Cardiovasculara
Respiratorya
Cerebrala
Agent
HR
MAP
Vent
B’dil
CBF
CMRO2
ICP
Propofol
0/↓↓
↓↓↓
↓↓/↓↓↓
?
↓↓↓
↓↓↓
↓↓↓
Thiopental
↑↑/↑
↓↓/↓↓↓
↓↓/↓↓↓
↓
↓↓↓
↓↓↓
↓↓↓
Etomidate
0
0/↓
↓/↓↓
0
↓↓↓
↓↓↓
↓↓↓
Midazolam
↑
↓↓
↓↓/↓↓↓
0
↓↓
↓↓
↓↓
Ketamine
↑↑/↑
↑↑/↑
0
↑↑/↑
↑↑
↑↑
↑
Dexmedetomidine
↓
↓
0
0
0
0
Propofol induces a biphasic pattern of electroencephalogram (EEG) activation that slows with increasing doses. After the initial activation, EEG slowing is dose related and proceeds to burst suppression and then to complete electrical silence [9]. During induction, patients older than 70 years reach significantly deeper EEG stages than younger patients, need a longer time to reach the deepest EEG stage, and need more time until a light EEG stage is regained [10]. The EEG changes described above cause a shorter duration of seizure in the patient on electroconvulsive therapy (ECT) , but they also allow for a blunted hypertensive and hyperdynamic response that is often seen in these patients [11]. Although propofol often allows for seizures of a clinically acceptable duration [11], many psychiatrists prefer methohexital for ECT. Although methohexital does allow for longer seizure duration, it does not block the hypertensive response to ECT as much and thus is not as ideal in the geriatric patient who is likely to have cardiac disease.
The brain becomes more sensitive to propofol with increasing age. Schnider et al. [12] reported that geriatric patients were approximately 30% more sensitive to the PD effects of propofol than younger patients, as measured by EEG changes. This was found to be true for both induction doses and infusions. Thus, it seems that increasing age causes changes in the brain that increase the effective potency of propofol for the geriatric patient.
Respirator y Effects
Propofol causes dose-related depression of ventilation and is thought to produce some bronchodilation, although this is controversial (Table 17.1) [13, 14]. In standard induction doses, propofol causes apnea [15]. However, when compared with thiopental, respirations are lost later and recovered earlier [15]. With intravenous infusions for sedation, propofol causes increasing levels of respiratory depression, mainly by affecting the tidal volume. Furthermore, airway reflexes are depressed more so with propofol than with equivalent doses of thiopental or etomidate, and this effect is greatly enhanced by the addition of opioids [16]. Also, although propofol does not inhibit hypoxic pulmonary vasoconstriction, it does seem to blunt both the hypoxic and hypercapnic ventilatory responses [15, 17–19].
All of these changes described above have particular relevance for the elderly patient. Because of increases in closing capacity with increasing age, which will exceed functional residual capacity (FRC) even in the upright position in a 65-year-old individual, desaturation can occur at a faster rate. In the elderly, this occurs as a result of an increase in shunt fraction rather than a reduced FRC, as is seen with the obese individuals or in patients with restrictive lung disorders [20]. The elderly also have a decreased cough reflex and thus a decreased ability to clear secretions [21]. This inherently decreased cough reflex in the elderly patient combined with the suppression of this reflex from propofol puts the elderly person at higher risk for aspiration during its use. Furthermore, the elderly patient already has a blunted hypoxic and hypercapnic ventilatory response compared with the average adult patient [21]. These changes call for great vigilance when administering propofol to an elderly patient for minimal alveolar concentration (MAC) anesthesia or even light sedation. Ventilation should be closely monitored, particularly if supplemental oxygen is used, because the hypercapnic ventilatory response will become the primary regulator of respiration. This is important because supplemental oxygen could prevent hypoxemia, but allow for a progressive hypercapnia that could be dangerous to the patient. Finally, all of these effects are increased with the concurrent use of opioids, thus requiring even greater attention in operative and procedural situations when the elderly patient is maintaining oxygenation and ventilation through spontaneous respirations without a secure airway or end-tidal carbon dioxide monitoring. However, all of the evidence cited thus far would suggest that increased PD sensitivity in the elderly patient moves in a parallel manner for respiratory depression and sedation/hypnosis; that is, the patient is not fully awake and merely experiencing decreased respiratory drive. Thus, in the spontaneously ventilating patient, the gradual titration of propofol matched with a vigilance attentive to signs of adequate respiration and level of sedation should provide for a safe and effective anesthetic.
Cardiovascular Effects
Propofol causes little change in heart rate but can cause profound changes in MAP when given in induction bolus doses (Table 17.1) [22]. These changes are caused by a reduction in systemic vascular resistance (via inhibition of sympathetic vasoconstriction) and preload, as well as direct effects on myocardial contractility. This hypotension is more pronounced than what is seen with the administration of thiopental, etomidate, or midazolam. In the normal adult patient, this hypotension is well tolerated and it is readily reversed during the stimulation of laryngoscopy and intubation. However, studies have shown that the degree of hypotension is increased and an adequate hemodynamic response to a bolus induction is decreased in the geriatric patient. This occurs by several mechanisms. First, propofol impairs the arterial baroreceptor reflex to hypotension, which is already decreased in the geriatric patient [23]. Second, the geriatric patient is more likely to have ventricular dysfunction. A decrease in preload in these patients may result in a significant decrease in cardiac output. Third, these patients are often taking beta-blockers and diuretics or other therapies that cause hypovolemia in the perioperative period. The former reduces the magnitude of any baroreceptor-mediated reflex tachycardia to a decrease in blood pressure, whereas the latter tends to make the patient more sensitive to changes in systemic vascular resistance and preload secondary to being relatively intravascularly hypovolemic [23]. Finally, it is possible for a profound decrease in preload to result in a vagally mediated reflex bradycardia [24]. Practically speaking, these concerns can be clinically applied in two general categories. First, for the geriatric patient with significant cardiac disease, it is best to avoid a rapid bolus induction with propofol. Second, many of the untoward effects noted above can be greatly minimized if a slower infusion induction is performed with laryngoscopy being performed after reaching a PD endpoint, such as a bispectral index (BIS) value of less than 60 (see discussion in sections further) [25].
Other Effects
Two unique beneficial effects of propofol are noteworthy. Propofol has both antiemetic and antipruritic properties [26, 27]. Thus, its intraoperative and perioperative use has the potential to reduce the need for traditional antiemetic and possibly antipruritic medications in the postoperative period. This is particularly important in the geriatric patient who may be more susceptible to the untoward effects of drugs that work at cholinergic and dopaminergic sites in the normal treatment of nausea and pruritus [28].
Metabolism and Disposition (Pharmacokinetics)
The PK of propofol involves a very large volume of distribution, rapid redistribution, and rapid elimination via hepatic and extrahepatic routes (see Table 17.2). Owing to high lipid solubility, it has an onset of action of one arm-to-brain circulation time (almost as fast as thiopental). Rapid awakening from a single bolus is the result of extensive redistribution to non-CNS sites throughout the body. Its initial distribution half-life in a healthy adult patient is approximately 2 min [29–32].
Table 17.2
Pharmacokinetic parameters for commonly used intravenous nonopiates
Vdss (1 k/g) | Cl (ml/kg/min) | t1/2 el (h) | CSHT1 (min) | CSHT3 (min) | F (%) | |
---|---|---|---|---|---|---|
Dexmedetomidine | 2–3 | 9–30 (↓) | 2–3 | ~20 | ~40 | 94 |
Etomidate | 2–5 | 12–25 | 3–5 | 5 | 8 | 76 |
Ketamine | 1–3 | 11–18 | 2–3 | 5 | 22 | 50 |
Midazolam | 1–2 | 6–11 (↓) | 2–3 | 32 | 60 | 95 |
Propofol | 2–10 | 20–30 | 4–7 | 10 | 21 | 98 |
Thiopental | 1–3 | 3–5 | 7–17 | 80 | 120 | 75 |
There are various changes in the PK of propofol in the elderly patient . The central volume of distribution is less, systemic clearance is reduced, and intercompartmental clearance is reduced. During a propofol infusion, the plasma concentration of the drug is about 20% higher in the elderly patient as compared with the average adult [30]. Furthermore, the context-sensitive half-time changes with increasing age. Studies have shown that the time required for a 50% reduction in effect-site concentration (50% effect-site decrement time) is significantly prolonged with advancing age in an exponential manner. For propofol infusions less than 1 h, there is little difference in the recovery time of the young adult and the elderly patient. However, after a 4-h infusion, there is a doubling of the 50% effect-site decrement time in an 80 versus a 20-year-old patient, and this difference becomes even greater with infusions of 10 h and longer [30]. This fact is of particular importance because this assumes that there have already been dosage adjustments for other PK parameters such that the plasma concentration is the same in both the patients. Thus, even at reduced infusion rates, the elderly patient will take longer to emerge than the young patient.
Indications
Propofol, as noted in the previous section, is routinely used for induction and maintenance phases of general anesthesia, as well as for various levels of sedation in OR and non-OR anesthesia [1], and also in the ICU.
Dosing in the Elderly
When the PK and PD changes are considered together, the current literature suggests a 20% reduction in the induction dose of propofol, if given as a bolus (see Table 17.3) [33–37].Practically, this has been reported as a reduction of the bolus dose from 2.0–2.5 to 1.5–1.8 mg/kg [31]. Of note, it is the authors’ clinical experience that if the induction dose is titrated to a neurologic endpoint (such as BIS or PSA4000) or given slowly to account for the effect-site hysteresis time (k e0), this dose is reduced to as low as 0.8–1.2 mg/kg in the elderly, which corresponds with the findings of Kazama et al. [25]. Furthermore, numerous reports have shown that there is less hemodynamic instability if this bolus is given over a longer period of time in the elderly patient than one fast bolus [25, 30, 32].
Table 17.3
Uses and doses of commonly used nonopiates drugs
Induction/Maintenance | ||||
---|---|---|---|---|
Sedation (iv) | Bolus | Infusion | Elderly (% reduction) | |
Dexmedetomidine | 0.5–1 μg/kga | 0.5–3 μg/kga | 0.1–2.5 μg/kg/h | 30–50 |
Etomidate | 0.2–0.4 mg/kg | n/a | 20–50 | |
Ketamine | 0.2–0.5 mg/kg | 1–2 mg/kg | 10–20 μg/kg/min | ?0 |
Midazolam | 0.02 mg/kg | 0.025–0.1 mg/kg | 0.3–1.5 μg/kg/min | 20 |
Propofol | 10–50 μg/kg/min | 1.0–1.5 mg/kg | 75–150 μg/kg/min | 20 |
Thiopental | 2–5 mg/kg | n/a | 20 |
Dosing requirements during an infusion are even less for the elderly patient. Schüttler and Ihmsen [32] have shown that for continuous low plasma level infusions, such as those used during the maintenance phase of an anesthetic for sedation (plasma concentration 1 μg/mL), a 75-year-old patient will require approximately 30% less drug than a 25-year-old patient to maintain the same level of drug concentration. However, this only takes into account the PK changes with age (Fig. 17.1) [32]. The age-related decline in the amount of propofol required for the same level of anesthesia becomes even more profound when one considers the PD data along with the PK data. For a surgical level of anesthesia, Shafer proposes an age-adjusted dosing guideline based on the compilation of several PK and PD studies (Fig. 17.2) [12, 31]. This PD change is also illustrated in Fig. 17.3, which shows that a 75-year-old patient will require a 50% lower propofol plasma concentration than a 25-year-old patient to have the same likelihood of being asleep after a 1-h infusion [12]. Additionally, as aforementioned, it must be noted that a prolonged propofol infusion should be stopped earlier in the elderly patient to have recovery at the same time as the younger patient (Fig. 17.4) [32].





Fig. 17.1
Propofol infusion rate required to maintain 1 μg/mL plasma level of propofol in patients of various ages. These dosing guidelines take into account the pharmacokinetic changes with aging. This correlates with a mild level of sedation (Reprinted from Schuttler and Ihmsen [32]. With permission from Wolters Kluwer Health, Inc)

Fig. 17.2
Propofol infusion rate required to maintain adequate surgical anesthesia in patients of various ages. These dosing guidelines account for the changes with age in propofol pharmacokinetics and pharmacodynamics (Reprinted from Shafer [31]. with permission from Elsevier)

Fig. 17.3
Effect of age on propofol pharmacodynamics. This logistic regression shows the age-related probability of being asleep after a 1-h infusion of propofol. A 75-year-old patient is 30–50% more sensitive to propofol than is a 25-year-old patient (Reprinted from Schnider et al. [12]. With permission from Wolters Kluwer Health, Inc.)

Fig. 17.4
Context-sensitive half-time of propofol in patients of various ages. Altered pharmacokinetics in the elderly become clinically significant after a 1-h infusion (Reprinted from Schuttler and Ihmsen [32]. With permission from Wolters Kluwer Health, Inc.)
Adverse Effects and Contraindications
The major adverse effect of propofol, a significant decrease in blood pressure, has been already mentioned. If proper dosage adjustments are made, propofol is a well-tolerated induction and infusion medication in the elderly. However, in the patient with significant ventricular dysfunction or hemodynamic instability, it may be best to use etomidate or thiopental for bolus induction. It is also of note that propofol routinely causes pain on intravenous injection . However, this is normally brief and mitigated by lidocaine admixture or pretreatment [30].
Future Considerations
One potential benefit of the use of propofol in the elderly population is its speculated anti-inflammatory and antioxidant properties [38–41]. Ongoing resear ch supports that a propofol infusion for the maintenance of anesthesia decreases the magnitude of the rise of inflammatory markers in the elderly patient when compared with volatile anesthetic agents. This is of particular importance when it is viewed in light of the research showing that increases in inflammatory markers , such as interleukin (IL)-6, tumor necrosis factor-alpha, C-reactive protein, and myeloperoxidase, are associated with increased rates of cardiovascular mortality and morbidity and postoperative cognitive dysfunction [42, 43].
Thiopental
Barbituric acid, a combination of urea and malonic acid that is lacking in sedative properties, was first synthesized in 1864 by J.F.W. Adolph von Baeyer, a Nobel prizewinning organic chemist [44]. The thiobarbiturates were first described in 1903 . However, because of fatal experiments in dogs, their use was not further explored until the 1930s [45–47]. In 1935, Tabern and Volwiler synthesized a series of sulfur-containing barbiturates, of which thiopental became the most widely used. Thiopental was clinically introduced by Ralph Waters and John Lundy, and became the preferred agent clinically because of its rapid onset of action and short duration, without the excitatory effects of hexobarbital [48]. In 2011, the American manufacturer of thiopental announced that it would stop production of thiopental not only in the USA but also at its Italian plant (because it was a supplier to the USA), because of its objection of thiopental use for lethal injection.
Pharmacology: Structure/Action
Thiopental is a hypnotically active drug that works at GABAA receptor sites in the CNS [49]. Thiopental is of the class of thiobarbiturates , which is defined by having a sulfur substituted at the position C2. Substitutions at the 5, 2, and 1 positions of the barbiturate ring confer different pharmacologic activities to the barbiturate nucleus. Substitutions at position 5 with either aryl or alkyl groups produce hypnotic and sedative effects. A phenyl group substitution at C5 produces anticonvulsant activity. An increase in length of one or both side-chains of an alkyl group at C5 increases hypnotic potency. Substitution of a sulfur at position 2 produces a more rapid onset of action, as seen with thiopental [50].
Pharmacodynamics
Thiopental produces sedation and sleep . Sufficient doses produce a CNS depression that is attended by loss of consciousness, amnesia, and respiratory and cardiovascular depression. The response to pain and other noxious stimulation during general anesthesia seems to be obtunded. However, the results of pain studies reveal that barbiturates may actually decrease the pain threshold in low doses, such as with small induction doses of thiopental or after emergence from thiopental when the blood levels are low [51]. The amnesic effect of barbiturates has not been well studied, but it seems decidedly less pronounced than that produced by benzodiazepines or propofol [52].
Central Nervous System Effects
Barbiturates, similar to other CNS depressants, have potent effects on cerebral metabolism. Several studies in the 1970s demonstrated the effect of barbiturates as a dose-related depression of the CMRO2, which produces a progressive slowing of the EEG, a reduction in the rate of adenosine triphosphate consumption, and protection from incomplete or focal cerebral ischemia [53, 54]. When the results of the EEG became isoelectric, a point at which cerebral metabolic activity is approximately 50% of baseline, no further decrements in CMRO2 occurred [55]. These findings support the hypothesis that metabolism and function are coupled. However, it must be noted that it is the portion of metabolic activity concerned with neuronal signaling and impulse traffic that is reduced by barbiturates, not that portion corresponding to basal metabolic function. The only way to suppress baseline metabolic activity concerned with cellular activity is through hypothermia. Thus, the effect of barbiturates on cerebral metabolism is maximized at a 50% depression of cerebral function in which less oxygen is required as CMRO2 is diminished, leaving all metabolic energy to be used for the maintenance of cellular integrity [55]. This may be of importance to the elderly patient undergoing neurosurgery for aneurysm clipping or carotid endarterectomy in which focal ischemia may occur.
With the reduction in CMRO2, there is a parallel reduction in cerebral perfusion, which is seen in decreased CBF and ICP (Table 17.1). With reduced CMRO2, cerebral vascular resistance increases and CBF decreases [56]. However, for thiopental, the ratio of CBF to CMRO2 is unchanged. Thus, the reduction in CBF after the administration of barbiturates causes a concurrent decrease in ICP. Furthermore, even though the MAP decreases, barbiturates do not compromise the overall CPP, because the CPP = MAP – ICP. In this relationship, ICP decreases more relative to the decrease in MAP after barbiturate use, thus preserving CPP. This is in contrast to propofol, which has a greater likelihood in the elderly patient of decreasing MAP to an extent that may compromise CPP, as noted above [57].
Onset of Central Nervous System Effects
Barbiturates produce CNS effects when they cross the blood –brain barrier. There are several well-known factors that help to determine the rapidity with which a drug enters the cerebral spinal fluid (CSF) and brain tissue. These factors include the degree of lipid solubility, degree of ionization, level of protein binding, and the plasma drug concentration. Drugs with high lipid solubility and low degree of ionization rapidly cross the blood–brain barrier, producing a fast onset of action. Approximately 50% of thiopental is nonionized at physiologic pH, which accounts in part for the rapid accumulation of thiopental in the CSF after intravenous administration. Protein binding also affects the onset of action in the CNS. Barbiturates are highly bound to albumin and other plasma proteins. As only unbound drug (free drug) can cross the blood–brain barrier, an inverse relationship exists between the degree of plasma protein binding and the rapidity of drug passage across the blood–brain barrier.
The final factor governing the rapidity of drug penetration of the blood–brain barrier is the plasma drug concentration. Simply because of concentration gradient, higher levels of drug concentrations in the plasma produce greater amounts of drug that diffuses into the CSF and the brain. The two primary determinants of the plasma concentration are the dose administered and the rate (speed) of administration. The higher the dose and the more rapid its administration, the more rapid is the effect. This is of particular importance in the elderly patient who may have a reduced central volume of distribution and thus require a reduced dose of thiopental to reach the same plasma concentration as a younger adult [58].
Cardiovascular System
Cardiovascular depression from barbiturates is a result of both central and peripheral (direct vascular and cardiac) effects [59]. The hemodynamic changes produced by barbiturates have been studied in healthy individuals and in patients with heart disease. The primary cardiovascular effect of barbiturate induction is peripheral vasodilation that results in a pooling of blood in the venous system. A decrease in contractility is another effect, which is related to reduced availability of calcium to the myofibrils. There is also an increase in heart rate. Mechanisms for the decrease in cardiac output include (1) direct negative inotropic action, (2) decreased ventricular filling because of increased capacitance, and (3) transiently decreased sympathetic outflow from the CNS. The increase in heart rate (10–36%) that accompanies thiopental administration probably results from the baroreceptor-mediated sympathetic reflex stimulation of the heart in response to the decrease in output and pressure. Thiopental produces dose-related negative inotropic effects, which seem to result from a decrease in calcium influx into the cells with a resultant diminished amount of calcium at sarcolemma sites. The cardiac index is unchanged or is reduced, and the MAP is maintained or is slightly reduced [59]. Thiopental infusions and lower doses tend to be accompanied by smaller hemodynamic changes than those noted with rapid bolus injections; however, their use in current practice is exceedingly limited if not gone altogether [25].
The increase in heart rate encountered in patients with coronary artery disease anesthetized with thiopental (1–4 mg/kg) is potentially deleterious because of the obligatory increase in myocardial oxygen consumption (MVO2) that accompanies the increased heart rate. Patients who have normal coronary arteries have no difficulty in maintaining adequate coronary blood flow to meet the increased MVO2 [60]. When thiopental is given to hypovolemic patients, there is a significant reduction in cardiac output (69%) as well as a substantial decrease in blood pressure [45–48]. Patients without adequate compensatory mechanisms, therefore, may have serious hemodynamic disturbance with thiopental induction. All of these concerns are of particular importance in geriatric patients, because they are more likely to have clinically significant coronary artery disease, are more likely to be intravascularly hypovolemic, and their compensatory mechanisms to maintain heart rate and blood pressure may be reduced because of age-related alterations and pharmacologic treatments such as beta-blockers or calcium channel blockers. Thus, it is of prime importance in the elderly patient to understand proper dose reduction (discussed further) and the effects of the rate of administration of an induction bolus. If these are not heeded, it becomes common to have significant hypotension in the geriatric patient with the need to administer vasopressors after induction , a practice that can be avoided if a proper understanding of the above principle is gained.
Respiratory System
Barbiturates produce dose-related central respiratory depression. There is also a significant incidence of transient apnea after their administration for induction of anesthesia [15]. The evidence for central depression is a correlation between EEG suppression and minute ventilation [61]. With increased anesthetic effect, there is diminished minute ventilation. The time course of respiratory depression has not been fully studied, but it seems that peak respiratory depression (as measured by the slope of CO2 concentration in the blood) and minute ventilation after delivery of thiopental 3.5 mg/kg occurs 1–1.5 min after administration. These parameters rapidly return to predrug levels, and within 15 min the drug effects are barely detectable [62]. Of note, respirations are lost sooner and return later than that seen with propofol. Patients with chronic lung disease are slightly more susceptible to the respiratory depression of thiopental. The usual ventilatory pattern with thiopental induction has been described as “double apnea.” The initial apnea that occurs during drug administration lasts a few seconds and is succeeded by a few breaths of reasonably adequate tidal volume, which is followed by a longer apneic period. During the induction of anesthesia with thiopental, ventilation must be assisted or controlled to provide adequate respiratory exchange. This is of particular concern in the elderly patient who will have an increased closing capacity, which will produce a shorter time to become hypoxemic, as compared with the young adult patient [21].
Metabolism and Disposition (Pharmacokinetics)
Thiopental PK has been described in both physiologic and compartmental models . These models basically describe a rapid mixing of the drug with the central blood volume followed by a quick distribution of the drug to the highly perfused, low-volume tissues (i.e., brain) with a slower redistribution of the drug to lean tissue (muscle). In these models, adipose tissue uptake and metabolic clearance (elimination) have only a minor role in the termination of the effects of the induction dose because of the minimal perfusion ratio compared with other tissues and the slow rate of removal, respectively. Both of these PK models describe rapid redistribution as the primary mechanism that terminates the action of a single induction dose [31, 63].
Awakening may be delayed in older patients mainly because of a decreased central volume of distribution relative to younger adults [64]. The initial volume of distribution is less in elderly patients when compared with that in young patients (80 versus 35-year-old patient), which explains a 50–75% lower dose requirement for the onset of EEG and hypnotic effects [58, 64]. However, except in disease states, the clearance of thiopental is not reduced in the elderly, and thus, awakening should only be prolonged in the elderly with a bolus administration and not with a constant infusion.
Indications
Although supply has been limited in many countries in recent years, thiopental is an excellent hypnotic drug for use as an intravenous induction agent and continues widespread use in low and middle-income countries [62]. The prompt onset (15–30 s) of action and smooth induction make thiopental a reasonable choice, as long as the cardiovascular limitations and dangers noted above are taken into account. The relatively rapid emergence, particularly after single use for induction, has also been a reason for the widespread use of thiopental in this setting. Thiopental does not possess analgesic properties and therefore it must be supplemented with analgesic drugs to obtund reflex responses to noxious stimuli during anesthesia induction, intubation, and surgical procedures. Thiopental can be used to maintain general anesthesia , because repeated doses reliably sustain unconsciousness and contribute to amnesia. However, the ease of using propofol for light sedation and total intravenous anesthesia has supplanted the use of thiopental for this purpose and relegated it mainly for use in the induction portion of an anesthetic.
Dosing in the Elderly
In contrast to propofol, numerous studies have shown that the brain of the elderly patient is not intrinsically more sensitive to the effects of thiopental than that of the younger patient [58]. Further studies concluded that the need for a reduction in the induction dose of thiopental in the elderly is attributable to a reduction in the central volume of distribution [65]. Shafer [31] collated the results of several studies to suggest that the optimal dose in an 80-year-old patient is 2.1 mg/kg, which is approximately 80% of the dose needed for a young adult. However, it should again be noted that slower bolusing of the induction dose will generally result in less-acute hemodynamic alterations. Furthermore, monitoring of an EEG-related endpoint during a slow induction can guide the amount of drug given and may allow for a more individualized dosing regimen [66].
Adverse Effects and Contraindications
The effects of barbiturates on various organ systems have been extensively studied. There are several side effects that occur in unpredictable, varying proportions in patients, whereas the cardiovascular and pulmonary side effects are dose related [67]. The complications of injecting barbiturates include garlic or onion taste (40% of patients), allergic reactions, local tissue irritation, and, rarely, tissue necrosis. An urticarial rash may develop on the head, neck, and trunk that lasts a few minutes. More severe reactions such as facial edema, hives, bronchospasm, and anaphylaxis can occur. Treatment of anaphylaxis is to stop any further administration of the drug, administer 1-mL increments of 1:10,000 epinephrine with boluses of intravenous fluids, give inhaled bronchodilators, such as albuterol, for bronchospasm, and then administer histamine antagonists, such as diphenhydramine and famotidine.
Studies have shown pain on injection to be 9% and phlebitis to be approximately 1% with thiopental use [50]. Tissue and venous irritation are more common if a 5% solution is used rather than the standard 2.5% solution. Rarely, intra-arterial injection can occur. The consequences of accidental arterial injection may be severe. The degree of injury is related to the concentration of the drug. Treatment consists of (1) dilution of the drug by the administration of saline into the artery, (2) heparinization to prevent thrombosis, and (3) brachial plexus block. Overall, the proper administration of thiopental intravenously into a briskly running IV is remarkably free of local toxicity [67]. However, it should be noted that thiopental can precipitate if the alkalinity of the solution is decreased, which is why it cannot be reconstituted with lactated Ringer’s solution or mixed with other acidic solutions. Examples of drugs that are not to be coadministered or mixed in solution with the barbiturates are pancuronium, vecuronium, atracurium, alfentanil, sufentanil, and midazolam. Studies have shown that in rapid-sequence induction, the mixing of thiopental with vecuronium or pancuronium results in the formation of precipitate that may occlude the intravenous line [50].
Midazolam
The first benzodiazepine found to have sedative-hypnotic effects was chlordiazepam in 1955 [68]. Diazepam was synthesized in 1959 and became the first benzodiazepine used for sedation and anesthesia induction. Subsequently, a number of benzodiazepines have been produced including lorazepam and the antagonist flumazenil. The benzodiazepines produce many of the elements important in anesthesia. They produce their actions by occupying the benzodiazepine receptor, which was first presented in 1971 [69]. In 1977, specific benzodiazepine receptors were described when ligands were found to interact with a central receptor [70]. The most frequently used benzodiazepine in the elderly is midazolam. Fryer and Walser’s 1976 synthesis of midazolam produced the first clinically used water-soluble benzodiazepine [71]; it was also the first benzodiazepine that was produced primarily for use in anesthesia [72].
Pharmacology : Structure/Action
Midazolam is water soluble in its formulation, but highly lipid soluble at physiologic pH [72]. Midazolam solution contains 1 or 5 mg/mL midazolam with 0.8% sodium chloride and 0.01% disodium edetate, with 1% benzyl alcohol as a preservative. The pH is adjusted to 3 with hydrochloric acid and sodium hydroxide. The imidazole ring of midazolam accounts for its stability in solution and rapid metabolism. The high lipophilicity accounts for the rapid CNS effect, as well as for the relatively large volume of distribution [73].
Pharmacodynamics
Central Nervous System Effects
All benzodiazepines have hypnotic, sedative, anxiolytic, amnesic, anticonvulsant, and centrally produced muscle relaxant properties. The drugs differ in their potency and efficacy with regard to each of these PD actions. The binding of benzodiazepines to their respective receptors is of high affinity, stereospecific, and able to fully saturate the receptors; the order of receptor affinity (thus potency) of the three agonists is lorazepam > midazolam > diazepam. Midazolam is approximately three to six times as potent as diazepam [74].
The mechanism of action of benzodiazepines is reasonably well understood [75–77]. The interaction of ligands with the benzodiazepine receptor represents an example in which the complex systems of biochemistry, molecular pharmacology, genetic mutations, and clinical behavioral patterns are seen to interact. Through recent genetic studies, the GABAA subtypes have been found to mediate the different effects (amnesic, anticonvulsant, anxiolytic, and sleep) [78]. Sedation, anterograde amnesia, and anticonvulsant properties are mediated via a1 receptors [78], and anxiolysis and muscle relaxation are mediated by the a2 GABAA receptor [78]. The degree of effect exerted at these receptors is a function of plasma level. By using plasma concentration data and PK simulations, it has been estimated that a benzodiazepine receptor occupancy of less than 20% may be sufficient to produce the anxiolytic effect, whereas sedation is observed with 30–50% receptor occupancy and unconsciousness requires 60% or higher occupation of benzodiazepine agonist receptors [78].
Agonists and antagonists bind to a common (or at least overlapping) area of the benzodiazepine portion of the GABAA receptor by forming differing reversible bonds with it [79, 80]. The effects of midazolam can be reversed by use of flumazenil, a benzodiazepine antagonist that occupies the benzodiazepine receptor, but produces no activity and therefore blocks the actions of midazolam. The duration of reversal is dependent on the dose of flumazenil and the residual concentration of midazolam.
The onset and duration of action of a bolus intravenous administration of midazolam largely depends on the dose given and time at which the dose is administered; the higher the dose given over a shorter time (bolus), the faster the onset. Midazolam has a rapid onset (usually within 30–60 s) of action. The time to establish equilibrium between plasma concentration and EEG effect of midazolam is approximately 2–3 min and is not affected by age [81]. Like onset, the duration of effect is related to lipid solubility and blood level [82]. Thus, termination of effect is relatively rapid after midazolam administration. But some physicians have a general sense that midazolam is associated with the production of confusion even after the termination of sedation. This has been reported in prior studies and case reports [83, 84]. However, a more recent study suggests that this might not be the case, particularly at lower doses [85]. Taken together, these data seem to suggest that single, lower doses of midazolam (0.03 mg/kg) will not cause confusion, whereas higher doses (0.05–0.07 mg/kg) along with an infusion of midazolam will have a greater association with confusion in the geriatric patient, as opposed to that seen with the use of a low-dose propofol infusion [83–85].
Respiratory Effects
Midazolam, like most intravenous anesthetics and other benzodiazepines, produces dose-related central respiratory system depression. The peak decrease in minute ventilation after midazolam administration (0.15 mg/kg) is almost identical to that produced in healthy patients given diazepam (0.3 mg/kg) [86]. Respiratory depression is potentiated with opioids and must be carefully monitored in elderly patients getting both. The peak onset of ventilatory depression with midazolam (0.13–0.2 mg/kg) is rapid (about 3 min), and significant depression remains for about 60–120 min [63, 87]. The depression is dose related. The respiratory depression of midazolam is more pronounced and of longer duration in patients with chronic obstructive pulmonary disease, and the duration of ventilatory depression is longer with midazolam (0.19 mg/kg) than with thiopental (3.3 mg/kg) [63].
At sufficient doses, apnea occurs with midazolam as with other hypnotics. The incidence of apnea after thiopental or midazolam when these drugs are given for induction of anesthesia is similar. In clinical trials, apnea occurred in 20% of 1130 patients given midazolam for induction and 27% of 580 patients given thiopental [72]. Apnea is related to dose and is more likely to occur in the presence of opioids. Older age [88] debilitating disease and other respiratory depressant drugs probably also increase the incidence and degree of respiratory depression and apnea with midazolam.
Cardiovascular Effects
Midazolam alone has modest hemodynamic effects. The predominant hemodynamic change is a slight reduction in arterial blood pressure, resulting from a decrease in systemic vascular resistance. The hypotensive effect is minimal and about the same as seen with thiopental [89]. Despite the hypotension, midazolam, in doses as high as 0.2 mg/kg, is safe and effective for induction of anesthesia even in patients with severe aortic stenosis. The hemodynamic effects of midazolam are dose related: the higher the plasma level, the greater the decrease in systemic blood pressure [90]; however, there is a plateau plasma drug effect above which little change in arterial blood pressure occurs. The plateau plasma level for midazolam is 100 ng/mL [90]. Heart rate, ventricular filling pressures, and cardiac output are maintained after induction of anesthesia with midazolam.
The stimulation of endotracheal intubation and surgery are not blocked by midazolam [91]. Thus, adjuvant anesthetics, usually opioids, are often combined with benzodiazepines. The combination of benzodiazepines with opioids and nitrous oxide has been investigated in patients with ischemic and valvular heart diseases [92–95]. Although the addition of nitrous oxide to midazolam (0.2 mg/kg) has trivial hemodynamic consequences, the combination of benzodiazepines with opioids does have a synergistic effect [96]. The combination of midazolam with fentanyl [93] or sufentanil [95] produces greater decreases in systemic blood pressure than does each drug alone.
Metabolism and Disposition (Pharmacokinetics)
Biotransformation of all benzodiazepines occurs in the liver. The two principal pathways involve either hepatic microsomal oxidation (N-dealkylation or aliphatic hydroxylation) or glucuronide conjugation [97, 98]. The difference in the two pathways is significant, because oxidation is susceptible to outside influences and can be impaired by certain population characteristics (specifically, old age), disease states (e.g., hepatic cirrhosis), or the coadministration of other drugs that can impair oxidizing capacity (e.g., cimetidine). Of the two, conjugation is less susceptible to these factors [97]. Midazolam undergoes oxidation reduction, or phase I reactions, in the liver [99]. The cytochrome P450 3A4 is primarily responsible for metabolism [100]. The fused imidazole ring of midazolam is rapidly oxidized by the liver, which accounts for the high rate of hepatic clearance. Neither age nor smoking decreases midazolam biotransformation [101]. Chronic alcohol consumption increases the clearance of midazolam [102].
The metabolites of the benzodiazepines can be important. Midazolam is biotransformed to hydroxymidazolams , which are active metabolites, and when midazolam is given in prolonged infusions, these metabolites can accumulate [103]. These metabolites rapidly conjugate and are excreted in the urine. The 1-hydroxymidazolam has an estimated clinical potency of 20–30% of midazolam [104]. It is primarily excreted by the kidneys and can cause profound sedation in patients with renal impairment [105]. Overall, the metabolites of midazolam are less potent and normally more rapidly cleared than the parent drug, making them of little concern in patients with normal hepatic and renal function. However, they may be a consideration in elderly patients with impaired renal function.
Midazolam is classified as a short-lasting benzodiazepine . The plasma disappearance curves of midazolam can be fitted to a two or three-compartment model. The clearance rate of midazolam ranges from 6 to 11 mL/kg/min [101]. Although the termination of action of these drugs is primarily a result of redistribution of the drug from the CNS to other tissues after bolus or maintenance use for surgical anesthesia, after daily (long-term) repeated administration or after prolonged continuous infusion , midazolam blood levels decreases more slowly.
Factors k nown to influence the PK of benzodiazepines are age, gender, race, enzyme induction, sepsis-related organ dysfunction, and hepatic and renal disease [106, 107]. Age reduces the clearance of midazolam to a modest degree [104]. Among the PK parameters of midazolam that vary significantly with age, it is clearance which does so most consistently [105]. In healthy adults, midazolam clearance is high, approximating 50% of hepatic blood flow [106]. However, with advanced age, there is a loss of functional hepatic tissue and a decrease in hepatic perfusion such that clearance is reduced in the elderly by as much as 30% from that of a young adult; recent modeling has predicted a 27% reduction in metabolic clearance in older versus younger patients [108]. The decreased clearance is not a result of age-related changes in CYP3A4 enzymatic activity in the liver which is unaffected by age [106]. As a result of the normal decline in lean tissue mass and concomitant increase in percent body fat in the aged, a slight increase is also observed in volume of distribution [107]. Moreover, according to one study, advanced age is in itself enough to cause the mean elimination half-life of midazolam to double [43]. Neither oral bioavailability nor midazolam protein binding are affected by age, despite reduced hepatic albumin synthesis and lower serum albumin concentrations in the elderly [105]. Finally, there appear to be no significant differences in PK variables between repeated bolus and continuously infused midazolam when used in ICU sedative doses [109].
Midazolam PK is affected by obesity. The volume of distribution is increased as drug goes from the plasma into the adipose tissue. Although clearance is not altered, elimination half-lives are prolonged, because of the delayed return of the drug to the plasma in obese individuals [104]. This can be of concern in elderly obese patients. Although the PK of midazolam is clearly affected by age, they are, with the exception of total clearance, not consistently altered to statistical significance. These PK changes with age do not explain [103] the increased sensitivity of the elderly to midazolam discussed above. There are PD factors that are yet to be fully understood that make midazolam more potent in the elderly than the young.
Indications
Intravenous Sedation
Midazolam is used for sedation as preoperative premedication [110], intraoperatively during regional or local anesthesia, and postoperatively for sedation. The anxiolysis, amnesia, and elevation of the local anesthetic seizure threshold are desirable benzodiazepine actions for regional anesthesia. It should be given by titration for this use; endpoints of titration are adequate sedation or dysarthria and maintained ventilation. The onset of action is relatively rapid with midazolam, usually with peak effect reached within 2–3 min of administration. There is an excellent correlation within individuals of sedation score to blood level, but between individuals there is considerable variation in blood level and sedation [107]. The duration of action primarily depends on the dose used. There is often a disparity in the level of sedation compared with the presence of amnesia (patients can be seemingly conscious and coherent, yet they are amnesic for events and instructions). The degree of sedation and the reliable amnesia, as well as preservation of respiratory and hemodynamic function, are better overall with midazolam than with other sedative-hypnotic drugs used for conscious sedation with the possible exception of propofol [88]. However, in elderly patients, inadvertent overdose for sedation during endoscopy has been reported to cause a decline in cognitive function [111]. Also, in a small percentage of patients (1.4%), a paradoxical reaction successfully treated with flumazenil has been reported during endoscopy resulting in agitation rather than sedation [112]. When using midazolam for sedation, a sedation score such as the Ramsay Sedation Scale is commonly used whereas the BIS in elderly patients might result in less-reliable sedation monitoring [113]. When midazolam is compared with propofol for sedation, the two are generally similar except that emergence or wake-up is more rapid with propofol [88]. Midazolam and propofol require close medical supervision because of potential respiratory depression and hypotension [108, 109]. Despite the wide safety margin with midazolam, respiratory function must be monitored when it is used for sedation to prevent undesirable degrees of respiratory depression [63, 114, 115]. This is especially true in the geriatric patient and when opioids are also given [88, 116]. There may be a slight synergistic action between midazolam and spinal anesthesia with respect to ventilation [117]. Thus, the use of midazolam for sedation during regional and epidural anesthesia requires vigilance with regard to respiratory function, when these drugs are given with opioids. Sedation for longer periods, for example, in the ICU, is accomplished with benzodiazepines. Prolonged infusion will result in accumulation of drug and, in the case of midazolam, significant concentration of the active metabolite. The chief advantages are the amnesia and hemodynamic stability, and the disadvantage, compared with propofol, is the longer dissipation of effects when infusion is terminated.
Induction and Maintenance of Anesthesia
With midazolam, induction of anesthesia is defined as unresponsiveness to command and loss of the eyelash reflex. When midazolam is used in appropriate doses, induction occurs less rapidly than with thiopental or propofol [72], but the amnesia is more reliable. Numerous factors influence the rapidity of action of midazolam. These factors are dose, speed of injection, degree of premedication, age, American Society of Anesthesiologists (ASA) physical status, and concurrent anesthetic drugs [72, 110]. In a well-premedicated, healthy patient, midazolam (0.2 mg/kg given in 5–15 s) will induce anesthesia in 28 s. The BIS may be used to monitor depth with midazolam and adjuvant drugs during anesthesia [118, 119]. Emergence time is related to the dose of midazolam as well as the dose of adjuvant anesthetic drugs [72]. Emergence is more prolonged with midazolam than with propofol [120, 121]. This difference accounts for some anesthesiologists’ preference for propofol induction for short operations. The best method of monitoring depth with midazolam is use of the BIS [122]. Over the years since its introduction into practice, midazolam has become much less used for induction and maintenance of anesthesia in part because of the delirium found with higher doses of the drug [123].
The amnesic period after an anesthetic dose is about 1–2 h. Infusions of midazolam have been used to ensure a constant and appropriate depth of anesthesia. Experience indicates that a plasma level of more than 50 ng/mL when used with adjuvant opioids (e.g., fentanyl) and/or inhalation anesthetics (e.g., nitrous oxideand volatile anesthetics) is achieved with a bolus loading dose of 0.05–0.15 mg/kg and a continuous infusion of 0.25–1 μg/ kg/min [124]. This is sufficient to keep the patient asleep and amnesic but arousable at the end of surgery. Midazolam when compared with dexmedetomidine MAC sedation during endoscopic nasal surgery produces more amnesia than dexmedetomidine [125]. Lower infusion doses almost certainly are required in elderly patients and with certain opioids.
Effects of Age on Pharmacology
Elderly patients require lower doses of midazolam than younger patients to reach various standard clinical endpoints of sedation, such as response to verbal command (Fig. 17.5) [126]. The usual induction dose of midazolam in elderly premedicated patients is between 0.05 and 0.15 mg/kg. Some studies show that patients older than 55 years and those with ASA physical status higher than 3 require a 20% or more reduction in the induction dose of midazolam [72]. However, Shafer, who collated the results of numerous PK and PD studies, recommends a 75% reduction in dose from the 20 to the 90-year-old patient. Thus, there is definitely a graded decrease in the amount of drug needed as a result of aging [31]. In a recent comparative study of midazolam and propofol in elderly patients (65–93 years old) having hip surgery under spinal anesthesia, midazolam and propofol were similar with regard to hemodynamic and most ventilatory effects, but midazolam prolonged recovery time [127]. Finally, when midazolam is used with other anesthetic drugs (coadministration), there is a synergistic interaction [124, 128, 129], and the induction dose is less than 0.1 mg/kg. The synergy is seen when midazolam is used with opioids and/or other hypnotics such as thiopental, propofol, and etomidate.


Fig. 17.5
Response curves to verbal commands in patients of various ages at varying plasma levels of midazolam. This demonstrates a pharmacodynamic change associated with aging in response to midazolam (Reprinted from Jacobs et al. [126]. With permission from Wolters Kluwer Health Inc.)
Awakening after midazolam anesthesia is the result of the redistribution of drug from the brain to other, less well-perfused tissues. The emergence (defined as orientation to time and place) of young, healthy volunteers who received 10 mg of intravenous midazolam occurred in about 15 min [128], and, after an induction dose of 0.15 mg/kg, it occurred in about 17 min. The effect of age on emergence has not been well studied, but it likely is prolonged compared with younger patients because of greater potency in the elderly.
Adverse Effects and Contraindications
Midazolam is a remarkably safe drug . It has a relatively high margin of safety, especially when compared with barbiturates. It is also free of allergenic effects and does not suppress the adrenal gland [129]. The most significant problem with midazolam is respiratory depression. It is free of venous irritation and thrombophlebitis, problems related to aqueous insolubility, and requisite solvents in other drug formulations [72]. When used as a sedative or for induction and maintenance of anesthesia, midazolam can produce an undesirable degree or prolonged interval of postoperative amnesia, sedation, and, rarely, respiratory depression. These residual effects can be reversed with flumazenil.
Etomidate
Pharmacology : Structure/Action
Etomidate is a hypnotic drug that is structurally unrelated to all other induction medications. It contains a carboxylated imidazole ring that provides water solubility in an acidic milieu and lipid solubility at physiologic pH. It is dissolved in propylene glycol, which often causes pain on injection. Etomidate works by depressing the reticular activating system and enhances the inhibitory effects of GABA by binding to a subunit of the GABAA receptor and thereby increasing its affinity for GABA. However, unlike the barbiturates, which have global depressant effects on the reticular activating system, etomidate has some disinhibitory effects, which accounts for the 30–60% rate of myoclonus with administration. Interestingly, one study has shown that this unwanted side effect can be reduced with pretreatment, similar to a defasciculating dose of neuromuscular blocking drugs (NMBDs) [130].
Pharmacodynamic s
Central Nervous System Effects
Etomidate induces changes in CBF, metabolic rate, and ICP to the same extent as thiopental and propofol. However, because this is not the result of a large reduction in arterial blood pressure, CPP is well maintained [130]. This is of particular importance in the elderly person who is at risk for ischemic stroke secondary to carotid occlusion. Etomidate has EEG changes similar to thiopental with a biphasic pattern of activation followed by depression. However, etomidate has been shown to activate somatosensory evoked potentials [57]. Additionally, etomidate causes myoclonic movements after induction, a disturbing effect of unknown significant, in approximately 75% of patients but this is not evidence of an insufficient induction dose [131]. Of note, etomidate does have a higher rate of postoperative nausea and vomiting associated with it than with the other intravenous induction drugs [132]. Finally, there are no PD changes with age with respect to etomidate as measured by EEG [130].
Cardiovascular Effects
Unlike propofol, etomidate has minimal effects on the cardiovascular system. There is a slight decline in the arterial blood pressure secondary to a mild reduction in the systemic vascular resistance. Etomidate does not seem to have direct myocardial depressant effects, because myocardial contractility, heart rate, and cardiac output are usually unchanged [133]. Etomidate does not cause histamine release. These aspects of the PD of etomidate make it very useful in the patient with compromised intravascular volume, coronary artery disease, or reduced ventricular function, as is often encountered in the elderly patient.
Respiratory Effects
Etomidate causes less respiratory depression than benzodiazepines, barbiturates, or propofol in induction doses. In fact, even an induction dose of etomidate often does not cause apnea [61]. This fact, combined with its minimal cardiovascular effects, makes etomidate a very useful drug in the setting of a hemodynamically brittle elderly patient with a possible difficult airway and little respiratory reserve.
Endocrine Effects
Induction doses of etomidate temporarily inhibit the synthesis of cortisol and aldosterone, lasting approximately 12–18 h after a single bolus dose [134]. However, the clinical significance of this has been debated in septic patients. Some studies have shown an increased mortality with a single bolus dose in septic patients, whereas others have not [135]. Alternatively, long-term infusions or closely repeated exposures can lead to adrenocortical suppression, which may be associated with an increased susceptibility to infection and an increased mortality rate in the critically ill patient [136].
Metabolism and Disposition (Pharmacokinetics)
Etomidate is used only in intravenous formulations and is generally used for the induction of general anesthesia. Etomidate is similar to thiopental in its distribution and onset of action. Although it is highly protein bound, etomidate has a very rapid onset of action because of its high lipid solubility and its large nonionized fraction. Redistribution to noncentral compartments is responsible for its rapid offset of action. Hepatic microsomal enzymes as well as plasma esterases rapidly hydrolyze etomidate to its nonactive metabolites. This rate of biotransformation is five times greater than that of thiopental, but less than that of propofol.
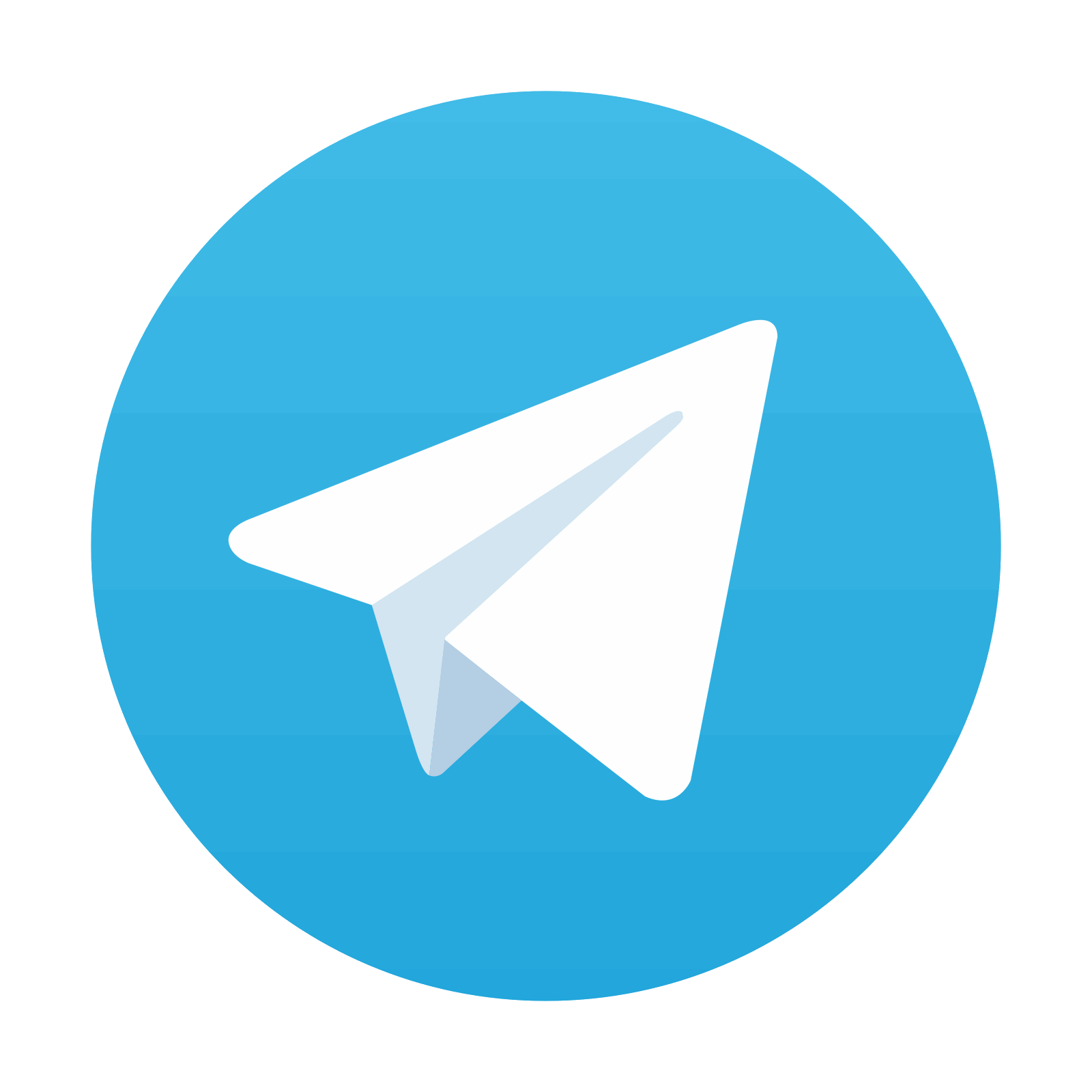
Stay updated, free articles. Join our Telegram channel

Full access? Get Clinical Tree
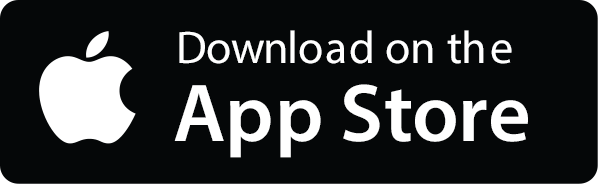
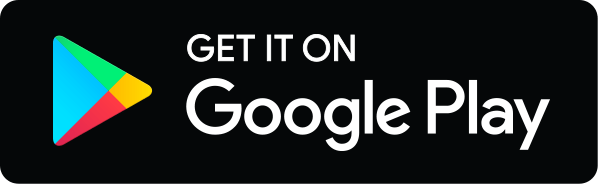