Approximately 50% of middle-aged and older adults in the United States regularly consume a diet high in saturated fat. High dietary saturated fat intake has been linked to promote atherothrombotic vascular disease. We tested the hypothesis that endothelial fibrinolytic function is diminished in middle-aged and older adults who habitually consume a diet high in saturated fat. Twenty-four healthy, sedentary middle-aged, and older adults (54 to 71 years) were studied: 10 (8 men and 2 women) with a dietary saturated fat intake <10% (lower saturated fat) of total calories and 14 (9 men and 5 women) with a dietary saturated fat intake ≥10% of total calories (high saturated fat). Net endothelial release of tissue-type plasminogen activator (t-PA), the primary activator of fibrinolysis, was determined, in vivo, in response to intrabrachial infusions of bradykinin (12.5 to 50.0 ng/100 ml tissue/min) and sodium nitroprusside (1.0 to 4.0 μg/100 ml tissue/min). Capacity of the endothelium to release t-PA in response to bradykinin was ∼30% less (p <0.05) in the high (from −0.7 ± 0.6 to 36.9 ± 3.3 ng/100 ml tissue/min) compared with the lower (from −0.3 ± 0.3 to 53.4 ± 7.8 ng/100 ml tissue/min) dietary saturated fat group. Moreover, total amount of t-PA released was significantly less (∼30%) (201 ± 22 vs 274 ± 29 ng/100 ml tissue) in the adults who reported consuming a diet high in saturated fat. These results indicate that the capacity of the endothelium to release t-PA is lower in middle-aged and older adults who habitually consume a diet high in saturated fat. In conclusion, endothelial fibrinolytic dysfunction may underlie the increased atherothrombotic disease risk with a diet high in saturated fat.
Middle-aged and older adults are at heightened risk for cardiovascular disease (CVD) because of impaired vascular endothelial function. The endothelium plays a central role in the regulation of vasomotor tone and fibrinolytic function. Endogenous fibrinolysis is the primary defense mechanism against thrombosis, and endothelial cells are the principal site of synthesis and release of tissue-type plasminogen activator (t-PA), the main activator of the fibrinolytic system. We have previously shown that endothelial release of t-PA decreases with advancing age and likely contributes to the increased risk of atherothrombotic events in middle-aged and older adults. The influence of dietary saturated fat intake on endothelial fibrinolytic function is not well understood. There are some data to suggest that dietary fat adversely influences plasma fibrinolytic concentrations ; however, it is the capacity of the endothelium to acutely release t-PA and not circulating plasma fibrinolytic concentrations that determines the efficacy of endogenous fibrinolysis. Moreover, circulating plasma fibrinolytic proteins provide an indirect, nonspecific, and potentially misleading assessment of fibrinolytic potential. The aim of the present study was to determine the influence of dietary saturated fat intake on endothelial fibrinolytic function in middle-aged and older adults. We hypothesized that the capacity of the endothelium to release t-PA would be diminished in middle-aged and older adults who habitually consume a diet high in saturated fat. To address this aim, we used an isolated forearm model to assess, in vivo, endothelial t-PA release in middle-aged and older adults with differing dietary habits.
Methods
Twenty-four healthy, sedentary middle-aged and older adults aged 54 to 71 years (17 men and 7 women) were studied ( Table 1 ): 10 (8 men and 2 women) with a dietary saturated fat intake <10% (lower saturated fat) of total calories and 14 (9 men and 5 women) with a dietary saturated fat intake ≥10% of total calories (high saturated fat). Dietary saturated fat intake classifications were based on Dietary Guidelines for Americans that recommend consuming <10% of total calories from saturated fat.
Body Mass (kg) | BMI (kg/m 2 ) | VO 2 max (mL/kg/min) | Total Calories (kcal) | Carbohydrates (%) | Protein (%) | Fat (%) | Saturated Fat (%) | |
---|---|---|---|---|---|---|---|---|
Lower saturated fat subject age (yrs) | ||||||||
54 | 67.0 | 23.5 | 31.3 | 2887 | 46 | 16 | 38 | 9 |
56 | 90.4 | 30.2 | 32.4 | 3137 | 52 | 14 | 34 | 9 |
57 | 73.1 | 23.9 | 42.0 | 2872 | 46 | 19 | 21 | 6 |
58 | 93.7 | 28.3 | 31.6 | 2331 | 45 | 15 | 30 | 6 |
58 | 99.6 | 30.4 | 35.4 | 1625 | 47 | 19 | 34 | 6 |
59 | 83.0 | 26.8 | 30.5 | 3913 | 37 | 22 | 40 | 8 |
63 | 56.6 | 20.8 | 24.9 | 1778 | 46 | 21 | 34 | 8 |
64 | 92.5 | 29.2 | 28.4 | 1653 | 45 | 15 | 30 | 6 |
67 | 82.0 | 25.9 | 24.5 | 2009 | 43 | 14 | 25 | 7 |
71 | 91.2 | 29.8 | 21.0 | 2319 | 48 | 15 | 37 | 8 |
High saturated fat subject age (yrs) | ||||||||
54 | 96.5 | 30.5 | 30.5 | 1972 | 45 | 18 | 37 | 14 |
54 | 79.1 | 28.7 | 20.1 | 2091 | 35 | 14 | 49 | 19 |
57 | 97.4 | 31.1 | 26.0 | 1961 | 35 | 11 | 54 | 13 |
58 | 90.8 | 28.7 | 28.3 | 2376 | 39 | 12 | 50 | 13 |
58 | 93.9 | 33.3 | 26.4 | 1164 | 49 | 20 | 31 | 10 |
58 | 87.7 | 31.5 | 18.1 | 2365 | 46 | 15 | 36 | 13 |
58 | 96.2 | 39.5 | 17.1 | 1542 | 54 | 15 | 32 | 12 |
59 | 84.9 | 26.8 | 35.0 | 2193 | 30 | 20 | 41 | 18 |
61 | 73.0 | 22.0 | 29.3 | 2547 | 58 | 11 | 31 | 10 |
62 | 88.0 | 29.1 | 31.4 | 1783 | 42 | 19 | 29 | 10 |
62 | 81.7 | 27.9 | 34.1 | 2648 | 28 | 18 | 50 | 14 |
65 | 93.0 | 28.7 | 24.5 | 2933 | 44 | 14 | 39 | 10 |
69 | 75.1 | 29.7 | 20.9 | 2034 | 56 | 12 | 32 | 11 |
70 | 81.0 | 27.4 | 27.4 | 2799 | 55 | 12 | 32 | 16 |
Subjects were excluded from the study if they presented a history or evidence of hepatic, renal, or hematologic disease, peripheral vascular disease, stroke, diabetes (fasting plasma glucose >125 mg/dl), dyslipoproteinemia (total cholesterol ≥240 mg/dl, triglycerides ≥300 mg/dl), and hypertension (arterial blood pressure ≥140/90 mm Hg). All subjects were screened for clinical evidence of CVD by medical history, physical examination, fasting blood chemistries, and electrocardiograms and blood pressure at rest and during incremental exercise performed to exhaustion. None of the subjects smoked or were taking medications including vitamins. All the women were at least 1 year postmenopausal and had never taken or had discontinued use of hormone replacement therapy at least 1 year before the start of the study. Before participation, all the subjects had the research study, and its potential risks and benefits explained fully before providing written informed consent according to the guidelines of the University of Colorado at Boulder.
Body mass was measured to the nearest 0.1 kg using a medical beam balance (Detecto, Webb City, MO). Body mass index was calculated as weight (kg) divided by height (m) squared. Percent body fat was determined by dual-energy X-ray absorptiometry (Lunar Corp., Madison, WI). Minimal waist circumference was measured according to the published guidelines. To assess aerobic fitness, subjects performed incremental treadmill exercise with a modified Balke protocol, as previously described. Maximal oxygen consumption ( <SPAN role=presentation tabIndex=0 id=MathJax-Element-1-Frame class=MathJax style="POSITION: relative" data-mathml='V˙O2′>V˙O2V˙O2
V ˙ O 2
max) was measured with on-line computer-assisted open-circuit spirometry.
Fasting plasma lipid, lipoprotein, glucose, and insulin concentrations were determined using standard techniques as previously described. Plasma concentrations of the inflammatory cytokine tumor necrosis factor (TNF)-α, interleukin (IL)-6, IL-18, and C-reactive protein (CRP) and oxidized low-density lipoprotein (oxLDL), a marker of oxidative stress, were determined by enzyme immunoassay (R&D Systems, Minneapolis, MN, and ALPCO Diagnostics, Windham, NH).
Subjects were instructed by a bionutritionist at the University of Colorado, Boulder Clinical and Translational Research Center (CTRC) on how to record their dietary intake for 4 days (3 consecutive weekdays and 1 weekend day) and to not alter their regular diet during the study period. All completed dietary recall records were analyzed by the CTRC bionutritionist using the Food Processor nutrition software (ESHA Research, Salem, OR).
All measurements were performed in a temperature-controlled room between 7 and 10 a.m. after a 12-hour overnight fast as previously described by our laboratory. Briefly, an intravenous catheter was placed in a deep antecubital vein of the nondominant arm. Thereafter, a 5-cm, 20-gauge catheter was introduced into the brachial artery of the same arm under local anesthesia (1% lidocaine). Forearm blood flow (FBF) was measured using strain-gauge venous occlusion plethysmography (D. E. Hokanson, Bellevue, WA) and presented as milliliters per 100 ml forearm tissue per minute. After the measurement of blood flow at rest for 5 minutes, bradykinin was infused intra-arterially at rates of 12.5, 25.0, and 50.0 ng/100 ml tissue/min and sodium nitroprusside at 1.0, 2.0, and 4.0 μg/100 ml tissue/min for 5 minutes at each dose as previously described. To avoid an order effect, the sequence of drug administration was randomized. Forearm volume was determined by the water displacement method. Net endothelial release of t-PA and plasminogen activator inhibitor-1 (PAI-1) antigen in response to bradykinin and sodium nitroprusside was calculated according to Jern et al using the following equation:
Net release = ( C V − C A ) × [ ( FBF ) × ( 101 − hematocrit/ 100 ) ]
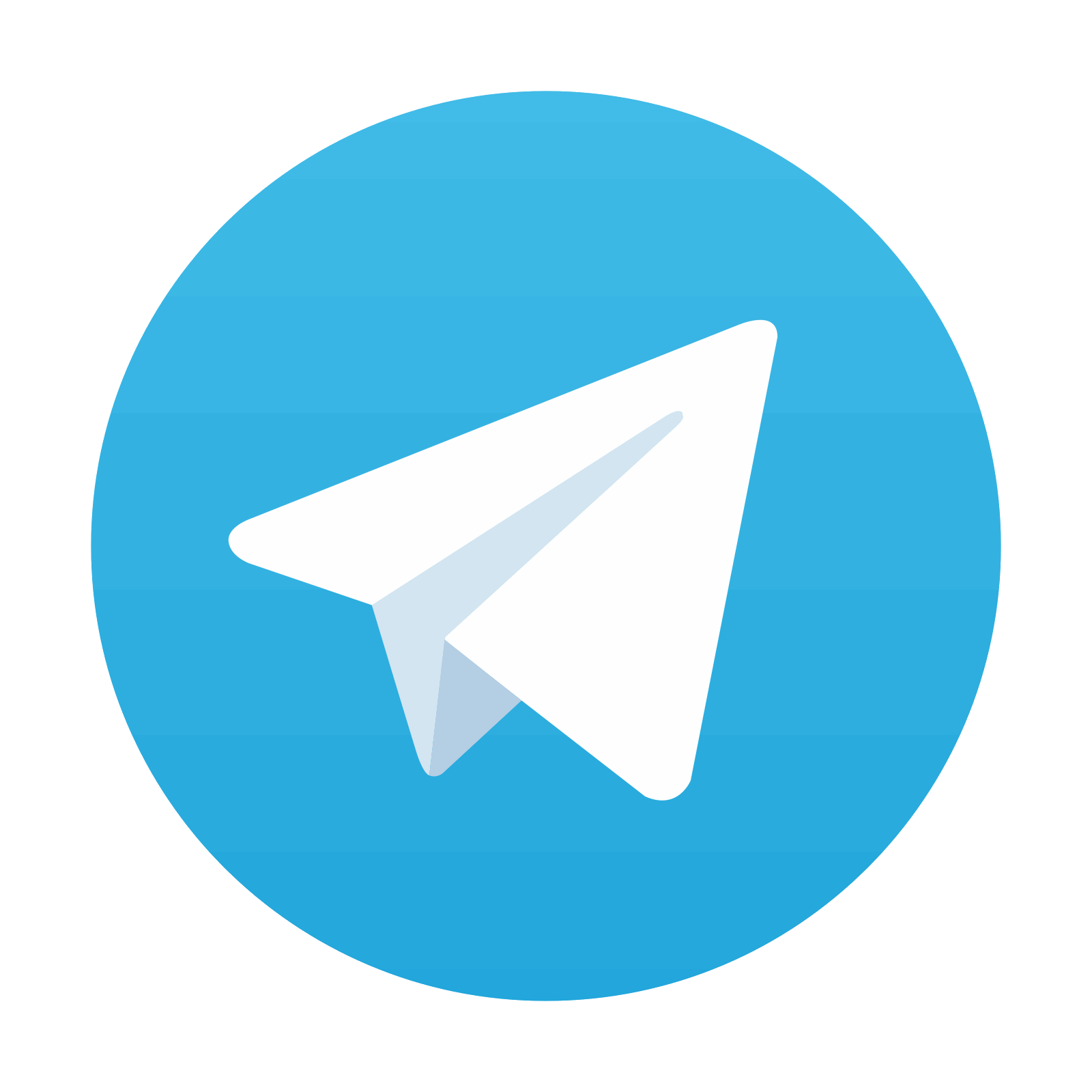
Stay updated, free articles. Join our Telegram channel

Full access? Get Clinical Tree
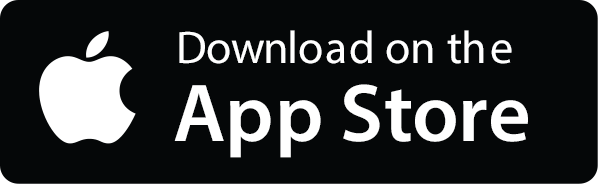
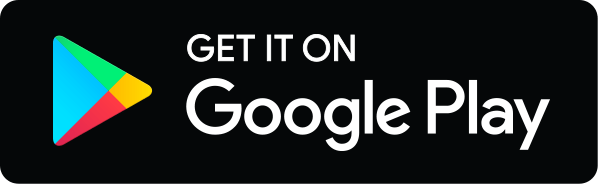