All aspects of the management of intracranial arterial disease have undergone major transformations in the past two decades. Alternatives are now available for conditions previously treatable only with a single modality. Moreover, some conditions that would have been considered untreatable in the past now have safe and effective therapies. Although the credit for these advances is attributable to many factors, several fundamental achievements are recognized. First, the advances in noninvasive diagnostic neuroimaging, namely, computed tomographic (CT) and magnetic resonance (MR) imaging and their respective variants have resulted in the increased detection of asymptomatic vascular lesions and improved diagnostic sensitivity. As will be discussed later, this early detection presents a clinical dilemma in determining the appropriate management on the basis of the natural history of specific lesions. Second, the refinement of microneurosurgical techniques has resulted in improved surgical outcomes. This experience has also allowed us to recognize the limitations of cerebrovascular surgery. Third, the advances in neurological critical care have resulted in improved morbidity rates and shorter hospitalizations. Finally, the emergence of neuroendovascular therapy into a multidisciplinary field involving neurosurgeons, neurologists, and radiologists has changed practice patterns dramatically. The ongoing explosion in endovascular advances has allowed for catheter-based therapeutics in virtually every aspect of cerebrovascular disease.
In this chapter, the current concepts of the major categories of intracranial vascular disease are reviewed. Given the inherent limitations of covering such a broad subject in one chapter, some discussions are very cursory and superficial. The topics most likely to be encountered in clinical practice, namely, aneurysms, ischemic stroke, and arteriovenous malformations (AVMs), are treated more rigorously.
To gain insight into the numerous pathological conditions of the human intracranial arteries and to develop sound therapies for these disorders, a review of the gross and microscopic structure of normal arteries is necessary. The walls of the cerebral arteries are composed of three regions. From the lumen to the outer surface, these layers are the tunica intima, tunica media, and tunica adventitia. The intima is composed of an endothelial cell layer and the internal elastic lamina. The media is relatively thin and is composed of 4 to 20 smooth muscle layers that account for roughly half the thickness of the artery wall.1 Collagen fibers and fibroblasts mostly comprise the adventitia. Innervation of the cerebral arteries is by myelinated and unmyelinated nerve fibers coursing in the adventitia or between the media and adventitia.2
There are notable differences between the structure of the intracranial and systemic arteries. The internal elastic lamina is extremely convoluted and well developed in the cerebral arteries.3 The internal elastic lamina has long been thought to account for most of the mechanical strength of the artery.4 Cerebral arteries have a smaller wall-to-lumen ratio than their systemic counterparts.5 In contrast to peripheral arteries, intracranial arteries have few elastic fibers within the media. The external elastic lamina is absent, and the adventitia is poorly developed in the cerebral arteries. Unlike the well-developed fibrous layer seen in systemic arteries, the adventitia of intracranial arteries is sparse and composed of a loose network of connective tissue. Cerebral arteries generally do not have vasa vasorum. Instead, the cerebrospinal fluid (CSF) that surrounds these vessels in the subarachnoid space provides nutrients through the porous adventitia.6 The adventitia also contains a distinct cell type that resembles the interstitial cells of Cajal; these cells may be responsible for rhythmic contraction of the arteries and may play a role in the regulation of cerebral blood flow (CBF).2
The conventional cerebral arterial structure described above becomes disorganized at bifurcation or branching points. Here, the internal elastic lamina is increasingly fenestrated, and the media is thin or absent. The smooth muscle cells are arranged in an organized, circumferential pattern around the lumen in straight segments of the arteries. However, the muscle cell orientation is random and multidirectional at branching points. In addition, asymmetric rings of connective tissue can be found encompassing the lumen at branching sites. Known as “intimal cushions,” these structures are composed of connective tissue matrix and smooth muscle cells. These cushions are common in individuals older than 20 years of age, but their functional significance is unknown. It has been observed that the media layer is attenuated or absent under large intimal cushions.7
Although cerebral angiography has been supplanted over the past two decades by noninvasive imaging for many diagnostic purposes, it has experienced a revival in recent years with the expansion of endovascular therapy. A thorough understanding of angiographic anatomy is mandatory before proceeding with any cerebrovascular intervention, either surgical or endovascular. In broad terms, the carotid artery and its intracranial branches are referred to as the anterior circulation, and the vertebrobasilar system is known as the posterior circulation.
Despite its invasiveness, cerebral angiography can be performed with low risk. More than 5500 diagnostic angiograms have been performed at the authors’ institution during the past 8 years with a complication rate of 0.3% (Hopkins LN, personal communication, July 2007). Cerebral angiography is performed typically through a transfemoral route, although the radial or brachial arteries may be used to gain vascular access in cases of aortoiliac occlusive disease. For a thorough discussion on cerebral angiographic anatomy, refer the comprehensive works by Krayenbuhl and Yasargil,8 Morris,9 and Osborn.10,11 A normal angiogram is shown in Figure 23-1 to serve as a guide for abnormal studies included later in this chapter.
FIGURE 23-1.
Normal angiographic anatomy. Right ICA injection—(A) Anteroposterior and (B) lateral intracranial views. In this patient, the right ACA is hypoplastic (a hypoplastic A1 is a common anatomic variant); consequently, the MCA branches can be easily visualized on the lateral projection. MCA branches: orbitofrontal, prefrontal, precentral sulcus, central sulcus, postcentral sulcus, angular, temporo-occipital. ICA segments: supraclinoid, ophthalmic, cavernous, laceral, petrous, and cervical. Left ICA injection—(C) Anteroposterior and (D) lateral intracranial views. Reciprocally, the left A1 is dominant with filling across the anterior communicating artery to supply bilateral A2 segments. ACA segments: right A2, left A2, and A1. MCA segments: M1, superior M2, and inferior M2. Left VA injection—(E) Anteroposterior and (F) lateral intracranial views. Right ECA injection lateral views—(G) Proximal and (H) distal views.


In this section, we review briefly the main routes for collateral circulation that are important in the setting of intracranial or extracranial artery occlusion or that of therapeutic intervention. The collateral network of the brain includes anastomoses between the cerebral arteries themselves and between the cerebral arteries and the extracranial vessels. For a comprehensive discussion, refer the texts by Wojak12 and by Marinkovic et al.13 The anastomoses can be grouped into the following six categories based on location: Cervical, extracranial (in the head but outside the cranium), extracranial–intracranial (EC–IC), intracranial–extracerebral, extracerebral–cerebral, and cerebral.
EC–IC collaterals interconnect branches of the external carotid artery (ECA) and the internal carotid artery (ICA) or branches of the ECA and the vertebral artery (VA). These anastomoses are located primarily in the nasal cavity, orbit, and tympanic cavity. The therapeutic implications are realized when embolization through what seems to be a benign ECA branch leads to a profound neurological deficit. Furthermore, small branches from the ECA territory supply cervical nerve roots and cranial nerves.
Branches of the ophthalmic artery (off the ICA) form anastomoses with branches of the ECA, usually in the setting of ICA occlusion (Figure 23-2). Examples include connections between the angular branch of the facial artery and the dorsal nasal branch of the ophthalmic artery, between the frontal division of the superficial temporal artery (STA) (off the ECA) and the supratrochlear branch of the ophthalmic artery, and between the anterior deep temporal artery (off the internal maxillary artery) and the lacrimal branch of the ophthalmic artery.13 The nasal and tympanic branches off the ECA also form anastomotic networks that may be important in chronic ICA occlusion. Persistent embryonic vessels, such as the persistent stapedial or hypoglossal arteries, also belong in the EC–IC category.
FIGURE 23-2.
External–internal carotid artery collaterals. This patient presented with a right hemispheric transient ischemic attack. A right common carotid artery injection demonstrated ICA occlusion at its origin. (A) Only the ECA branches are filling. (B) An intracranial view shows collaterals between the internal maxillary artery and ophthalmic artery (dotted circle) with resultant filling of the intracranial ICA proximally and distally.

The ascending pharyngeal artery is a particularly dangerous vessel. The meningeal branch of the ascending pharyngeal artery forms anastomoses with the cavernous and petrous branches of the ICA. Other branches off this artery supply the trigeminal ganglion and lower cranial nerves. These branches have major implications during embolization of skull base tumors, especially those involving the jugular foramen. Rarely, the posterior inferior cerebellar artery may originate directly from the ascending pharyngeal artery rather than the VA.12
The occipital artery forms collaterals with the muscular branches of the VA and may reconstitute the distal VA in the setting of proximal occlusion. This artery may give rise to a stylomastoid branch that supplies the facial nerve as it exits the skull. We have had one case of a complete facial palsy developing after embolization of the occipital artery for a dural arteriovenous fistula (DAVF).
The internal maxillary artery forms several potentially dangerous anastomoses with branches of the ophthalmic artery and dural branches of the ICA. The middle meningeal artery, which arises proximally off the internal maxillary artery, is involved in many of these anastomoses. Additionally, the middle meningeal artery supplies the trigeminal ganglion and the first division of the trigeminal nerve.12
This group includes connections directly between the cerebral arteries. The circle of Willis is the best-known anastomotic structure in the brain. It provides connections between the right and left ICA systems through the anterior communicating artery. In addition, the posterior communicating arteries (PCoA) connect the ICA system (anterior circulation) to the vertebrobasilar system (posterior circulation). It has the shape of an irregular polygon and is located mainly at the base of the frontal lobes. There are numerous anatomic variations of the circle of Willis, including agenesis or hypoplasia of certain components (see pp. 135-136 in Ref. 9). Under normal conditions, a hemodynamic balance exists between the anterior and posterior circulation and between the right and left carotid circulation. A change in the balance, as seen with occlusive cerebrovascular disease, causes redistribution of blood through the circle of Willis. The complexity of these hemodynamic imbalances is responsible for the high frequency of aneurysm formation along branches of the circle of Willis.
There are numerous leptomeningeal anastomoses that form on the surface of the brain and that are important in the setting of large artery occlusions. They occur in every major arterial territory. For example, a middle cerebral artery (MCA) occlusion may activate anastomoses between leptomeningeal branches of the MCA and the leptomeningeal branches of the anterior cerebral artery (ACA) or posterior cerebral artery (PCA). Another example is the anastomosis between the splenial artery (off the PCA) and the pericallosal artery (off the ACA) after occlusion of the ACA or PCA. These collaterals are seen on the lateral surface of the cerebral hemisphere. Connections between the anterior and posterior choroidal arteries occur in the choroid plexus of the lateral ventricle.
The main reason for concern about cerebral aneurysms is the consequences of their rupture and resultant subarachnoid hemorrhage (SAH). The most common cause of SAH is trauma; however, this discussion will focus on aneurysmal SAH. Cerebral aneurysms can be broadly classified by size, location, shape, and etiology. The causes of their formation and rupture are not well known. Unless noted otherwise, most of this discussion will focus on saccular lesions, which are the most common aneurysms by far. Once thought to be congenital, it is widely accepted that these lesions develop later in life.
Histological examination of saccular intracranial aneurysms reveals an absent or discontinuous internal elastic lamina, absence of the media at the aneurysm neck, deficient endothelium in the aneurysm lumen, and atherosclerotic changes in the parent vessel at the origin of the aneurysm. The walls of most small aneurysms are composed of discontinuous endothelium surrounded by fibrous adventitial tissue and no smooth muscle. Large (diameter between 1.0 and 2.5 cm) and giant aneurysms (≥2.5 cm diameter) may have more organized walls consisting of fibrous tissue, foam cells, and deposits of calcium.14,15 It is believed that aneurysms form over a relatively short period of time, and either they rupture or they stabilize and remain unruptured.16
The reasons for aneurysm development and rupture are not known completely. It has been postulated that aneurysm formation may be related to the intimal cushions and defects of the media at the branching points described above,17 but this has not been proven. Destruction of the internal elastic lamina seems to be the crucial event for aneurysm formation and is likely an early event that weakens the arterial wall.4 Collagen also appears to play a role in aneurysm pathology. Part of the stabilization of the aneurysm is exuberant collagen formation, which leads to thickening of its wall. In fact, unruptured aneurysms have almost twice the collagen content of normal arteries. It is not known if this increased-collagen formation is a compensatory mechanism for the internal elastic lamina loss. In contrast, ruptured aneurysms have decreased and poorly organized collagen content.18 Unlike normal arteries and unruptured aneurysms, ruptured lesions have a disrupted endothelial lining. Therefore, it has been proposed that endothelial injury may contribute to aneurysm formation and rupture.19,20
The ultimate source of the destruction of the internal elastic lamina and endothelium remains unknown, but an underlying inflammatory cause was proposed as early as the mid-19th century by Virchow.19 T cells (natural killer cells) and macrophages have been found in both ruptured and unruptured aneurysms.19 Additionally, lysosomal granule deposition and increased metalloproteinase activity has been observed within aneurysm walls.21 These and other findings suggest at least a link between inflammation and aneurysm formation and, ultimately, rupture.19 Because inflammatory changes are obligatory features of atherosclerosis,22 aneurysm development may be part of an atherosclerotic spectrum in the parent vessel.
Debate continues regarding whether intracranial aneurysm pathogenesis has a genetic, acquired, or combined etiology. Support for a genetic mechanism comes from the association of aneurysms and several inheritable connective tissue disorders. These include autosomal dominant polycystic kidney disease, type IV Ehlers-Danlos syndrome (EDS) (see below), Marfan’s syndrome, α1-antitrypsin deficiency, neurofibromatosis type 1, and tuberous sclerosis—to list only a few.23,24 The true incidence of aneurysms caused by these disorders is unknown because of their variable phenotypes. However, it seems these diseases are responsible only for a small percentage of aneurysms.
Familial intracranial aneurysms have been described for several decades. However, the pattern of inheritance is unknown. Some studies have shown up to a fivefold increase in aneurysm or SAH incidence in first-degree relatives of patients harboring intracranial aneurysms.25,26 Regardless, the evidence has been sparse, and screening for asymptomatic intracranial aneurysms is not well defined. The yield of screening first-degree relatives and their lifetime risk of SAH are low when only one family member harbors an aneurysm.27,28 The authors’ strategy has been to evaluate first-degree relatives with CT angiography or MR angiography in families with two or more affected members. This is based on evidence that the yield from such screening is roughly 10%.29,30 If the initial screening fails to reveal an aneurysm, we repeat a noninvasive study every 5 years in order to detect any de novo lesions.
Acquired risk factors are not well defined for SAH. Cigarette smoking is the one consistent factor identified in all studies to date. The estimated risk of SAH is up to 10 times higher in smokers than in nonsmokers. Smoking accelerates intracranial aneurysm growth, and continued smoking may increase the risk of de novo aneurysm development after an initial SAH.31,32,33,34 Many reports have demonstrated the role of hypertension in increasing aneurysm development and rupture.34,35 However, this risk does not seem to be as high as with smoking. While low-level alcohol consumption may lower SAH risk, high-level consumption and binge drinking seem to increase the risk.33
Annual incidence rates of SAH are estimated between 9 and 15 cases per 100 000 persons.36,37 This translates into roughly 30 000 cases of SAH in North America annually. However, there are considerable global variations in these figures. For example, a Finnish study reported an incidence as high as 30 per 100 000 person-years.38 In contrast, a French study reported an incidence of 2.2 per 100 000.39 In most studies, women have a 1.5 times greater incidence of SAH than men, with hormonal factors implicated for the gender inequity.40 Unlike other types of stroke, SAH is unique with its female predilection. Studies from the United States have shown a more than two times greater risk for SAH in blacks than in whites.41,42 The peak incidence of SAH is between 55 and 60 years of age. Indeed, our experience parallels the above figures in that the typical patient with SAH is a middle-aged female smoker.
The true prevalence of intracranial aneurysms remains unknown and is difficult to determine. It has been estimated at 5% on the basis of autopsy studies.43 On the other hand, incidental aneurysms were detected in only 1% of cases during a random review of cerebral angiograms.44 Not surprisingly, these studies are criticized for selection bias (e.g., what was defined as an aneurysm) and patient population. A prevalence of approximately 2% is generally assumed, as suggested by a contemporary meta-analysis.45
The outcome from aneurysmal SAH is dismal.46 Approximately one-third of patients with a first SAH will die in the acute phase. One-third will remain with significant neurological disability. Only the remaining third will recover well from the hemorrhage and its sequelae.37 Although there are some variations in these statistics from other studies, the above figures serve as easy reminders that the overall prognosis for SAH remains poor despite advances in initial resuscitation, aneurysm treatment, critical care, and treatment of cerebral vasospasm. Predictors of a good recovery from SAH include young age and good neurological function at presentation.40
Given the gravity of SAH, knowledge of the natural history of cerebral aneurysms is important to understand the effects of treatment. In this section, the discussion has been limited to the known natural history of unruptured aneurysms. Knowing the natural history is particularly germane now that more aneurysms are being discovered with noninvasive imaging. The natural history of the aneurysm and the life expectancy of the patient must be matched against the risks of treatment.
Unfortunately, the natural history of intracranial aneurysms is not well established. Historically, the risk of rupture of an aneurysm was believed to be 1% to 2% per year. Of course, this risk can vary, depending on the patient and the aneurysm. Any contemporary discussion on the natural history must include the International Study of Unruptured Intracranial Aneurysms (ISUIA).47,48 For this discussion, we focus on the ISUIA because of its influence on current practice patterns. The ISUIA was the largest systematic study undertaken to evaluate the natural history of unruptured aneurysms and to evaluate treatment risk. The study contained two parts. The first part, published in 1998, had both retrospective and prospective components.47 In the retrospective cohort, 1937 aneurysms in 1449 patients from 53 centers were evaluated. These patients were subdivided into two groups:—those with previous SAH from another aneurysm and those without previous SAH. In the prospective arm, the surgical outcomes of unruptured aneurysms in 1172 patients were analyzed. For patients without previous SAH and with aneurysms <10 mm in size, the risk of rupture was 0.05% per year. Aneurysms <10 mm in patients with previous SAH had a rupture risk of 0.5% per year. Aneurysms >10 mm had a rupture risk of 1% per year in both groups. These rupture risks were much less than previously thought. The surgical morbidity of 15.7% was also much higher than reported in previous studies.47 One major criticism of this study was that there was significant selection bias toward “low-risk” aneurysms because the “high-risk” lesions were selected out for treatment. In other words, the study did not represent all unruptured aneurysms, and therefore the unusually low rupture rates could not be applied to all lesions.49,50
The second part of the study48 was published in 2003 and is ongoing. This part included 4060 patients, of whom 1692 patients harboring 2686 aneurysms were observed and 2368 atients were treated with surgery or coiling. Of the treated group, 1917 patients underwent surgery and 451 were treated with coiling. The risks associated with surgical and endovascular treatments were still higher than in previous reports at 12.6% and 9.8% at 1 year, respectively. Predictors of poor outcome from treatment included increased patient age, lesion diameter >12 mm, and aneurysm location in the posterior fossa. The rupture risk was 2.5% over 5 years for lesions involving the posterior circulation in patients with no previous SAH and 0% over 5 years for anterior circulation lesions. On the other hand, patients with previous SAH had 5-year rupture risks of 1.5% in the anterior circulation and 3.4% in the posterior circulation. The authors of the ISUIA proposed that there was no benefit in treating aneurysms <7 mm in size in patients with no previous SAH because of the low risk of rupture (0.1% per year) and the relatively high risk of treatment.48 Once again, this part of the study has been criticized for selection bias because 534 of the 1692 patients selected for observation ultimately crossed over to treatment owing to concerns of rupture risk.50
One natural history study cited frequently to challenge the ISUIA is from Finland.51 Most of the 142 patients in this study had previously suffered SAH and were followed without treatment for a mean of 19.7 years.51 The annual incidence of rupture was approximately 1.3% in this cohort. Despite the criticisms of ISUIA and the debate over the exact aneurysm rupture risks, it seems the rupture risk is much less than previously thought. Furthermore, the clinical impact of the ISUIA cannot be disputed. Consequently, we have noted that a larger number of incidental aneurysms are being managed conservatively.
It is widely recognized that a severe headache of sudden onset is a hallmark of aneurysmal SAH. The phrase “worst headache of my life” has become almost synonymous with aneurysm rupture. The headache is usually global and radiates to the neck and is often accompanied by nausea and vomiting. The patient typically has nuchal rigidity and photophobia. Transient loss of consciousness may be seen in one-third of patients. Seizures may be associated with SAH in 5% to 8% of patients. Focal neurological deficits may be seen from mass effect from large and giant aneurysms (see below). Cranial neuropathies may occur from compression by these aneurysms. For example, 30% of patients with ophthalmic artery aneurysms can have visual field deficits or monocular blindness.52 Third cranial nerve palsies can occur with PCoA or basilar apex aneurysms. Posterior fossa aneurysms may cause tinnitus, trigeminal neuralgia, hemifacial spasm, facial weakness, or dysphagia from cranial nerve compression.
CT scanning usually confirms the diagnosis of SAH. Although the sensitivity of modern CT imaging exceeds 95% on the day of the ictus53 and it decreases to 50% after 1 week and 30% by 2 weeks. CT is also important in the diagnosis of hydrocephalus, which occurs in 20% of patients with SAH or an associated intracerebral hemorrhage (ICH). The hydrocephalus may account for an initially poor grade on the neurological examination. If the clinical suspicion is high but CT fails to demonstrate SAH, a lumbar puncture is mandatory for diagnosis. Xanthochromia is the hallmark of SAH on lumbar puncture and occurs from lysis of erythrocytes in the CSF. Lumbar puncture is considered the gold standard for the diagnosis of SAH. However, at least 6 hours and preferably 12 hours should elapse after symptom onset to ensure the presence of xanthochromia and to reduce the chance of a false-negative result. Traumatic lumbar punctures can usually be differentiated from SAH. Bloody CSF that does not clear between the first and fourth tubes is highly suspicious of SAH. Furthermore, xanthochromia is absent in traumatic lumbar punctures, provided the sample is analyzed immediately.
In addition to neurological concerns, systemic manifestations of SAH are important. Cardiac abnormalities include arrhythmias, cardiac “stunning” with transient ventricular dysfunction, or myocardial infarction. Pulmonary disorders include aspiration pneumonia from decreased level of consciousness, neurogenic pulmonary edema, and bronchospasm.54 Electrolyte disturbances are also seen, the most common being hyponatremia from cerebral salt wasting. The hypovolemia resulting from salt wasting may exacerbate cerebral vasospasm.
Once SAH is diagnosed, the aneurysm must be identified. This is classically done with cerebral angiography, which identifies the lesion in 85% of all SAH cases. If the first angiogram is unremarkable and the presence of an aneurysm is suspected, angiography is repeated in 1 week. CT angiography has been increasingly used to diagnose or exclude aneurysms, and its sensitivity has increased with improved multidetector, multislice technology that improves spatial and temporal resolution. The sensitivity of CT angiography is proportional to the size of the aneurysm. CT angiography sensitivities of 100% for aneurysms >4 mm, 94% for aneurysms 2 to 4 mm, and 50% for aneurysms <2 mm have been reported recently.55 The accuracy of detecting aneurysms by CT angiography has been also been reported recently, with sensitivity of 98% and specificity of 100% in the setting of SAH.56
The most common locations for aneurysms in the anterior circulation are the anterior communicating artery, the ICA, and the MCA. Collectively, the ICA aneurysms, which include those at the ophthalmic artery, superior hypophyseal artery, PCoA, anterior choroidal artery, and at the ICA terminus, account for 40% of all aneurysms. Aneurysms of the PCoA alone account for half of the ICA aneurysms. Aneurysms of the MCA comprise 20% to 25% of all intracranial aneurysms.
In the posterior circulation, the basilar artery (BA) bifurcation is the most common location, accounting for 8% of all intracranial aneurysms. The posterior inferior cerebellar artery is the second most-frequent location, with 3% arising from this location. Less common are basilar trunk aneurysms and peripheral aneurysms off the major branches of the BA.
For completion, the authors mention intracavernous carotid artery aneurysms, i.e., aneurysms that arise from the ICA segment that lies within the cavernous sinus. These aneurysms are, by definition, extradural. Therefore, SAH from these lesions is rare unless part of the aneurysm protrudes into the subarachnoid space. Rupture of these aneurysms can cause a fistula between the ICA and the cavernous sinus. Life-threatening epistaxis may occur if the aneurysm ruptures directly into the adjacent sphenoid sinus. More commonly, unruptured aneurysms in this location become symptomatic by causing retroorbital pain, diplopia, or facial numbness. Diplopia and altered facial sensation result from cranial nerve (third, fourth, fifth, and sixth nerves) compression within the cavernous sinus.57
As mentioned, giant aneurysms are ≥2.5 cm in diameter (Figure 23-3). These are fortunately rare lesions, and only 3% of intracranial aneurysms are in this category. The risk of rupture of these aneurysms is high, and one-third of patients harboring these lesions present with SAH. The remaining patients present with symptoms and signs from mass effect such as headache, seizures, altered mental status, or focal neurological deficits. Frequently, there is edema around these lesions, and their effects are similar to those of tumors. Many of these lesions contain thrombus as a result of turbulent blood flow, and the aneurysms may manifest with thromboembolism. Cerebral angiography may reveal only partial filling of the aneurysm because of thrombus, and MR imaging or CT scanning is necessary to delineate the true size of the lesion.
FIGURE 23-3.
Giant cerebral aneurysm. A 49-year-old woman presented with chronic headaches and was found to have (A) a giant left MCA aneurysm with a relatively wide neck. A three-dimensional view of the left ICA injection (AP projection) shows that the superior (S) and inferior (I) M2 divisions can be separated from the (B) aneurysm neck. Unlike the case with most MCA aneurysms, endovascular treatment of this lesion was undertaken. A Neuroform (Boston Scientific Target, Fremont, CA) stent was placed in the superior M2 division, followed by packing of the aneurysm with coils. (C and D) The final views showed minimal aneurysm neck residual with preservation of the M2 divisions.

Pediatric aneurysms are rare and account for no more than 2% of all intracranial aneurysms.58,59 Underlying disorders are present in more than one-half of these cases.59 Infective causes such as endocarditis and sepsis were responsible for 25% of pediatric aneurysms in one series.60 Although giant and fusiform aneurysms are rare in adults, a higher percentage of these complex lesions are found among infants and children.58,61
Infectious aneurysms, historically known as mycotic aneurysms, are uncommon and account for approximately 2% to 4% of all intracranial aneurysms.62 They are usually found in association with infective endocarditis and subsequent septic embolization. Bacterial aneurysms tend to form distally in the cerebral circulation, most commonly in the distal MCA. They can form rapidly (within days) and develop from inflammatory necrosis of the vessel wall, starting in the adventitia and extending into the media. The weakened arterial wall then leads to aneurysm formation.63 Consequently, these lesions do not have a well-defined neck. The incidence of clinically significant aneurysms within the setting of endocarditis can be as high as 5.4%.64 These lesions can also develop from local spreading of an infection such as sinusitis, skull base osteomyelitis, or meningitis. In the setting of endocarditis, the infective organism is usually identified on blood culture. The most commonly identified bacteria are Staphylococci and Streptococci. Endocarditis must be included in the differential diagnosis of a febrile patient with neurological symptoms and signs. Infectious aneurysms are very friable and carry a high rupture rate. Either SAH or ICH occurs in 50% of patients.62 The high morbidity and mortality from infectious aneurysms are caused by their high rupture rate and the severity of the underlying systemic illness. Fortunately, serial angiograms demonstrate that up to 50% of these aneurysms resolve or decrease in size with antibiotic therapy.65 However, selected lesions require treatment, usually in the form of surgical or endovascular parent vessel sacrifice. Because these aneurysms are friable, direct clipping or coiling is not usually feasible. If parent vessel sacrifice is not possible, excision of the lesion and bypass may be necessary.
Traumatic aneurysms are even less common than infectious ones. They represent less than 1% of all aneurysms.66,67 Most are associated with blunt head trauma and skull fractures, although 3.5% of patients with penetrating trauma have these lesions, particularly with low-velocity projectile injuries.68 Traumatic aneurysms may also be iatrogenic lesions resulting from craniotomies or skull base procedures. Their location may be divided into the peripheral cortical arteries or the large skull base arteries. The peripheral traumatic aneurysms typically involve the MCA distribution, while the basal traumatic lesions affect the cavernous, petrous, or supraclinoid ICA.66 The distal ACA is also a fairly common location because of brain contusion against the falx cerebri. Damage to the internal elastic lamina may predispose to traumatic aneurysm formation. While the walls of saccular aneurysms are composed of intima and adventitia, those of traumatic aneurysms can be one of three types: true, false, or mixed.69 True traumatic aneurysms result from partial arterial wall disruption caused by blunt injury. The trauma damages the intima and media while leaving the adventitia intact. In contrast, false aneurysms result from disruption of the full thickness of the artery wall, usually in the form of an arterial laceration. The false aneurysm does not contain any of the normal arterial elements; rather it is simply an organized thrombus formed after remodeling of the resultant hematoma from the laceration.69 False aneurysms seem to be unstable lesions. Indeed, 50% of these aneurysms rupture within 2 weeks of the trauma. Mortality approaches 50% after rupture of these aneurysms.70 The most common presentation of a traumatic aneurysm is a delayed deterioration following head trauma. Delayed, acute ICH should raise the suspicion of a traumatic aneurysm. Like infectious aneurysms, these lesions tend to arise from the distal cerebral arteries. Direct treatment with clipping or coiling is difficult, and parent vessel sacrifice is usually necessary. Endovascular placement of stents to span the injured region of the artery has emerged as an attractive option to reconstruct the vessel and avoid sacrifice of the parent artery.71,72
As mentioned above, the natural history for small intracranial aneurysms appears to be more benign than previously thought. The consequence is that many unruptured aneurysms are not treated and are followed radiographically. In this section, current therapeutic options for intracranial aneurysms are discussed. These are broad generalizations, and the nuances of surgical and endovascular treatment depend on the location, size, and morphology of the individual aneurysm and its relationship to other neurovascular structures. The goal of aneurysm therapy is to prevent rupture, or in the case of ruptured aneurysms, to prevent rehemorrhage. The traditional method has been open surgery and clipping of the aneurysm. The outcomes from aneurysm surgery have been optimized over the past four decades with the introduction of the operative microscope, development of microneurosurgical techniques, improvements in anesthesiology and intraoperative cerebral protection, and advanced neurocritical care. Over the past 15 years, endovascular therapy has emerged as a safe and effective method of treating certain aneurysms. The application of endovascular techniques has expanded significantly with the introduction of balloon- and stent-assisted coiling.
Surgical clipping provides essentially a permanent cure if complete obliteration is achieved. Even incompletely clipped aneurysms seem to have very low rupture rates. Long-term angiographic follow-up showed asymptomatic recurrence in only 2 of 135 lesions in one series of completely clipped aneurysms.73 Additional benefits of surgery are the ability to reconstruct the parent vessel with clips, evacuation of any associated intracerebral hematoma from a ruptured aneurysm, and immediate collapsing of an aneurysm after clipping. The disadvantages of surgery include the potential need for brain retraction, prolonged general anesthesia during the procedure, potential injury to cranial nerves and arterial branches during dissection of the lesion, intraoperative aneurysm rupture, and prolonged convalescence after surgery. The 30-day morbidity and mortality rates associated with surgery for unruptured aneurysms range from 1% to 7% and from 4% to 15%, respectively.74,75
Coil embolization does not require craniotomy or brain retraction; aneurysm microdissection is also not necessary. Coiling involves microcatheterization of the aneurysm and packing the lesion with coils (Figure 23-4). The procedure is relatively safe with a mortality rate of less than 1% and a permanent morbidity rate of less than 10%.76 However, incomplete aneurysm occlusion is more common with coiling than with surgery. Unlike clipping, endovascular treatment frequently results in aneurysm recanalization, especially in wide-necked and large aneurysms. These recanalization rates approach 30%,77,78 although the impact of recanalization on hemorrhage rates is not clear (Figure 23-5). Even complete occlusion of the aneurysm may still result in recanalization from coil compaction. Therefore, one inherent disadvantage of coiling is the need for periodic follow-up evaluation and potential retreatment. Certain aneurysm morphologies, namely, wide-necked and large-sized lesions, present endovascular challenges as a result of the greater chance of coil herniation into the parent vessel. Some of these concerns have been addressed with balloon- and stent-assisted coiling and with three-dimensional coils that conform to the shape of the aneurysm. However, increased endovascular manipulation raises the chances of thromboembolic complications. The potential need for anticoagulants and antiplatelet agents during and after the endovascular procedures may confound adjunctive procedures, such as ventriculostomy, and other bedside procedures necessary in the setting of SAH.
FIGURE 23-4.
Cerebral aneurysm. This 66-year-old man was found to have an incidental left ICA terminus aneurysm during a workup for minor head trauma. (A) An oblique view of the left ICA injection shows that the aneurysm has a slightly wide neck. The microcatheter is in a stable position within the aneurysm fundus. (B) Three-dimensional coils were used, and the coiling procedure was performed uneventfully. (C) A final anteroposterior fluoroscopic view shows good packing of the lesion without herniation of coils into the ACA or MCA.

FIGURE 23-5.
Aneurysm recanalization. This 41-year-old man underwent coil embolization of a ruptured basilar tip aneurysm at an outside hospital and made a good recovery. He presented for follow-up evaluation and was found (by digital subtraction angiography [DSA]) to have significant recanalization of this (A) wide-necked aneurysm. (B) He underwent placement of a stent that spanned the distal BA and the right PCA (unsubtracted view). (C [DSA] and D [unsubtracted view]) The aneurysm was then coiled without herniation of the coils into either PCA (stent markers are circled).

Historically, poor-grade patients with ruptured aneurysms or inoperable lesions were selected for endovascular therapy. Posterior circulation aneurysms, particularly basilar tip aneurysms, are associated with higher surgical morbidity. The surgical mortality–morbidity rate approaches 15% to 20% for basilar tip aneurysms.79 In contrast, MCA aneurysms are better suited for surgical clipping because of their relatively wide necks and close association of important branches that are at high risk for occlusion during endovascular therapy. As the experience with endovascular therapy has grown over the past decade, a selection bias has developed at many centers. Most patients in poor medical or neurological condition and many patients with posterior circulation aneurysms are treated with coiling, whereas patients with MCA aneurysms are referred for surgery. A fair appraisal of endovascular therapy must discriminate experience from the early 1990s to contemporary experience in this decade. This is a reflection of the rapid advancements in endovascular technology. For example, a comparison of these two eras showed procedure-related morbidity rates of 9.1% from 1990 to 1995 and 4.8% from 1996 to 2002.80
To compare surgery against endovascular therapy, each treatment approach for ruptured and unruptured aneurysms has been considered here. The ISUIA and its impact on neurovascular practice have been discussed previously. The other major study that has shaped current aneurysm therapy is the International Subarachnoid Aneurysm Trial (ISAT).81 ISAT was the first large-scale, randomized study comparing surgery and coiling for ruptured aneurysms. The study was designed to randomize patients with ruptured aneurysms thought to be suitable for either treatment. The trial was conducted mostly at European centers and demonstrated an absolute risk reduction of morbidity and mortality at 1 year of 6.9% in favor of endovascular therapy. The study was criticized for heavy selection bias because only 24% of patients enrolled were actually randomized.82 The majority of randomized patients were in good clinical condition, and MCA and posterior circulation aneurysms were rare. The randomization obviously reflected inherent practice patterns described previously. Nonetheless, ISAT has provided level I evidence in support of endovascular therapy for certain ruptured aneurysms. It reiterates the notion that durability of treatment is not the immediate concern in SAH and that coiling offers improved short-term outcomes.
In contrast, the goal of treating an unruptured aneurysm is durable prevention of SAH. No prospective, randomized data is available for the treatment of unruptured aneurysms. In one review of 2500 patients, a morbidity rate of 18.5% was reported for surgical cases versus 10.6% for endovascular therapy.83 Mortality rates in the same study were 2.3% versus 0.4% in favor of coiling. In the second part of ISUIA, 1-year surgical and endovascular morbidity rates of 12.6% and 9.8%, respectively, were reported.48 However, this trial was not randomized, and many aneurysms were selected out for observation (see above).
Cerebral vasospasm classically refers to a transient, self-limited constriction of cerebral arteries several days after exposure to subarachnoid blood, most frequently caused by rupture of a cerebral aneurysm. Cerebral vasospasm can refer to angiographic narrowing of cerebral blood vessels after SAH, neurological deficits caused by secondary ischemia from the resulting diminished blood flow, or both. Angiographic vasospasm is seen within 3 to 4 days of SAH, peaking in incidence and severity by day 6 to 8, and generally resolving by day 12.84,85 Approximately 67% of patients demonstrate some degree of angiographic vasospasm in the second week after SAH,86 with moderate-to-severe (vessel lumen narrowed by >25% of its original diameter) angiographic vasospasm seen in 30% of patients.87,88 Those with more severe hemorrhage suffer from an increased incidence of severe vasospasm, with >50% narrowing seen in 55.9% of grade III to V patients.89 Although vasospasm >50% is generally required before flow restriction results in clinical ischemia,90 other factors, such as location of spasm, collateral supply, and cerebral perfusion pressure, also influence whether ischemic symptoms become manifest. As a result, the incidence of clinical vasospasm is approximately half that of angiographic vasospasm and can be seen in up to one-third of patients.86,89,91
Extensive evidence points to the presence of subarachnoid blood clot as the chief etiology in cerebral vasospasm. The amount and location of subarachnoid blood predict the subsequent site and degree of cerebral vasospasm,92,93 as does the rate of clearance of subarachnoid clot via endogenous means.94 Hemoglobin within red blood cells is a key spasmogen,95,96 although other components of the subarachnoid blood clot also contribute to vasospasm.97 The underlying pathophysiology appears to be prolonged contraction of vascular smooth muscle cells rather than fibrosis or cellular proliferation,98,99,100 as also evidenced by the ability of intra-arterial (IA) vasodilators such as papaverine and nicardipine to (at least transiently) ameliorate vasospasm.
Prophylaxis against cerebral vasospasm with oral nimodipine is considered standard of care in the treatment of SAH, because it significantly improves the odds of a good outcome and reduces death and disability owing to vasospasm.101,102,103,104 However, once cerebral vasospasm becomes clinically apparent by causing neurological deficit(s), immediate/first-line therapy has traditionally involved the institution of so-called “triple-H” (hypertension, hemodilution, hypervolemia) therapy with use of increased fluids and/or pressors, particularly when access to endovascular treatment is limited or unavailable. Of the three components, induced hypertension is likely the most important,105 with the common denominator of this approach being an increase in cerebral perfusion pressure, thereby enhancing both flow through a vasospastic segment and also collateral supply to the distal ischemic brain. Although never subjected to a randomized trial, outcomes with such treatment appear to be significantly better than the natural history of untreated vasospasm.103,106
Endovascular therapy for symptomatic cerebral vasospasm is generally undertaken after medical intervention has been deemed unsuccessful. It is important to rapidly maximize medical therapy to determine its efficacy or lack thereof and subsequent need for endovascular treatment because a delay in decision making can lead to fixed rather than reversible cerebral ischemic deficits. Endovascular options may be broadly categorized as IA infusion of vasodilators or balloon angioplasty. The first and most frequently used IA vasodilator drug is papaverine, initially described by Kaku et al.107 and Kassell et al.108 in 1992 for the treatment of vasospasm after aneurysmal SAH. Effects of papaverine are unfortunately short-lasting and include the potential side effects of raised intracranial pressure (ICP) and seizures, prompting renewed interest in IA calcium 2+ channel blockers, such as nicardipine109 or verapamil,110 or the treatment of vasospasm. Although side effects of IA calcium 2+ blockers (raised ICP in particular) are less frequent, their short-term effect has led to the adoption of balloon angioplasty, introduced in 1984 by Zubkov et al.111 for symptomatic cerebral vasospasm. Current techniques with over-the-wire balloons provide a long-lasting, relatively safe treatment for vasospasm, although technical challenges diminish successful application in the ACA as a result of vessel angulation and tortuosity.112,113 IA vasodilators still play a role in the treatment of distal vasospasm (inaccessible to balloon angioplasty) or in the pretreatment of very severe vasospasm in order to permit navigation by angioplasty balloons.
The term “stroke” is all encompassing and includes ischemic stroke, ICH, and SAH. Annually, 15 million people worldwide suffer a stroke.114 Of these, one-third die and one-third are permanently disabled.114 Although the incidence of stroke is declining in industrial countries because of improved control of hypertension and smoking, the absolute numbers of strokes is increasing because of the aging population in these countries. Stroke is the third-leading cause of death in developed countries behind cardiovascular disease and cancer. In 2002, 10% of all deaths worldwide were attributed to stroke.114 Ischemic strokes account for 87% of all strokes. Each year, approximately 700 000 people in the United States suffer a stroke.115 Of these, 500 000 are first-time strokes. The estimated cost of stroke in the United States will exceed $62 billion in 2007.115
The hallmark feature of stroke is the sudden onset of neurological symptoms and signs. Focal neurological symptoms such as diplopia, weakness on one side, loss of sensation, dysarthria, aphasia, and visual field deficits are suggestive of ischemic stroke. In contrast, presentations of severe headache, seizures, alterations in level of consciousness, and vomiting are more likely to reflect hemorrhage.
Ischemic stroke can be classified by the type of vascular lesion or by the mechanism of ischemia. Numerous mechanisms may cause brain ischemia. Severe arterial stenosis or occlusion from atherosclerosis or thrombus may reduce perfusion in a purely hemodynamic fashion. Embolism may originate from a more proximal source and result in arterial occlusion. The small end-arteries in the deep gray matter may be affected by local atherosclerosis or lipohyalinosis. Miscellaneous causes of ischemic stroke include hypercoagulability, arterial dissection, arterial vasospasm, vasculitis, fibromuscular dysplasia, and moyamoya disease. Of all these causes, the four most frequent are large-vessel atherosclerosis, cardioembolism, small-vessel disease, and cryptogenic.116 Extracranial large-arterial atherosclerosis, which is responsible for up to 40% of all ischemic strokes, is beyond the scope of this chapter. Intracranial atherosclerosis will be addressed later in this chapter.
Cardioembolism accounts for 15% to 30% of all ischemic strokes.116 The most common cardiac sources of embolism include valvular disease such as mitral stenosis or regurgitation, endocarditis, mural thrombus following anterior myocardial infarction, left atrial appendage from atrial fibrillation, severe cardiomyopathies, and ventricular aneurysms. Less common is embolism from atrial myxoma or paradoxical right to left embolism from a patent foramen ovale. A cardiac source is presumed when multiple infarcts involving more than one cerebral vascular territory are seen clinically or radiographically. Most embolic obstruction is cleared automatically within 48 hours through the homeostatic processes of recanalization and fibrinolysis.
Lacunar strokes also account for 15% to 30% of all ischemic strokes. These strokes involve the small vessels supplying the deep structures of the brain such as the basal ganglia and thalamus. These penetrating arteries can become stenotic or occluded by microatherosclerosis and lipohyalinosis. Uncontrolled hypertension and diabetes mellitus are often implicated with these strokes. Because these vessels are less than 400 μm in diameter, angiography is often unremarkable. Most lacunar strokes are diagnosed clinically with pure motor or sensory syndromes. MR imaging may demonstrate a small, deep infarct.
In this section, the characteristics of specific large-vessel occlusions are outlined in the broadest terms. More specific descriptions can be found in standard neurology texts.
Occlusion of the main trunk of the MCA causes contralateral hemiparesis, contralateral anesthesia, ipsilateral gaze preference, and contralateral hemianopsia. If the dominant cerebral hemisphere is involved, the patient will also suffer from global aphasia. Involvement of the nondominant hemisphere leads to impaired spatial perception. Less severe combinations of these symptoms may occur, depending on occlusions of distal branches rather than the main trunk.
Infarction in the ACA territory typically results in paresis and anesthesia in the contralateral lower extremity. Motor neglect and disinclination to use the contralateral extremities may be observed. Bilateral ACA infarction is particularly devastating with resultant behavior and personality changes, severe apathy, incontinence, and muteness. These features are attributed to involvement of both the medial frontal lobes and the limbic structures.
Occlusion of the PCA usually causes a contralateral homonymous hemianopsia caused by injury to the occipital lobe. Proximal PCA occlusion may also injure the thalamus and midbrain because of the involvement of perforating branches. This typically results in contralateral loss of sensation, altered mental status, and impaired vertical gaze.
There are many brainstem infarction syndromes depending on the level (midbrain, pons, or medulla) and location in the brainstem. For interventional purposes, we have limited this discussion to vertebrobasilar occlusion. Rather than describing the dozens of brainstem syndromes, we will focus on what happens when the VA or BA itself are occluded. Occlusion of the BA or VA is more often the cause of brainstem infarction than occlusion of perforating branches.117 The posterior circulation is easy to overlook as a source of stroke because of the wide spectrum of presentations, many of which are nonspecific. However, failure to recognize a VA or BA occlusion may have disastrous consequences. The posterior circulation should be considered in patients with bilateral or crossed (e.g., ipsilateral face and contralateral limb) motor and sensory signs, disconjugate eye movements or nystagmus, dysmetria and ataxia, involvement of cranial nerves, and altered levels of consciousness.
Classically, malignant infarction is described for large strokes involving the MCA. It is an emergency that carries a rate of mortality six to eight times higher than seen in other ischemic strokes. By definition, more than 50% of the MCA territory is involved with subsequent brain edema that may result in elevated ICP and eventual herniation and death.118 Malignant MCA infarction may account for up to 10% of all ischemic strokes.118 Besides the focal neurological deficit caused by the stroke itself, a progressive decline in the patient’s level of consciousness is seen at approximately 48 hours after the onset of the stroke. In young patients with no baseline brain atrophy, clinical deterioration may begin as early as 12 hours from the onset.119 Despite maximal medical management of intracranial hypertension, including osmodiuresis, hypothermia, and barbiturate coma, the mortality approaches 80%.120
Mechanical decompression of the edematous cerebral hemisphere through a hemicraniectomy has been performed for decades in numerous settings. Hemicraniectomy is very effective at reducing ICP and may be life-saving. Until recently, level I evidence supporting this procedure has been lacking. Controversial issues in the setting of stroke have been timing of hemicraniectomy and performance of this operation on left-sided lesions. The historical argument against left hemicraniectomy has been that survivors will remain globally aphasic and hemiplegic. In contrast, right-sided lesions may be more compatible with a functional outcome because of the absence of language involvement. One retrospective analysis concluded that hemicraniectomy reduced the mortality from malignant stroke to 24% and that patients with left-sided strokes had similar outcomes to those with right hemispheric strokes.121 Additionally, patients younger than the age of 50 years seemed to have better outcomes. It has also been proposed that early (<24 hours from onset) hemicraniectomy improves outcomes.122
The effect of decompression on functional outcomes in these malignant strokes has been evaluated in three randomized controlled European trials.123 These studies had similar design, and their data were pooled for analysis in a recent publication.123 Two of the three trials were halted because the results showed a significant benefit of surgical decompression on mortality. The pooled analysis demonstrated a clear beneficial effect on survival and unfavorable outcome. However, these benefits were driven by an increase in patients with major disability. Therefore, the net benefit of surgical decompression must still be individualized.124 The preliminary analysis did not show any significant difference in outcomes in patients decompressed within 23 hours versus 48 hours.123
The other classic surgical emergency occurs with large posterior fossa strokes. Although the stroke burden is small compared to that associated with a large MCA stroke, the posterior fossa is a much more confined space with little tolerance for brain edema. Mass effect in the posterior fossa can lead to compression of the fourth ventricle and hydrocephalus and eventual brain stem compression. These patients tend to improve with posterior fossa decompression in the form of suboccipital craniectomy.125 These patients have survivable deficits (usually ataxia or dysmetria from cerebellar involvement), and their outcome is favorable if decompression is performed at the earliest signs of deterioration.
Medical management of ischemic stroke may be classified by its time course as emergent, thrombolytic, supportive, rehabilitative, and finally, preventative. Emergent and supportive measures are best reviewed in the recent guidelines from the AHA126 and are summarized in Table 23-1 (rehabilitative and preventative treatments are outside the scope of this chapter). Medical thrombolytic treatment is primarily reviewed here, in contrast to interventional treatment, which is discussed in the subsequent section. The ischemic stroke imaging section follows both the medical and endovascular therapy sections because the reader will then know why we image and what we image for and will be aware of the therapeutic implications of the imaging findings.
|
On the basis of the first successful randomized controlled trial of intravenous (IV) thrombolysis for acute stroke, the National Institute of Neurological Disorders and Stroke (NINDS) trial,127 administration of 0.9 mg/kg recombinant tissue plasminogen activator (rt-PA) within 3 hours of stroke onset is now recommended for suitable candidates.126 However, <1.25% of patients admitted to hospitals with strokes in the USA received rt-PA between 1999 and 2002.128,129 This may be because of the strict eligibility requirements (Table 23-2126), lack of stroke centers or stroke experts, and a fear of ICH among front-line caregivers.
Diagnosis of ischemic stroke causing measurable neurological deficit |
Neurological signs that are not clearing spontaneously or are not minor and isolated |
Caution should be exercised in treating a patient with major deficits |
Stroke symptoms that are not suggestive of SAH |
Onset of symptoms <3 h before beginning treatment |
No head trauma or prior stroke in the previous 3 months |
No myocardial infarction in the previous 3 months |
No gastrointestinal or urinary tract hemorrhage in the previous 21 days |
No major surgery in the previous 14 days |
No arterial puncture at a noncompressible site in the previous 7 days |
No history of previous ICH |
Blood pressure not elevated (systolic <185 mm Hg and diastolic <110 mm Hg) |
No evidence of active bleeding or acute trauma (fracture) on examination |
Not taking an oral anticoagulant or, if anticoagulant being taken, international normalized ratio ≤1.5 |
If receiving heparin in previous 48 h, activated partial thromboplastin time must be in normal range |
Platelet count ≥100 000 mm3 |
Blood glucose concentration ≥50 mg/dL (2.7 mmol/L) |
No seizures with postictal residual neurological impairments |
CT does not show a multilobar infarction (hypodensity >1/3 cerebral hemisphere) |
The patient or family members understand the potential risks and benefits from treatment |
The original NINDS trial127 demonstrated unequivocal benefit for rt-PA in acute stroke: infarct volumes were smaller on CT (15 vs. 24 mL),130 inpatient stays were shorter with discharges to home occurring more frequently,131 and patients were 32% more likely to have no or minimal disability at 3 months as measured on the Barthel index.127 Efficacy of rt-PA was reflected by low numbers needed to treat (NNT), with an NNT of 8 for no or minimal residual deficit (National Institutes of Health Stroke Scale [NIHSS] score of 0-1) and an NNT of 7 for achieving independence at 3 months (modified Rankin Scale [mRS] score of 0-2).132 Subsequent criticism of baseline NIHSS score imbalance between treatment groups (more patients with NIHSS 0-5 were randomized to rt-PA, whereas more patients with NIHSS >20 were randomized to placebo) was addressed by a rigorous reanalysis that confirmed the benefit of rt-PA across all quintiles of the NIHSS.133 Phase 4 clinical studies, such as the Standard Treatment with Alteplase to Reverse Stroke study, have also helped confirm the NINDS results, with a similar proportion (43%) of patients achieving independence (mRS 0-2) at 3 months following rt-PA treatment134 as did those in the original NINDS trial. Meta-analysis of 15 postapproval publications found rates of symptomatic ICH (sICH), mortality, and excellent recovery (mRS 0-1) similar to those in the NINDS trial.135 A more recent pooled analysis of all six rt-PA trials, Alteplase ThromboLysis for Acute Noninterventional Therapy in Ischemic Stroke, European and Australian Cooperative Acute Stroke Study, and NINDS, examined 2775 patients treated within 6 hours of onset (representing 99% of all patients treated with rt-PA within randomized trials) and found clear benefit of rt-PA despite a very strict definition of good outcome (mRS 0-1), with_an odds ratio of 2.8 favoring treatment within the first 90 minutes and an odds ratio of 1.6 for the next 90 minutes after stroke onset.136
A key issue raised by the initial NINDS trial was increased sICH in patients receiving rt-PA (6.4%, vs. 0.6% in the placebo group). sICH was associated with higher NIHSS score, brain edema (acute hypodensity on CT scan) and mass effect on CT before treatment,137 with benefit of rt-PA persisting despite hemorrhage. Risk of sICH may also increase with age.138 Numerous authors have since demonstrated a similar safety profile for thrombolysis in both community and academic settings across the United States and Europe, with rates of sICH between 3.3% and 5.9%.128,134,135,136,139
IV thrombolysis is less efficacious (but likely still superior to placebo) in certain patient subgroups. Intuitively, patients with more severe strokes do worse.133 Recanalization at 24 hours is much less frequent in diabetic patients possibly because of the higher endogenous levels of plasminogen activator inhibitor in these patients.140 Patients older than 80 years benefit less than do those younger than 80 years: less independence at 3 months (mRS 0-2 achieved in 32%–36% vs. 62%–63%), higher mortality at 3 months (21%–40% vs. 5%–16%), and a questionably higher rate of sICH (2.6%–11.1% vs. 2.6%–6%).141,142,143 Nevertheless, a subgroup analysis of NINDS data failed to show any age cutoff for benefit from rt-PA.144 Patients receiving rt-PA within 3 hours with ICA-T occlusions (verified on CT angiography or MR angiography) fare worse than those with MCA occlusions,145 but better than patients not receiving any thrombolysis at all.146,147 Recent studies have discouraged subgroup analysis, pointing out that existing randomized trials of rt-PA are underpowered for such examinations.148
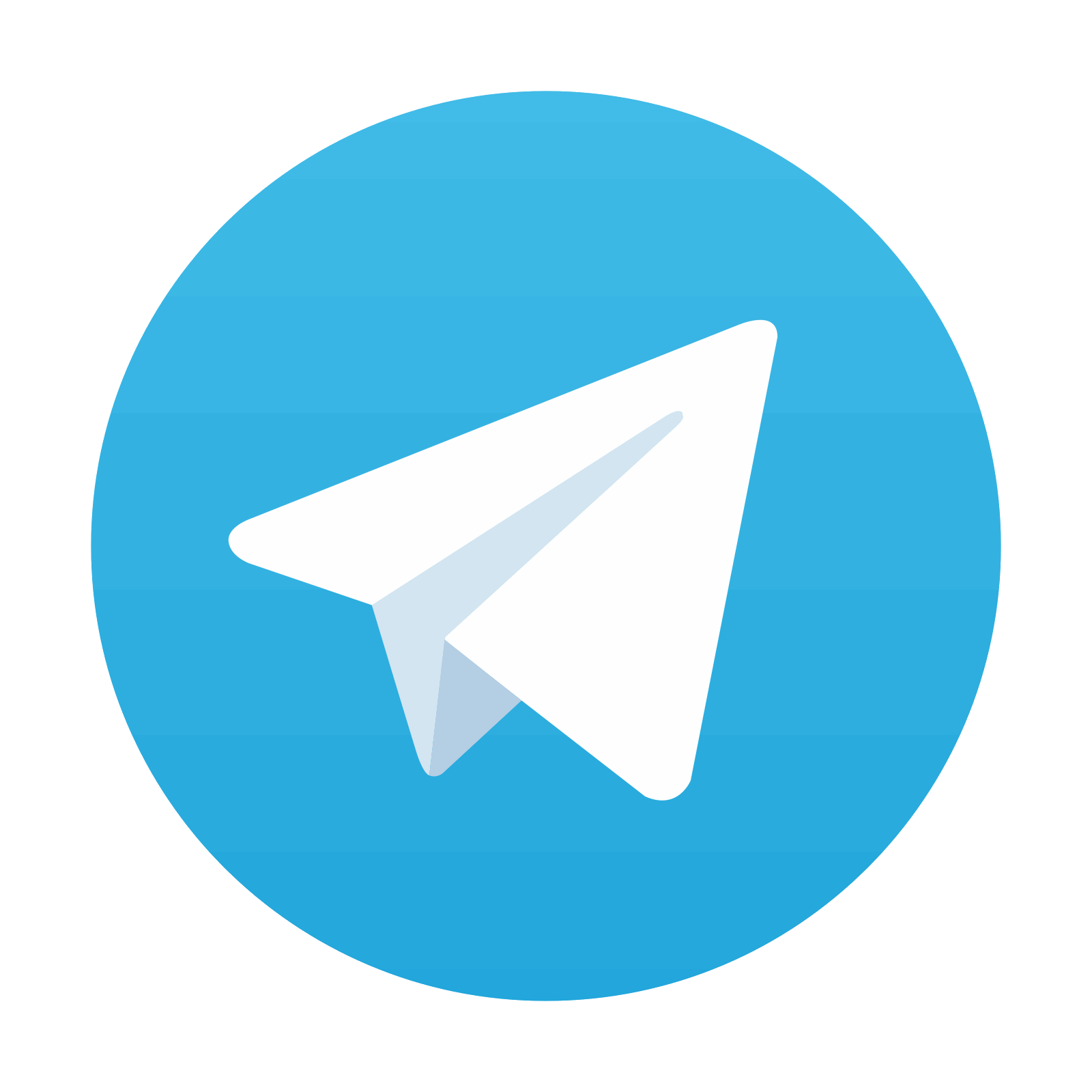
Stay updated, free articles. Join our Telegram channel

Full access? Get Clinical Tree
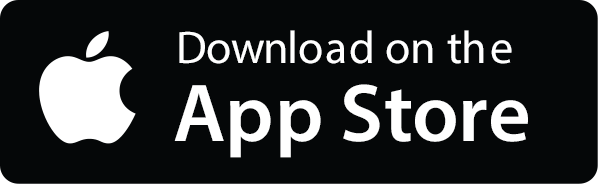
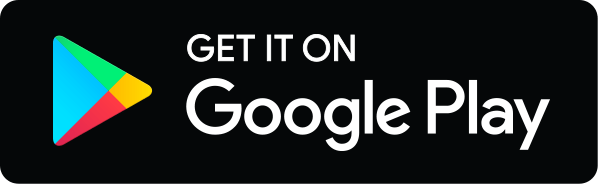