBBB, activation of the His bundle and proximal RBB is normal. RBB potentials persist at the base of the moderator band and are absent at the midanterior wall, where the moderator band normally inserts (Fig. 5-8). This form of bundle branch block only occurred when the moderator band was cut during surgery. Thus, this form of RBBB is extremely rare as a spontaneous form of bundle branch block. In distal RBBB, the apical and midsepta are normally activated, but activation of the free wall at the level of the moderator band and subsequent activation of the RVOT is delayed (Figs. 5-9 and 5-10). Epicardial activation shows a small area of apical right ventricular activation that is the same as preoperatively. Activation at the midanterior wall, which was the site of epicardial breakthrough in the right ventricle preoperatively, was delayed, as was subsequent activation of the remaining right ventricle (Fig. 5-10).
Terminal RBBB was the second most common form of RBBB observed following either transatrial or transventricular
repair of tetralogy of Fallot. Activation along the specialized conducting system (i.e., His bundle branch and RBB) remains normal up to the Purkinje–myocardial junction (Fig. 5-11). In contrast to proximal and distal RBBB, activation of the midanterior wall is unchanged from normal, and only the RVOT shows delayed activation. Epicardial mapping demonstrates slowly inscribed isochrones from the infundibulum to the base of the heart (Figs. 5-12 and 5-13). Of note, terminal RBBB, which is probably due to interruption of the terminal Purkinje network and/or intramyocardial delay can have two quantitatively similar but qualitatively different patterns.12
repair of tetralogy of Fallot. Activation along the specialized conducting system (i.e., His bundle branch and RBB) remains normal up to the Purkinje–myocardial junction (Fig. 5-11). In contrast to proximal and distal RBBB, activation of the midanterior wall is unchanged from normal, and only the RVOT shows delayed activation. Epicardial mapping demonstrates slowly inscribed isochrones from the infundibulum to the base of the heart (Figs. 5-12 and 5-13). Of note, terminal RBBB, which is probably due to interruption of the terminal Purkinje network and/or intramyocardial delay can have two quantitatively similar but qualitatively different patterns.12
During terminal bundle branch block produced by ventriculotomy, the latest activation is adjacent to the ventriculotomy scar. In contrast, when terminal bundle branch block is produced by transatrial resection, then the delayed activation appears as a smooth homogenous slow spread from the anterior infundibulum to the posterobasal aspects of the outflow tract. These data help resolve previously reported experimental work and clinical studies by a variety of authors.16,17,18,19,20,21 Our findings were similar to those of Wyndham,13 Wyndham et al.,14 and Van Dam.15
Clinically, these findings11,12,13,14,15 are relevant because terminal or distal bundle branch block, when accompanied by disorders of left ventricular conduction, may not indicate an increased risk of heart block. Such distal or terminal block has been seen in atrial septal defect, where conduction in the RBB is normal but stretching of terminal Purkinje fibers and/or muscle causes delayed activation of the RVOT.19 I have also seen terminal bundle branch block in patients with cardiomyopathy and chronic lung disease. Finally, I have also seen this pattern in rare patients with right ventricular infarction associated with the development of an RBBB pattern. In this latter case the duration of the QRS does not exceed 120 msec. In my
opinion, these data favor a delay in intramyocardial conduction as the primary cause of terminal conduction delay. Determination of either proximal or terminal bundle branch block can easily be made in the clinical electrophysiology laboratory by demonstrating normal activation time of the mid- and apical septa in the presence of RBBB. If RBBB is proximal, activation of the mid- and apical septa will be delayed, producing a V (onset of the QRS) to local RV activation time exceeding 30 msec.22,23 If the RBBB is the rare distal type or, more commonly, the terminal RBBB, the local activation time at the mid- and apical septa will be normal (i.e., <30 msec), whereas that of the anterior wall (in the case of distal RBBB) and/or the outflow tract (either distal or terminal RBBB) will be delayed. Electroanatomic mapping allows one to display right and/or left ventricular activation during bundle branch block. An example of RBBB due to proximal RBBB and passive RV activation by transseptal spread from the left ventricle is shown in Figure 5-14.
opinion, these data favor a delay in intramyocardial conduction as the primary cause of terminal conduction delay. Determination of either proximal or terminal bundle branch block can easily be made in the clinical electrophysiology laboratory by demonstrating normal activation time of the mid- and apical septa in the presence of RBBB. If RBBB is proximal, activation of the mid- and apical septa will be delayed, producing a V (onset of the QRS) to local RV activation time exceeding 30 msec.22,23 If the RBBB is the rare distal type or, more commonly, the terminal RBBB, the local activation time at the mid- and apical septa will be normal (i.e., <30 msec), whereas that of the anterior wall (in the case of distal RBBB) and/or the outflow tract (either distal or terminal RBBB) will be delayed. Electroanatomic mapping allows one to display right and/or left ventricular activation during bundle branch block. An example of RBBB due to proximal RBBB and passive RV activation by transseptal spread from the left ventricle is shown in Figure 5-14.
![]() FIGURE 5-9 Selected epicardial electrograms in distal right bundle branch block. ECG leads 1, 2, and 3 are shown with a reference electrogram recorded in the left ventricle (LV) and mapping electrograms recorded at the right ventricular apex (RVA), right ventricular anterior wall (RVAW), and right ventricle outflow tract (RVOT) before and after transatrial repair of tetralogy of Fallot. The vertical lines indicate the onset of the QRS, and numbers indicate time (in milliseconds) from the vertical line to the local electrograms. Before repair, the RVAW was the earliest right ventricular site. After repair, right bundle branch block (RBBB) was present, and the earliest activation was at the RVA. Despite the presence of RBBB, the RVA activation time after repair did not significantly differ from the value before repair. Activation of the RVAW and RVOT were delayed (cf. Fig. 5-10). (From Horowitz LN, Alexander JA, Edmunds LH Jr. Postoperative right bundle branch block: identification of three levels of block. Circulation 1980;62:319.) |
Left Bundle Branch Block
Far fewer data are available to evaluate the site of conduction abnormalities in LBBB. No human studies have traced conduction from the His bundle down the left bundle system, as done in RBBB.11,12 Epicardial mapping data in a few patients with LBBB13,14,15 demonstrate (a) that right ventricular activation is normal but occurs relatively earlier in the QRS; (b) that a discrete left ventricular breakthrough site is absent, in contrast to normal, in which two or three breakthrough sites may be observed; (c) that transseptal conduction is slow, as manifested by crowded isochrones in the interventricular sulcus with more rapid isochrones along the left ventricular free wall. In LBBB with normal axis, Wyndham13 found that the latest left ventricular site activated was not the A-V sulcus, as it is with normal intraventricular conduction. These studies, however, not only were limited by the small number of patients but by the fact that the authors did not consider myocardial disease and did not adequately address the effect of axis deviation.
Furthermore, the extent to which intramural conduction delay contributed to QRS widening has never been assessed.
Furthermore, the extent to which intramural conduction delay contributed to QRS widening has never been assessed.
We therefore decided to evaluate endocardial activation during LBBB in a heterogenous group of patients, including four with no organic heart disease, six with congestive cardiomyopathy, and eight with coronary artery disease and previous infarction.24 All but one patient had a QRS >140 msec. Only three patients had normal H-V times. Unfortunately, we did not record right bundle potentials or distal His potentials to localize the source of H-V prolongation (see the following discussion).
We performed catheter mapping studies, as described in Chapter 2. We recorded standard activation sites (Fig. 5-15) in the right and left ventricle in sinus rhythm with fixed and variable-gain electrograms using both a 1-cm and 5-mm interelectrode bipolar recording. There was no difference in activation times between them. We therefore defined local activation as the point on the 1-cm variable-gain electrogram at which the largest rapid deflection crossed the baseline. When a fractionated electrogram was present without a surface discrete deflection >1 mV in amplitude, we used the rapid deflection of highest amplitude as local activation time. In addition, we measured the onset and offset of local activation from the fixed-gain electrogram from the time the electrical signal reached 0.1 mV from baseline to the time of the amplifier decay signal (see Fig. 2-11). We defined transseptal conduction time as the difference between local activation time at the right ventricular septum (usually near the apex) and the earliest left ventricular activation time. We also evaluated the total left ventricular activation time, which was the difference in time from the earliest to the latest left ventricular endocardial activation. The average number of left ventricular sites mapped was 14 ± 3 per patient (range, 8 to 19). There was
no difference in a number of sites mapped among any of the three groups.
no difference in a number of sites mapped among any of the three groups.
![]() FIGURE 5-12 Selected epicardial electrograms in terminal right bundle branch block (RBBB). ECG leads 1, 2, and 3 are shown with a left ventricular (LV) reference electrogram and with mapping electrograms recorded at the right ventricular apex (RVA), right ventricular anterior wall (RVAW), and right ventricular outflow tract (RVOT) before and after transatrial repair of tetralogy of Fallot. The vertical lines indicate the beginning of the QRS, and the numbers indicate time (in milliseconds) from the vertical line to the local electrograms. Before repair, right ventricular activation was earliest in the RVAW and latest in the RVOT, 67 msec after the onset of the QRS. After repair, RBBB was present and RVAW and RVA activation were not changed, but activation of the RVOT was delayed 45 msec (cf. Fig. 5-13). (From Horowitz LN, Alexander JA, Edmunds LH Jr. Postoperative right bundle branch block: identification of three levels of block. Circulation 1980;62:319.) |
Twelve of 18 patients had only one site of a left ventricular endocardial breakthrough. In nine patients, this was in the middle third of the left ventricular septum, and in three patients, it was at the apical third of the septum. In the remaining six patients, we observed simultaneous early activation at two left ventricular sites; in two patients, two sites were on the septum (one in the middle third and one at the apical septum), and in one, the apical septum and superior basal free wall. In contrast to the studies of Wyndham,13 we found that the latest site of left ventricular activation was frequently at the base of the heart in patients with normal axis, while it was more variable in those with left axis (Table 5-1).24
Left ventricular endocardial activation began a mean of 52 ± 17 msec after the onset of the surface QRS (Table 5-2). We observed no difference in any of the three groups. In the normal patients, left ventricular activation began 44 ± 13 msec after the QRS, in patients with cardiomyopathy, left activation began 58 ± 13 msec after the onset of the QRS, and in the patients with prior infarction, left ventricular septal activation began at 51 ± 20 msec after the QRS.
The earliest activity in local anteroseptal sites was similar in the normal and cardiomyopathic groups, at 23 ± 9 and 23 ± 19 msec. However, earliest activation recorded in the septum in
patients with prior anteroseptal infarction was only 11 ± 11 msec and was significantly shorter than that reported in the other groups (p < 0.05).
patients with prior anteroseptal infarction was only 11 ± 11 msec and was significantly shorter than that reported in the other groups (p < 0.05).
TABLE 5-1 Relationship between QRS Axis and Activation Sequence | ||||||||||||||||||||||||||||||||||||||||||||||||||||||||||||||||||||||||||||||
---|---|---|---|---|---|---|---|---|---|---|---|---|---|---|---|---|---|---|---|---|---|---|---|---|---|---|---|---|---|---|---|---|---|---|---|---|---|---|---|---|---|---|---|---|---|---|---|---|---|---|---|---|---|---|---|---|---|---|---|---|---|---|---|---|---|---|---|---|---|---|---|---|---|---|---|---|---|---|
|
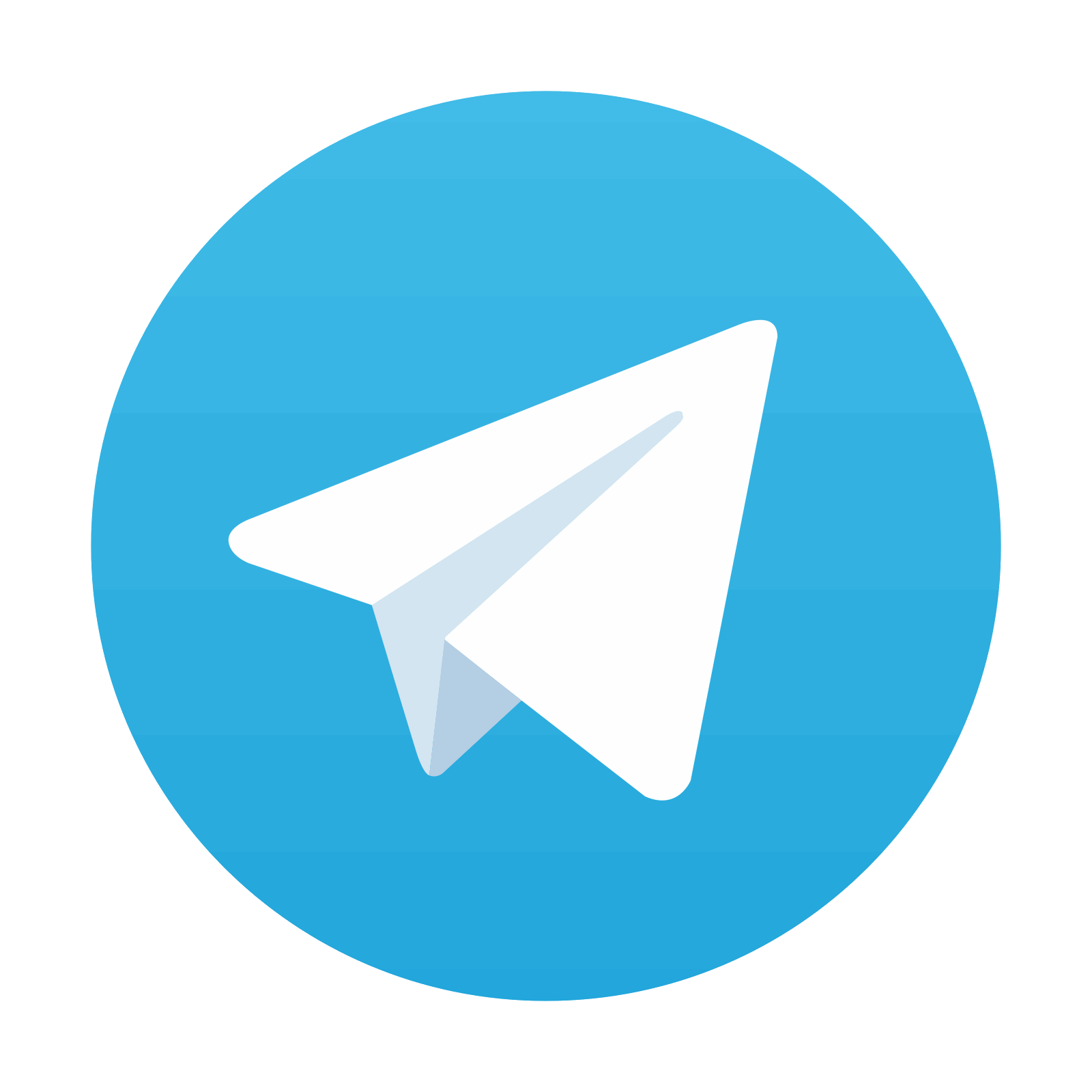
Stay updated, free articles. Join our Telegram channel

Full access? Get Clinical Tree
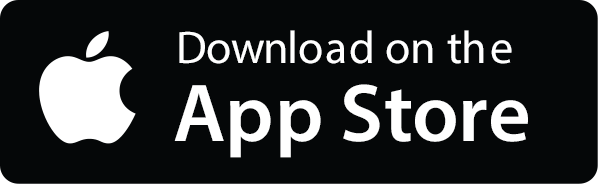
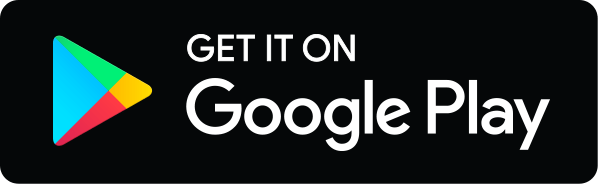