Fig. 13.1
(a) Top view of the 28 mm diameter Mobin-Uddin filter, scaled in centimeters (b) top view of the 20 mm diameter Mobin-Uddin filter (c) Side view of the 20 mm diameter Mobin-Uddin filter (Reprinted with permission from Elsevier Harlal et al. [11])
The modern era of IVC filters began in 1973 with the introduction of the transvenous Greenfield filter (Boston Scientific/Meditech, Natick, MA), a stainless steel umbrella made of six legs radiating from the apex. The Greenfield filter was the earliest device to effectively trap venous emboli while maintaining caval patency. Its funnel-shaped design forces trapped thrombi into the center while preserving circumferential blood flow which allows the Greenfield filter to minimize the risk of IVC thrombosis. Twenty years of long-term follow-up demonstrated a 4 % rate of recurrent PE and a filter patency rate of 98 % [14, 15]. The durability and safety of the Greenfield continue to be the standard benchmarks for modern IVC filters.
Early versions of the Greenfield filter used large introducer sheaths for placement and usually required a surgical venous cutdown. This part of the procedure increased the morbidity and introduced the risk of DVT at the surgical insertion site. Advances in technology then allowed for percutaneous insertion using smaller introducer sheaths. The Greenfield filter was first placed percutaneously in 1984 marking the advent of a new generation of devices with improved ease of deployment, flexibility, clot-trapping effectiveness, and, in recent years, retrievability [16].
Indications
While therapeutic anticoagulation remains the first-line treatment for DVT and PE, patients with proximal DVT who have a contraindication to or proven failure of anticoagulation require an IVC filter [17, 18]. For this group of patients, IVC filter placement provides an effective method of PE prophylaxis that is well supported by level 1 evidence and recent consensus guidelines [18]. Appropriate patient selection that strictly adheres to well-defined insertion criteria will ensure that IVC filters are used responsibly with a favorable risk-benefit ratio [19–21] (Tables 13.1, 13.2, 13.3, 13.4, and 13.5).
Table 13.1
Absolute indications for inferior vena cava filters
Contraindication to anticoagulation with documented PE, IVC, iliofemoral, femoral, or popliteal DVT |
Recurrent PE on therapeutic anticoagulation |
Hemorrhage on anticoagulation for PE/DVT |
Table 13.2
Relative indications for inferior vena cava filters
Free-floating IVC/iliac thrombus |
Septic pulmonary embolism |
Extension of DVT despite adequate anticoagulation |
High risk for complications from anticoagulation for DVT/PE: syncope, unsteady gait, poor compliance |
Massive PE with residual DVT with limited reserve for further insult (pulmonary hypertension or cor pulmonale) |
Occlusion of >50 % of the pulmonary vascular bed without reserve |
Recurrent PE with IVC filter |
DVT with severe cardiopulmonary disease and limited reserve for further insult |
Recent DVT undergoing major surgery |
Pregnancy with proximal DVT |
During DVT thrombolysis |
Table 13.3
Expanded indications for retrievable cava filters
Prophylaxis |
Need for major surgery with acute DVT (<6 months) |
Need for major surgery with significant thromboembolism risk (hypercoagulable state, history of DVT/PE, venous reconstructions) |
Documented VTE in cancer patient, burn patient, or pregnancy |
Prophylaxis for VTE in “high-risk” surgical, medical, and trauma patients |
Table 13.4
Absolute contraindications for IVC filter insertion
Thrombosis of the IVC without PE |
Complete thrombosis of access vessels (venous obstruction) |
Table 13.5
Relative contraindications for IVC filter insertion
Uncorrectable severe coagulopathy |
Bacteremia/untreated infection |
Pediatric population |
Congenital IVC abnormalities |
Upper extremity DVT |
The ease of use and retrievability of modern IVC filters have blurred the indications for treatment and lowered the threshold for IVC filter insertion. In response to the dramatic rise in the use of IVC filters, the American College of Chest Physicians (AACP) and the Society of Interventional Radiology (SIR) published indication guidelines specific to retrievable IVC filters [20, 21]. These retrievable filters can be removed when the risk of VTE is reduced and/or anticoagulation therapy is no longer contraindicated.
Debate continues on expanding the use of IVC filters to a wider population of patients outside the absolute indications described above (Tables 13.2 and 13.3). Clinicians in favor of placing IVC filters for relative and prophylactic indications cite the fatal consequences of a potentially preventable PE and the low morbidity of filter placement. Despite its intuitive appeal, the argument for lowering the threshold for IVC filter placement relies on nonrandomized data and observational studies. Ingber et al. reviewed the current clinical evidence and concluded that the only inarguable indication for IVC filter use is in patients with proximal DVT and a contraindication to anticoagulation [22]. In this specific context, a retrievable IVC filter should be placed and then removed as soon as therapeutic anticoagulation is reestablished. According to Ingebar et al., major complication rates for IVC filters equaled or exceeded the incidence of PE in patients without an IVC filter. Objective clinical evidence therefore does not support the liberal use of IVC filters for relative or prophylactic indications.
Absolute contraindications for IVC filter placement involve clinical conditions which preclude safe deployment of the device at its intended position. Examples of absolute contraindications include chronic IVC occlusion, IVC anomalies, inability to access the IVC, and IVC compression. Relative contraindications include a variety of clinical situations which increase the risk for IVC filter-associated morbidity. A large diameter IVC which approaches or exceeds the maximum diameter of the filter constitutes a relative contraindication because of the risk of device migration or dislodgement. IVC filters should also not be used in patients for whom placement of the filter is unlikely to improve their clinical course. A noteworthy example of this contraindication is the placement of a filter in the superior vena cava (SVC). The rationale for placing a filter in the SVC is to prevent PE in a patient with upper extremity DVT and a contraindication to anticoagulation. Not only does SVC filter placement have an unacceptably high periprocedural morbidity rate, patients who receive SVC filters also have limited survival due to the severity of their underlying disease [23]. Although IVC filters have been used in the SVC, no commercially available filter is specifically designed for this location [24]. Reported complications of IVC filters placed in the SVC include SVC perforation, cardiac tamponade, SVC thrombosis, and pneumothorax [25].
Evidence of Efficacy
Despite the exponential rise in IVC filter use for an expanding list of indications, data on device efficacy remain limited [4]. Even the core principle and absolute indication for IVC placement has not been critically evaluated. To date, no randomized trial or prospective observational study has been performed to evaluate IVC filters as PE prophylaxis in patients with DVT who cannot receive anticoagulation.
The best available data come from a single, large, randomized controlled European trial examining the use of anticoagulation with or without IVC filters as the initial treatment of DVT. The PREPIC (Prevention du Risque d’Embolie Pulmonaire par Interruption Cave) study has been influential in shaping the current recommendations for IVC filter use. PREPIC randomized patients with a DVT to receive permanent IVC filters and anticoagulation vs. anticoagulation alone for at least 3 months. Patients were discharged on oral anticoagulation if not contraindicated. Pulmonary angiograms performed on day 12 showed a new PE in only 1.1 % of filtered patients compared to 4.8 % of anticoagulated patients who did not have a filter (P = 0.03). This difference in PE rate did not translate into a decrease in mortality as survival curves were identical for the two groups of patients. Although 2-year data showed no difference in the incidences of symptomatic PE, major bleeding, and overall mortality between the two treatment arms, PE-related death was 10 times higher (0.6 vs. 6.3 %) in the unfiltered group. Patients who received an IVC filter had an increased rate of recurrent DVT (21 %) compared to patients in the no IVC filter group (11.6 %, p = 0.02) [26]. Of the patients with recurrent DVT in the IVC filter group, 16 patients (9 %) had symptomatic IVC thrombosis.
At 8 years follow-up, less than 50 % of patients in the PREPIC study had received anticoagulation for more than 1 year. There were lower rates of symptomatic PE in the IVC filter group compared to the no filter group (6.2 % vs. 15.1 %, P = 0.008), and a lower rate of fatal PE with a filter (P = 0.014). Despite these differences in PE incidence, mortality was the same for both groups, and a filter was not protective relatively to long-term survival according to multivariate analysis. The long-term clinical cost of an IVC filter was a higher incidence of symptomatic DVT (35.7 % vs. 27.5 %, P = 0.042). Overall IVC filter placement with anticoagulation reduced the risk of PE, but increased the risk of DVT without improving long-term survival. The authors concluded that IVC filter placement should be reserved for patients at high risk of recurrent PE [27].
Although PREPIC stands alone as the only randomized, prospective study on IVC filters, it had several limitations. The study included different types of permanent filters and randomly assigned patients to anticoagulation with unfractionated or low-molecular weight heparin. Long-term anticoagulation was not standardized in terms of drug, dosage, or duration of therapy. Statistical adjustments for multiple comparisons were not performed making the PREPIC data underpowered for subgroup analyses. Although it failed to show long-term survival benefit for IVC filters, PREPIC did show that IVC filters significantly decrease PE. These data provide the best evidence supporting the comparative efficacy of filters while providing an objective analysis of filter complications. Smaller studies have corroborated IVC filter efficacy for PE prophylaxis, as another review also reported a 1.3 % incidence of symptomatic PE after IVC filter placement [28].
Prophylactic Indications in Specific Patient Populations Without Documented DVT
Prophylactic indications for IVC filter placement apply to patients who pose a high risk for VTE and cannot receive standard DVT chemoprophylaxis or anticoagulation. Preemptively placing an IVC filter is an attempt to reduce the PE risk among patients who have no other form of protection from VTE. In theory, a prophylactically placed retrievable IVC filter could be removed when the patient’s VTE risk decreased or when the patient could safely receive chemoprophylaxis for DVT. The practice of prophylactic IVC filter placement has gained popularity for several patient populations with varying amounts of supporting evidence.
Trauma Patients
VTE is the third most common cause of death in multiple-injury trauma patients who survive for at least 24 h [29]. Risk factors for VTE in the trauma population include advanced age, severity of injury, head injury, spinal cord injury, pelvic and long-bone fractures, multiple blood transfusions, and prolonged immobility [30–32]. Shackford et al. reported a 7 % incidence of VTE in multi-trauma patients despite thromboprophylaxis compared to a VTE rate of 58 % in comparable trauma patients without prophylactic anticoagulation. Geerts et al. confirmed these high rates of VTE in a similar study [30, 33]. These studies provide solid evidence supporting the recommendation that all trauma patients receive some form of thromboprophylaxis, preferably anticoagulation [34].
Many patients with traumatic injuries cannot receive adequate thromboprophylaxis because of the risk of bleeding. Prophylactic placement of a retrievable IVC filter offers an alternative method of reducing PE risk for this subgroup of trauma patients [35–37]. Clinical studies examining the effectiveness of prophylactic IVC filters have reported mixed results. In one study, 244 IVC filter placements were analyzed in a group of 4,936 trauma patients. Approximately 93 % of retrievable filters were placed for prophylactic indications, while only 59 % were eventually retrieved. Two fractures, two migrations, and one filter tilt were documented. The PE rate was 1.6 % in patients who had IVC filters placed; however, the 0.4 % overall rate of clinically significant PE was not significantly different from the historical rate of 0.7 % (P = NS) [38]. Additional studies failed to detect a significant decrease in the incidence of clinical PE in trauma patients treated with temporary IVC filtration [39–41].
Some guidelines for the treatment of trauma patients cite levels II and III data which suggest a possible benefit of prophylactic IVC filter placement in appropriately selected patients at high risk for VTE [42–44]. Rodriguez et al. studied a cohort of critically injured patients who received IVC filters and found that PE occurred in 3 % of those with IVC filters vs. 18 % in historical controls [45]. Carlin et al. compared trauma patients receiving therapeutic IVC filters for documented PE to those receiving prophylactic IVC filters. Mortality rates were 11 % in the PE group vs. 3 % in the prophylaxis group [44]. Both of these studies compared dissimilar patient populations which undermines the strength of their conclusions. Instead of relying on these flawed studies, clinicians who treat trauma patients should use more sound clinical evidence in deciding on the use of IVC filters. As previously discussed, current evidence argues against prophylactic IVC filter placement.
The cost of the procedure and device along with the potential morbidity of IVC filter placement also contribute to the controversy regarding prophylactic filter use. Cost analysis and modeling in high-risk trauma patients demonstrated that the minimal effectiveness of prophylactic IVC filters had an estimated cost exceeding $380,000 per gained quality of life year. In terms of both in the initial hospitalization and long-term projection, prophylactically placing an IVC filter for the prevention of PE is not a cost-effective intervention [46].
Although there is no level 1 evidence showing that prophylactic IVC filters in trauma patients is superior to other VTE prevention measures, adherence to VTE prophylaxis has a wide regional variation possibly due to conflicting guidelines from different consensus panels (the Eastern Association of Trauma (EAST) vs. the American College of Chest Physicians (ACCP)) [18, 47]. Some institutions place more than 90 % of trauma filters for prophylactic indications [4], while retrieval rates rarely exceed 50 %. Over time, the cost of prophylactic IVC filter placement along with its potential morbidity should temper the excessive enthusiasm for IVC filter placement in trauma patients especially if high-quality, supporting evidence fails to materialize [4]. The authors believe that IVC filters are primarily indicated in trauma patients who fall under the absolute indication for filter use (a proven DVT/PE with a contraindication to therapeutic anticoagulation). Based on limited evidence and in agreement with Geerts et al., prophylactic IVC filter placement should be reserved for trauma patients with high predictive risk factors for VTE who cannot receive pharmacologic thromboprophylaxis for more than 4 days [48]. In the absence of convincing evidence, the use of IVC filters in trauma patients will continue to generate debate, and the ultimate clinical decision remains individualized.
Major Orthopedic Surgery
Major orthopedic surgery poses a high risk for developing VTE. Fortunately, routine perioperative thromboprophylaxis has significantly decreased the incidence of DVT/PE. Although several studies suggest that retrievable filters enhance protection against PE in selected orthopedic patients during the period of highest risk, the lack of level 1 evidence from randomized studies should continue to limit IVC filter use in this patient population [49, 50].
Cancer
Cancer increases the risk for VTE, and IVC filters have a well-defined role in the treatment of VTE in patients with malignancy [51]. In contrast, limited evidence supports the prophylactic use of IVC filters in cancer patients who do not have a documented proximal DVT or PE. Increased rates of venous thrombotic events (e.g., PE, recurrent DVT, and vena caval thrombosis) have been reported in cancer patients with IVC filters [52–54]. Although these complications are not usually fatal, they can significantly diminish comfort and quality of life. Therefore, except in carefully selected patients, prophylactic IVC filter use is generally not indicated in cancer patients. Therapeutic treatment with low-molecular weight heparin is the first-line treatment for cancer patients with DVT/PE. If recurrent DVT/PE occurs despite therapeutic anticoagulation in patients with malignancy, the intensity of anticoagulation is often adjusted instead of automatically proceeding to IVC filter placement [55]. Permanent rather than retrievable IVC filters should be used in appropriately selected cancer patients as the increased risk for PE is likely to be lifelong [56].
Major General Surgery
In patients undergoing major general surgery, the type and duration of surgery and the patient’s medical risk factors influence the risk of VTE. Numerous randomized clinical trials recommend routine pharmacologic thromboprophylaxis for patients after major surgical procedures [18, 34]. Preoperative, prophylactic anticoagulation is also safe and effective and may benefit patients at high risk for VTE [57]. The optimal duration of thromboprophylaxis following major surgery remains unclear. Shorter hospital stays raise concerns about prematurely terminating thromboprophylaxis during the postoperative period at highest risk for VTE. Although prophylactic IVC filters could extend PE protection during the postoperative period following discharge home, no solid evidence supporting IVC filter use in this setting exists. Therefore, IVC filter use in patients following major general surgery should be limited to the standard indications that apply to the general population.
Pregnancy
Pregnancy increases the risk of VTE and anticoagulation with low-molecular weight heparin is the first-line therapy for DVT during pregnancy [58]. Indications for IVC filters during pregnancy do not differ from standard guidelines and apply specifically to pregnant patients with a proximal DVT and a contraindication to anticoagulation. There is no evidence to support prophylactic IVC filter placement in pregnant patients based on high-risk status alone. Compression of the infrarenal IVC by the gravid uterus mandates suprarenal positioning of the IVC filter via transjugular access. In most cases, a permanent filter is required as the diameter of the suprarenal cava generally exceeds 28 mm which is the approved size for all retrievable filters. Results from small case series suggest that IVC filters provide safe and effective PE protection for pregnant women with VTE [59, 60].
Bariatric Surgery
Obesity is a strong risk factor for VTE, with an adjusted relative risk of 2.9 in a recent study. Hamad et al. identified BMI greater than 60, truncal obesity, and hypoventilation syndrome in bariatric surgery patients as risk factors for VTE [61, 62]. In addition, appropriate dosing of both therapeutic and prophylactic anticoagulation is difficult in obese patients due to their metabolic variability. Despite using standardized heparin protocols or two thromboprophylactic measures simultaneously, the rate of PE is still 1–3 % in bariatric surgical patients and increases to as high as 17 % in super obese patients (BMI >55 kg/m2) [63–65].
Due to the difficulties and challenges of prophylactic anticoagulation in this high-risk, morbidly obese population, IVC filter placement for PE prophylaxis has become increasingly common despite an absence of supporting level 1 evidence [66–69]. According to a 2010 study by Birkmeyer et al., IVC filters not only failed to protect bariatric surgery patients from PE, placement of an IVC filter also proved to be a major source of morbidity and mortality in this population. No subgroup of patients benefited from IVC filter placement, and more importantly, half of the complications resulting in death or disability among IVC filter patients were related to the device itself. This study, based on a Michigan statewide registry, was the first to demonstrate that IVC filter use may not be as beneficial as previous data from single centers had suggested [66]. This large prospective cohort study supports the current consensus opinion that prophylactic filters are not beneficial in the morbidly obese patients undergoing bariatric surgery.
Technical Details of Filter Placement
IVC filters are placed most often with the aid of fluoroscopy. Many patients, especially in the trauma population, have a recent CT scan of the abdomen and pelvis available for preprocedural review of renal vein landmarks and anomalies. Contrast venography performed as part of the procedure provides visualization of anatomic landmarks and accurate, real-time positioning of the IVC filter. Venous access usually involves a femoral or internal jugular approach using a vein uninvolved with clot. Previous studies suggest an increased risk of subsequent femoral vein thrombosis related to femoral vein access, particularly in patients receiving prophylactic filters [70, 71]. The use of an ultrasound guidance to evaluate for clot, rule out abnormal anatomy, and assist in venous cannulation can minimize access-related complications. A micropuncture kit is typically used to cannulate the vein. If there is any suspicion for clot between the puncture site and the infrarenal cava, a venogram should be obtained before proceeding any further to decrease the risk of embolizing clot. Once patency has been confirmed, the micropuncture catheter is exchanged over a wire for a larger sheath, and a long starter wire is advanced into the IVC under fluoroscopy (Fig. 13.2a). For a femoral approach, a catheter is positioned near the L4 level under fluoroscopy. An inferior cavagram is obtained with the patient in Valsalva to maximize visualization of the renal veins and to obtain an accurate diameter measurement of the IVC at this level (Fig. 13.2b). In some cases, an adequate venocavogram requires a power injection of contrast (20 cc/s rate; 40 cc total volume). A marker wire or catheter should be used as a reference to measure caval diameter immediately below the renal veins. Caval diameter smaller than 18 mm [72] should preclude filter placement unless the SafeFlo IVC filter designed for smaller vena cavas is used (16–19 mm). In this clinical scenario, the indications for filter placement should be compelling, if not lifesaving, as the radial force of the filter in a small cava can lead to perforation of the struts. Caval diameter >28 mm precludes the use of most standard retrievable filters and mandates the use of the permanent Bird’s Nest filter (Cook Medical, Bloomington, IN) if filter placement is essential.
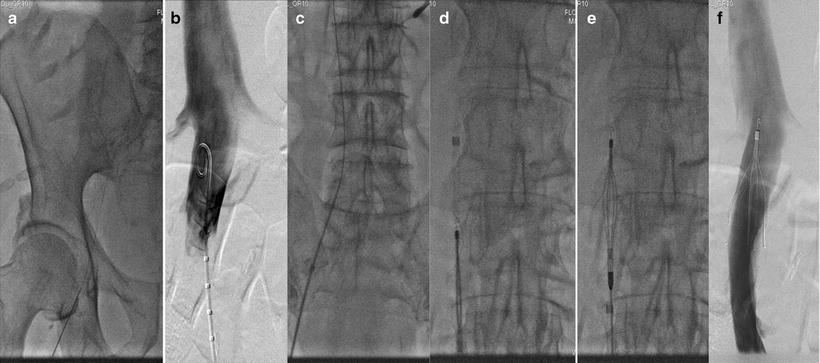
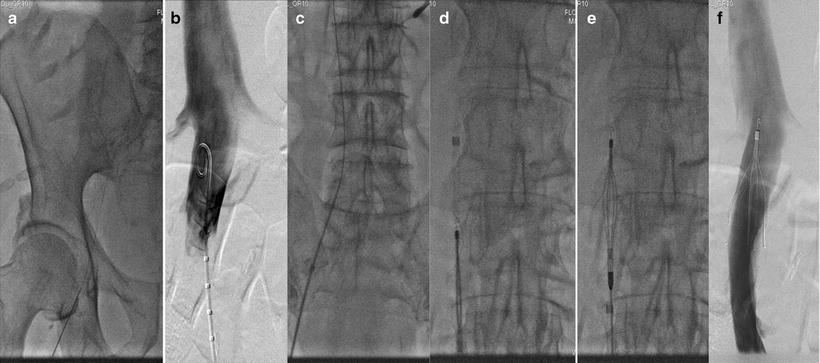
Fig. 13.2
Sequential fluoroscopic images taken during various steps of IVC filter placement. (a) A micropuncture wire is advanced though the right common femoral vein; (b) inferior venacavogram showing the renal veins; (c) the delivery sheath is advanced over the wire; (d) the filter is advanced through the delivery sheath; (e) the sheath is withdrawn to expose and release the filter; (f) completion venacavogram showing the deployed IVC filter
Renal vein anomalies, IVC transposition, IVC duplication, and IVC agenesis are anatomic variants important to identify before IVC filter placement. Empiric placement of IVC filters by bony landmarks alone should be discouraged due to the relatively high prevalence of venous anomalies. Contrast venography performed during filter placement provides a roadmap of venous anatomy and helps identify the renal veins to guide placement of the device. The IVC filter is inserted into the sheath and positioned with the superior tip of its cone between the renal veins. Under direct fluoroscopic guidance, the sheath is slowly withdrawn to release and deploy the IVC filter (Fig. 13.2c, d). A completion venogram is performed to ensure proper placement, confirm IVC patency, and evaluate for significant filter tilt (Fig. 13.2f). The clinical scenarios which warrant suprarenal filter placement are listed in Table 13.6. As previously discussed, the larger diameter of the suprarenal cava usually precludes the use of standard retrievable IVC filters.
Table 13.6
Indications for suprarenal IVC filter placement
• Thrombus in IVC |
• Malpositioned infrarenal filter |
• Duplicate IVC |
• Gonadal vein thrombosis |
• Pregnancy |
Troubleshooting
Occasionally, the nonselective venocavogram fails to demonstrate the location of the renal veins. In these cases, selective cannulation of the renal veins with a curved catheter (Cobra C-2, Terumo Medical Corporation, Somerset, NJ) can determine the level of each renal vein and guide filter placement. If venous anomalies are suspected, selective venography provides more detailed anatomic information which changes the intended location of IVC filter placement in a significant number of patients compared to nonselective venography [73]. In the authors’ opinion, the increased cost, time, contrast, and radiation loads to the patient do not justify selective renal vein cannulation in routine cases.
Filter tilt is common with the traditional triangular, cone-shaped filters especially when deployed from femoral vein access. Internal jugular vein access involves less torque on the filter resulting in a lower tendency to tilt. Retrievable filters placed through the internal jugular vein can also be easily retrieved and redeployed if filter tilt is severe. If a filter is tilted after deployment through the femoral vein, it can be gently manipulated with a J-wire or pigtail catheter to reposition the filter. Attempted manipulation of the filter should be done with caution to avoid dragging the filter inferiorly or causing the cone to tilt into one of the renal veins. If this does occur, the interventionalist should establish access through the internal jugular vein in order to retrieve and reposition the filter.
Rarely, the filter fails to completely deploy because the struts stick together and do not expand to fill the lumen of the IVC (Fig. 13.3). This technical problem can be corrected with gentle manipulation using a pigtail catheter, being careful not to embolize the filter as it is not yet anchored to the caval wall [74]. IVC diameter varies depending on the patient’s volume status. All currently available filters can be safely deployed in cavas up to 30 mm in diameter, depending on the specifics of each manufacturer’s indications for use. An excessively large diameter IVC or so-called megacava may require a Bird’s Nest filter which has been approved for the use in caval diameters of up to 40 mm. Alternatively, standard diameter filters can be placed in both the common iliac vein to provide PE protection [75].
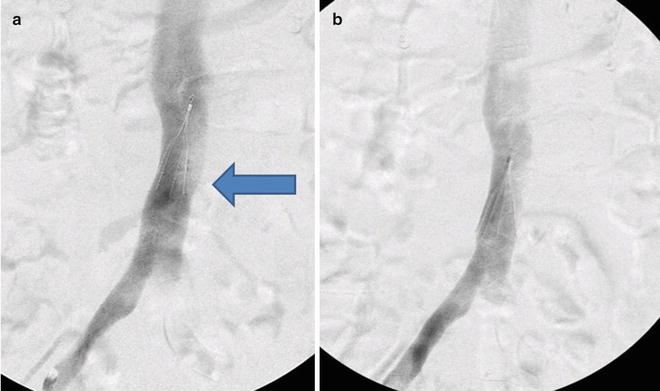
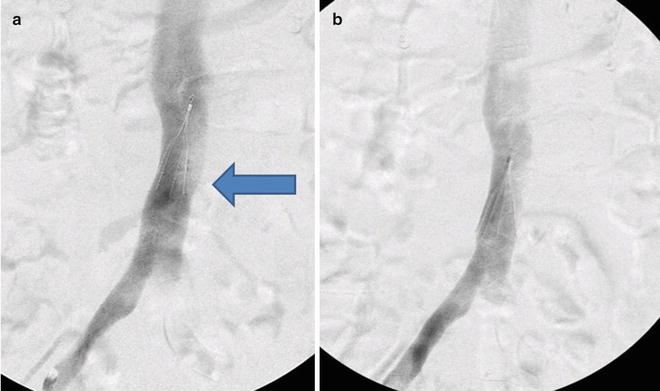
Fig. 13.3
(a) Filter is deployed and fails to fully expand (arrow). (b) After gentle manipulation with a pigtail catheter, the struts expand
Bedside Filter Placement Using Transabdominal or Intravascular Ultrasound
In patients who are too unstable to transfer to the operating room or interventional suite, ultrasound-guided IVC filter placement is safe and cost-effective [76]. Although large body habitus, recent abdominal surgery, and excessive bowel gas make adequate sonographic visualization less predictable, a single-center study reported a 97.7 % technical success rate for IVC filter placement with transabdominal ultrasound. If transabdominal ultrasound was not adequate, intravascular ultrasound was used to place the filter with a technical success rate of 96.2 % [77–79].
Filter Selection
No randomized controlled trials between different IVC filter models have been performed, and comparative observational studies failed to show superiority of one filter over another. The interventionalist placing the filter should consider the features unique to each type of filter as well as the specific clinical scenario in deciding which type of filter to use. IVC filters are designed in the following categories:
Permanent filters: Provides permanent, lifelong caval filtration. The design of these filters maximizes secure fixation to the IVC wall.
Convertible filters: Provides permanent, lifelong caval filtration if needed. The filter structure maximizes secure fixation. The filtration portion of the device can be removed if no longer necessary, leaving the circumferential structure of the filter in place as an open IVC stent. These filters are currently in clinical trials.
Retrievable filters: Provides caval filtration with the option of retrieval if the filter is no longer needed. Filters anchor to the caval wall with hooks and radial force, but can be retrieved within a specific time interval; filters not retrieved within this interval can function as permanent filters.
FDA-Approved Permanent and Retrievable Filters
The stainless steel conical Greenfield filter was introduced in 1973 and has been extensively evaluated. The Greenfield filter spans a diameter of 30 mm and traps 3 mm or larger emboli [2, 14, 15, 80]. Initially, there was a high rate of venous access site thrombosis (up to 41 %), due to the 24 Fr delivery system [81]. In 1989, Greenfield filters were constructed out of titanium alloy (nonthrombogenic, nonferromagnetic, resistant to fatigue and corrosion) and delivered via a 12 Fr sheath. Subsequent modifications have minimized technical complications including tilt (<5 %), migration (1 %), recurrent PE (2 %), and access site thrombosis (4 %). Caval patency after Greenfield filter placement is approximately 98 % [15].
The Bird’s Nest filter was introduced in 1982. This permanent filter is delivered by a 12 Fr system. At deployment, two struts are released to attach to the caval wall, and four wires extend in a random “Bird’s Nest” configuration. The unique feature of the Bird’s Nest filter is the struts which span a diameter of up to 60 mm allowing the filter to accommodate large caliber cavas. Unlike other filters, the Bird’s Nest filters rarely perforate the IVC because the devices do not have radially oriented struts [82]. The tighter configuration of the Bird’s Nest filter traps smaller emboli resulting in a low recurrent PE rate of 1–7 %. The downside of the Bird’s Nest geometry is a higher rate vena caval thrombosis (3–19 %) [2, 83, 84].
The Vena Tech LGM (B Braun, Bethlehem, PA) permanent IVC filter is made from biocompatible and nonferromagnetic Phynox (cobalt, chromium, iron, nickel, molybdenum). It maintains a conical shape with a central apex and six radiating struts containing flat side rails with hooks. The Vena Tech LGM accommodates caval diameters as large as 28 mm and is deployed through a 10 Fr delivery from either a jugular or femoral approach. The Vena Tech Low-Profile (LP) (B Braun, Bethlehem, PA) IVC filter is similar to the LGM but uses a 7 Fr delivery system. An experience with both Vena Tech LGM and LP IVC filters has exposed technical problems and complications including incomplete opening of the filter, recurrent PE rates as high as 6 %, and access site thrombosis rates as high as 23 % [85]. Caval patency rates for Vena Tech LGM filters are 92 % at 2 years, but only 67 % at 9 years [86].
The Simon nitinol (Bard Peripheral Vascular Inc., Tempe, AZ) permanent IVC filter was approved in 1990 and is made from nonferromagnetic nitinol. The bi-level conical configuration includes a superior cone with eight overlapping loops in radial array from the apex, while the inferior cone has six limbs in radial array from its apex, each with a hook to engage the caval wall. The bi-level configuration allows entrapment of large emboli in the inferior limbs and of small emboli in the superior dome [87, 88]. It can accommodate caval diameters as large as 28 mm. Long-term studies report recurrent PE rate of 4 % and vena caval thrombosis of 4 %. The 7 Fr delivery system is appropriate for femoral, jugular, and antecubital approaches with a reported 4 % rate of access site thrombosis [89].
The TrapEase (Cordis, Miami, FL) permanent IVC filter was introduced in 2000. Its unique symmetric trapezoidal double-basket designs connects a cephalic conical basket to its mirror image pointing caudally through six side struts, each with hooks providing filter attachment to the caval wall. A 6 Fr delivery system deploys this 50 mm long and 35 mm wide IVC filter through antecubital, jugular, and femoral approaches. The OptEase (Cordis, Miami, FL) IVC filter is similar to the TrapEase design with the addition of a hook placed on the caudal apex and unidirectional anchoring barbs to allow retrievability. Based on initial safety and efficacy data on retrievals, the FDA approved OptEase IVC filter retrieval up to 14 days after placement [90].
TrapEase and OptEase have high technical success rates with limited filter migration, insertion site thrombosis, and filter fracture rates. In a large single-center experience, symptoms of PE developed in 8 % of patients with TrapEase filters, but only 0.1 % developed into fatal PEs. The double-basket filter design has generated concern that TrapEase and OptEase filters have a higher risk of vena cava occlusion. In animal models, trapped emboli in the TrapEase filter slow blood flow between the inferior basket of the filter and the caval wall. This stagnant blood flow promotes further thrombosis. Schutzer et al. reported symptomatic vena caval thrombosis of 1.5 %, and Corriere et al. reported TrapEase filters had an increased risk for vena caval thrombosis compared to other filter designs [91, 92]. In a large study, venography before retrieval confirmed intrafilter thrombus in 22 % of TrapEase filters; however, the study failed to make a clear distinction between captured emboli and thrombus formation [93].
The Recovery G-2 (Bard Peripheral Vascular Inc., Tempe, AZ) permanent IVC filter was modified from the original Recovery nitinol IVC filter. Approved in 2006, the 41 mm length filter is conical in design with six radiating stabilizing arms and six separate radiating fixation legs with hooks for anchoring. In 2008, the Recovery G-2 IVC filter and G-2 Express filter incorporated a hook at the apex of the conical filter to allow for retrievability. These filters are delivered through a 7 Fr low-profile system and can accommodate caval diameters up to 28 mm. Early reports cite PE rates of 2.8 % and no cases of caval thrombosis [94]. The EVEREST trial recorded a 95 % successful retrieval rate of Recovery G-2 filters with a mean indwelling time of 140 days and a maximum of 300 days [95]. In 2010, the FDA approved the Eclipse, an electropolished finish version of the G-2 Recovery IVC filter (Bard Peripheral Vascular Inc., Tempe, AZ). The electropolish creates an ultrasmooth finish to prevent micro-imperfections.
The nonferromagnetic Gunther Tulip (Cook Medical, Bloomington, IN) IVC filter was approved in 2000 for permanent use and in 2003 for retrieval. The conichrome filter is conical with four filters legs with barbed anchoring hooks radiating from the apex and four additional wires wrapped around these legs. The Gunther Tulip filter is 50 mm long and 30 mm wide and delivered with an 8.5 Fr system from the femoral approach and a 7 Fr system from the jugular approach. A hook at the apex allows removal even after long dwell times of greater than 180 days [96]. Rates of recurrent PE rates are 1–2 %, filter tilt 4–11 %, IVC penetration 2–4 %, and caval thrombosis 5–7 % [97–101].
An updated model of the Tulip IVC filter is the Celect (Cook Medical, Bloomington, IN), approved in 2007 for permanent use and in 2008 for retrievable use. Changes to the tulip structure included eight secondary legs to improve stabilization and centering and a wider base for use in caval diameters up to 30 mm. Although the Celect IVC filter provides PE protection and retrievability (symptomatic PE rate of 2.8 %; successful retrieval 89 % at 52 weeks), it also demonstrated a high incidence of caval penetration by a filter leg [102, 103].
Recently, approved IVC filters include ALN (ALN Implants Chirurgicaux Ghisonaccia, France), SafeFlo (Rafael Medical Technologies, Caesarea, Israel), Crux (Crux Biomedical, Menlo Park, CA), and Option (Rex Medical/Argon Medical Devices, Athens, TX). The ALN filter is designed from nonferromagnetic stainless steel in a conical shape with three additional long curved legs radiating from the apex to facilitate self-centering. After a median follow-up of 21 months in a small group of patients receiving the ALN filter, no recurrent PE or vena caval thrombosis rates were reported [104]. Successful ALN filter retrieval rates dramatically decrease after 3 months; only 50 % of IVC filters were retrieved with failures due to adherence to the wall and filter tilt [105]. SafeFlo IVC filter has a non-tilt spiral shape oversized to a double-ring platform. The available filter sizes correlate with the caval diameter: small, 16–19 mm; medium, 19–22 mm; and large, 22–25 mm. Data on the long-term safety and efficacy of the SafeFlo filter remains limited. The novel design of the Crux filter includes two nitinol spiral parts crimped at the ends to form a double-looped helical structure. A filter mesh is attached to one loop, while three fixation anchors are attached to the opposite loop. Delivered through a 6 Fr system, two sizes are available: one for small cavas (17–22 mm) and one for large cavas (22–28 mm). The symmetric design of the Crux filter allows retrieval using a jugular or femoral approach [106]. The Option IVC filter is laser cut from one single piece of nitinol. Its conical shape has six struts radiating from the apex and includes a caudal hook for retrieval. Although it only requires a 5 Fr delivery system, the Option filter can be used in a maximal caval diameter of 32 mm. In a multicenter, single-arm clinical trial, 8 % of patients had recurrent PE, 2 % of patients had filter migration, and 3 % had caval thrombosis [106].
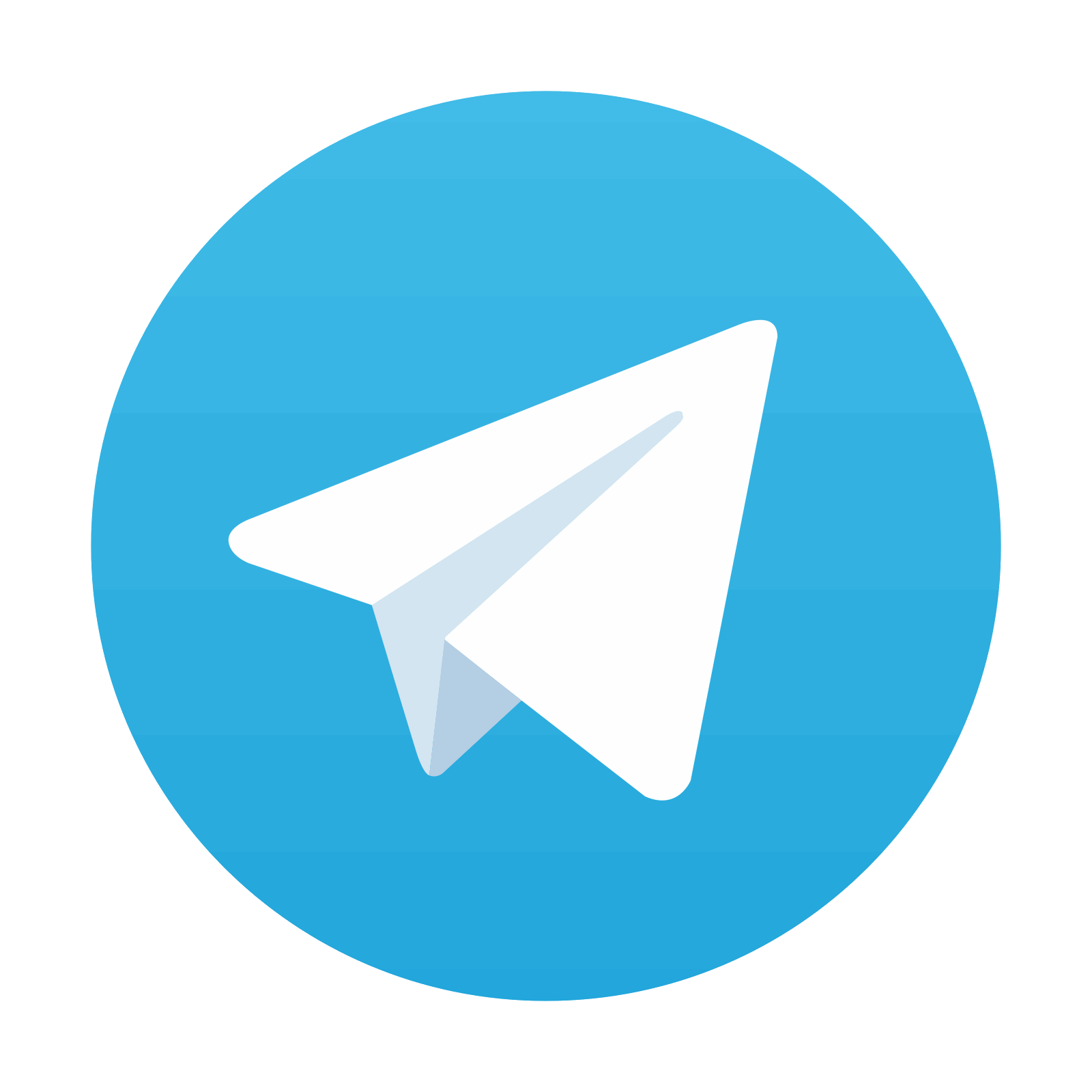
Stay updated, free articles. Join our Telegram channel

Full access? Get Clinical Tree
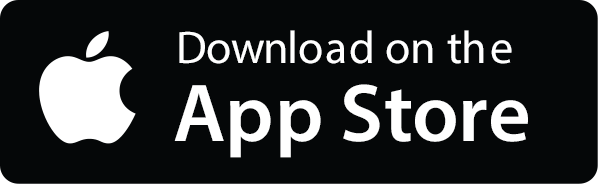
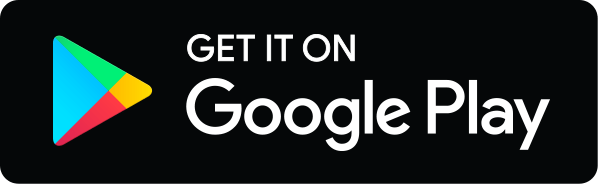