How the left ventricle remodels in response to a high-volume stimulus is important in evaluating the endurance athlete’s heart. Marathoners and patients with isolated, moderate chronic compensated mitral regurgitation (MR) represent physiologic and pathologic forms of eccentric left ventricular (LV) remodeling in response to intermittent and chronic volume overload, respectively. Thus, in this study, magnetic resonance imaging with tissue tagging and 3-dimensional data analysis at rest were performed in 19 marathoners (mean age 39 ± 10 years, 47% women), 17 patients with isolated MR without coronary artery disease or medical therapy (mean age 46 ± 5 years, 53% women), and 24 controls (mean age 45 ± 8 years, 50% women). Marathoners and patients with MR had approximately 35% greater LV end-diastolic volume indexes, approximately 50% greater end-systolic volume indexes, and approximately 34% greater LV stroke volume indexes (p <0.0001) compared to controls. However, marathoners’ hearts had increased long-axis length, while those of patients with MR did not differ from the hearts of controls. The hearts of patients with MR had greater LV global and apex sphericity compared to those of marathoners and controls (p <0.0001). Marathoners had normal LV mass/volume ratios and wall thicknesses, whereas these were significantly decreased in the MR group. In marathoners, the baseline LV work rate was similar to that in controls and higher in patients with MR compared to controls. In conclusion, marathoners’ hearts achieve elevated stroke volume at rest with adherence to an elliptical shape defined by 3-dimensional geometry and mass/volume ratio. Thus, a comprehensive evaluation of LV geometry and mass/volume ratio may be important in the evaluation of the athlete’s heart.
Marathoners experience cardiac remodeling that is characterized by commensurate increases in left ventricular (LV) volume and mass that result in increased stroke volume (SV). This eccentric cardiac hypertrophy is a putative adaptation to endurance training that is generally thought to enhance physiologic reserve capacity. However, there is controversy regarding the potential pathologic consequences of cardiac enlargement. Chronic compensated mitral regurgitation (MR) represents another form of cardiac enlargement. Although the 2 conditions are associated with an increase in LV SV, MR is facilitated by regurgitation through a secondary ejection pathway into the left atrium that preserves LV shortening and ejection fraction. Importantly, hearts with MR do not achieve similar large increases in LV volumes and SV due to functional MR that is usually a result of primary myocardial disease, but rather due to primary degenerative mitral valve disease with a normal LV ejection fraction. Although not nearly the same facilitation of ejection, a marathoner’s heart ejects into a relatively compliant vascular bed. A previous study compared these 2 conditions in terms of their biochemical and molecular signaling mechanisms. However, how changes in LV geometry and mechanics in marathoners differ from a pathologic form of volume overload that still maintains LV systolic shortening in a normal range remains to be elucidated. The question of the “appropriateness or suitability” of cardiac enlargement in the athlete’s heart is frequently raised. One major factor in defining a physiologic response to chronic hemodynamic stress is the adherence to an elliptical LV shape, as opposed to an increase in LV sphericity, and the maintenance of a normal LV mass/volume ratio. Deviations from a normal match of geometry and muscle mass can cause increases in wall stress and myocardial oxygen demand, resulting in decreased LV function or sudden death. Therefore, in the present study, we compared marathon runners’ hearts to those of patients with isolated MR with similar LV volumes and SVs using magnetic resonance imaging (MRI) with tissue tagging and 3-dimensional (3D) analysis.
Methods
Nineteen marathoners, 17 patients with degenerative isolated MR, and 24 controls constituted the study population. The control subjects and the marathoners had no histories of cardiovascular disease and were not using any prescription medications. Control subjects were not engaged in any aerobic training, with only varying degrees of recreational activities. Marathoner designation was based on having run 4 full marathons over the previous 2 years and running an average of 50 miles per week. Chronic isolated MR was defined as at least moderate severity, with an LV EF >60% on Doppler echocardiographic examination in the absence of symptoms or obstructive coronary artery diseases determined by exercise testing with nuclear perfusion. No patient with MR had a history of hypertension or was taking any medication at the time of study. The study protocol was approved by the University of Alabama at Birmingham institutional review board, and all participants gave written informed content.
All participants underwent MRI on a 1.5-T scanner (Signa; GE Healthcare, Milwaukee, Wisconsin) optimized for cardiac imaging. An electrocardiographically gated, breath-hold, segmented k-space steady-state free precession technique was used to obtain cine images with standard (2-, 3-, and 4-chamber and short-axis) views using the following typical parameters: field of view 40 × 40 cm, image matrix 256 × 128, flip angle 45°, repetition time 4 ms, echo time 1.8 ms, 20 cardiac phases, slice thickness 8 mm, and no slice gap. Tagged MRI was acquired on the same scanner using the following typical parameters: repetition time 8 ms, echo time 4.2 ms, and tag spacing 7 mm. Tag lines were tracked and edited, if necessary, by expert users. Endocardial and epicardial contours were manually traced on cine MRI acquired near end-diastole and end-systole by blinded assignment. Volumes were calculated from summated serial short-axis volumes and indexed to body surface area. LV 3D geometric parameters were measured on the basis of the contours using software developed in-house. LV 2-dimensional apical curvatures were computed from endocardial contours drawn on a 4-chamber view using a standard formula. The sphericity index was defined as the ratio of LV long-axis length to inner diameter. LV end-systolic (LVES) maximum shortening was computed at all wall segments (excluding the apex) using software developed in-house and averaged. LVES 3D twist was computed as described by Russel et al. LV 2-dimensional twist was calculated in each time frame using improved harmonic phase tracking, and a 2-dimensional twist-time curve was constructed and differentiated with respect to time to compute LV peak early diastolic untwist velocity (degrees per beat), which was normalized to heart rate (HR).
LV work rate (mm Hg × L/min) was defined as LV SV × LV-P es × HR/1,000, where LV-P es is LVES pressure, which can be approximated by the mean LV systolic blood pressure, P mean , defined as LV-P es ≈ P mean = (2/3 × systolic blood pressure) + (1/3 × diastolic blood pressure). Systolic and diastolic blood pressures were measured by sphygmomanometry, with patients in the supine position immediately before and after MRI. The reported systolic and diastolic blood pressures were the average of pre-MRI and post-MRI values.
One-way analysis of variance and generalized Wald tests were used to compare groups for continuous and categorical variables, respectively. Homogeneity of variance was tested using Levene’s test. Appropriate data transformation was conducted if the homogeneity assumption was violated. The Tukey-Kramer procedure was performed to control the pairwise comparisons among the groups. Data are presented as mean ± SD. A p value <0.05 was considered statistically significant. All statistical analysis was performed using SAS version 9.2 (SAS Institute Inc., Cary, North Carolina).
Results
Controls, marathoners, and patients with MR were matched for age, gender, and body surface area ( Table 1 ). Marathoners had lower rest HRs than controls and patients with MR. Diastolic and systolic blood pressures did not differ among the 3 groups. LV work rate did not differ in the marathoners compared to the controls, while it was significantly higher in patients with MR. Marathoners and patients with MR had greater LV end-diastolic volume, LVES volume, and LV SV indexes compared to controls ( Table 2 ). The LV ejection fraction did not differ among the groups. Marathoners had significantly greater LV mass indexes compared to controls, but LV mass index did not differ between patients with MR and controls. LV mass/volume ratio was significantly lower in patients with MR compared to controls and marathoners. Marathoners had commensurate increases in right ventricular (RV) end-diastolic volume, RV end-systolic volume, and RV SV indexes compared to controls, while RV volumes in patients with MR did not differ from those in controls. RV ejection fractions were similar in all groups.
Variable | Controls (n = 24) | Marathoners (n = 19) | Patients With MR (n = 17) |
---|---|---|---|
Age (yrs) | 45 ± 8 | 39 ± 10 | 46 ± 5 |
Women | 50% | 47% | 53% |
Body surface area (m 2 ) | 1.91 ± 0.17 | 1.78 ± 0.23 | 1.90 ± 0.20 |
HR (beats/min) | 67 ± 11 | 55 ± 9 ∗ | 64 ± 11 † |
Diastolic blood pressure (mm Hg) | 77 ± 9 | 73 ± 10 | 75 ± 9 |
Systolic blood pressure (mm Hg) | 123 ± 13 | 114 ± 15 | 118 ± 15 |
LV work rate (mm Hg × L/min) | 613 ± 174 | 544 ± 177 | 789 ± 215 ∗ † |
Variable | Controls (n = 24) | Marathoners (n = 19) | Patients With MR (n = 17) |
---|---|---|---|
LV end-diastolic volume index (ml/m 2 ) | 69 ± 10 | 92 ± 15 ∗ | 98 ± 18 ∗ |
LVES volume index (ml/m 2 ) | 25 ± 7 | 37 ± 8 ∗ | 36 ± 8 ∗ |
LV SV index (ml/m 2 ) | 44 ± 7 | 55 ± 7 ∗ | 62 ± 14 ∗ |
LV ejection fraction (%) | 65 ± 7 | 60 ± 4 | 63 ± 6 |
LV mass index (g/m 2 ) | 52 ± 12 | 62 ± 9 ∗ | 58 ± 10 † |
LV mass/volume ratio (g/ml) | 0.76 ± 0.18 | 0.76 ± 0.12 | 0.60 ± 0.07 ∗ † |
RV end-diastolic volume index (ml/m 2 ) | 72 ± 11 | 104 ± 13 ∗ | 78 ± 15 † |
RV end-systolic volume index (ml/m 2 ) | 34 ± 8 | 47 ± 8 ∗ | 35 ± 8 † |
RV SV index (ml/m 2 ) | 39 ± 8 | 58 ± 8 ∗ | 43 ± 10 † |
RV ejection fraction (%) | 54 ± 8 | 55 ± 5 | 55 ± 7 |
As listed in Table 3 , LV dimensions were significantly increased in patients with MR and marathoners compared to controls, while LVES inner diameter did not differ between marathoners and controls. However, marathoners had significantly longer LV lengths than patients with MR. Therefore, marathoners had normal sphericity indexes, whereas the hearts of patients with MR were more spherical. MR patients’ hearts also had significantly lower apical curvatures compared to those of marathoners and controls, as demonstrated in Figures 1 and 2 .
Variable | Controls (n = 24) | Marathoners (n = 19) | Patients With MR (n = 17) |
---|---|---|---|
LVED diameter (mm) | 51 ± 4 | 54 ± 4 ∗ | 59 ± 4 ∗ † |
LVES diameter (mm) | 36 ± 4 | 38 ± 4 | 39 ± 3 ∗ |
LVED length (mm) | 90 ± 8 | 98 ± 10 ∗ | 89 ± 10 † |
LVES length (mm) | 71 ± 8 | 76 ± 9 | 67 ± 10 † |
LVED sphericity index | 1.77 ± 0.19 | 1.80 ± 0.18 | 1.53 ± 0.16 ∗ † |
LVES sphericity index | 1.96 ± 0.29 | 1.96 ± 0.17 | 1.71 ± 0.20 ∗ † |
LVED apical curvature (1/cm) | 1.40 ± 0.29 | 1.36 ± 0.26 | 1.00 ± 0.31 ∗ † |
LVES apical curvature (1/cm) | 2.84 ± 1.21 | 2.20 ± 0.53 | 1.70 ± 0.43 ∗ † |
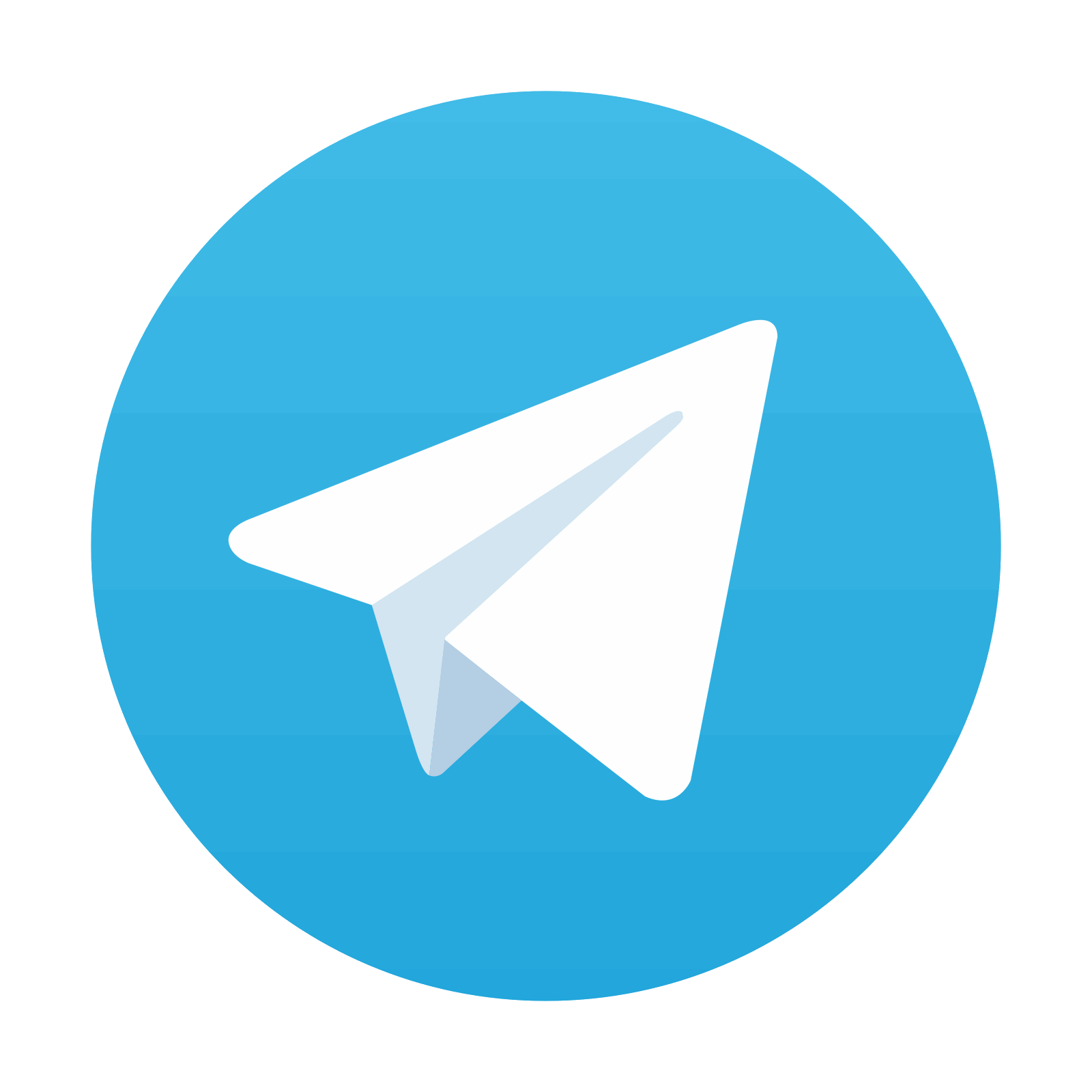
Stay updated, free articles. Join our Telegram channel

Full access? Get Clinical Tree
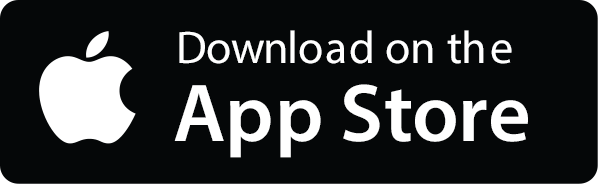
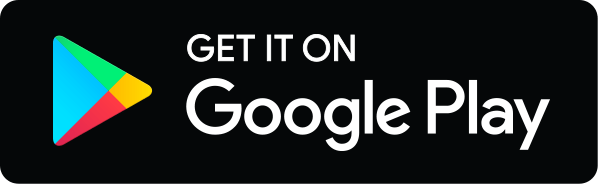
