Left bundle branch block (LBBB) causes an abnormal pattern of cardiac activation and affects regional myocardial function. Although recognition of LBBB on the surface electrocardiogram is straightforward, dissecting its effect on patient treatment and outcome can be more challenging. The altered pattern of cardiac activation in LBBB causes electrical and mechanical ventricular dyssynchrony, influences ischemia detection on the surface electrocardiogram, and affects stress testing and imaging modalities dependent on wall motion and thickening. Restoration of synchrony by biventricular pacing can improve symptoms and longevity in carefully selected patients. The diagnostic, prognostic, and therapeutic implications of LBBB across this spectrum are discussed in this review.
The presence of left bundle branch block (LBBB) on a 12-lead electrocardiogram, whether new or established, poses multiple important and perplexing questions to the healthcare provider. Realistically, LBBB should be considered a “cardiac clinical entity,” rather than just an electrocardiographic finding. Its presence has far reaching consequences in acute clinical care, such as in the setting of acute myocardial infarction (AMI), and in chronic conditions, such as heart failure (HF), where it can be helpful in guiding cardiac resynchronization therapy (CRT), and stable coronary artery disease. LBBB provides prognostic information, but it also poses challenges in performance and interpretation of diagnostic tests. The present review summarizes the current knowledge of the diagnostic, prognostic, and therapeutic implications of LBBB.
Anatomy and Electrocardiographic Recognition
Anatomically, the left bundle arises from the His bundle on the left endocardial side of the muscular septum. On rare occasions, when the His bundle lies on the right side of the interventricular septum, it might actually traverse through the septum. The proximal portion of the left bundle might vary in width, from 1 to 14 mm, a variability that is also influenced by the location of the His bundle in relation to the septum. The left bundle then broadens as it travels apically and divides into subdivisions; it hardly ever has 2 distinct anterior and posterior subdivisions. More commonly, it is composed of multiple interconnected subdivisions, which vary in number, size, and morphology. However the simplistic division into anterior and posterior fascicles does have some usefulness for clinical care. The anterior subdivisions are usually supplied by the septal perforators from the left anterior descending artery, and the posterior subdivisions receive vascular supply from the left anterior descending artery and posterior septal perforator branches of the posterior descending artery, making it more resistant to ischemia and necrosis.
The accepted electrocardiographic criteria for diagnosing LBBB include a QRS duration ≥120 ms; a broad-notched or slurred R wave in leads I, aVL, V 5 , and V 6 (occasionally, an RS pattern in V 5 and V 6 ); absent Q waves in leads I, V 5 , and V 6 ; and an R peak time >60 ms in leads V 5 and V 6 but normal in leads V 1 to V 3 ( Figure 1 ). A small, but significant, proportion of patients with left ventricular (LV) hypertrophy and left anterior fascicular block can meet these criteria without actually having LBBB. In patients with true LBBB, the QRS duration will be ≥130 ms and will show characteristic notching, which represents septal and lateral wall breakthrough of the activation front. Incorporation of these criteria might be particularly relevant in patients with HF being considered for CRT.
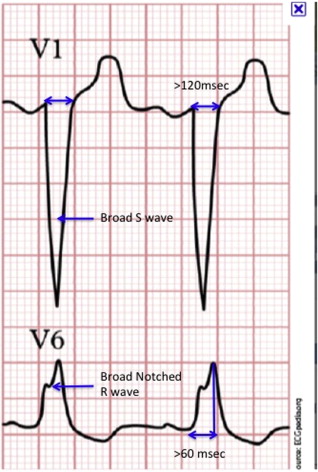
LBBB can be transient or dependent on alterations in heart rate (“rate-dependent LBBB”). At faster heart rates, this phenomenon occurs because of impulse falling in the relative refractory period of the bundle branch cells, also referred to as “phase-3 block.” The exact mechanism responsible for the block at slower heart rates is controversial, but the most accepted explanation is that it is related to spontaneous depolarization in phase 4, thus, rendering the cells refractory to the next impulse.
Epidemiology and Natural History
LBBB is rare in those <50 years of age and almost never occurs in those <35 years of age, suggesting that it is an acquired disorder. In population studies, its prevalence increases steadily from <1% at age 50 to ∼6% by 80 years. Factors that have been associated with its development include LV hypertrophy on the electrocardiogram, an increased cardiac volume, hypertension, valvular heart disease, cardiomyopathies, myocarditis, and coronary artery disease. Some, however, develop LBBB in the absence of any of these risk factors. What is of significant interest is whether a newly diagnosed LBBB, in the presence or absence of these risk factors, is associated with increased mortality or morbidity, because that will affect the approach in taking care of a patient with a newly discovered LBBB who is otherwise asymptomatic.
Well-conducted, population-based, longitudinal studies of those with LBBB have shown an increase or a trend toward increased cardiovascular mortality, sudden cardiac death, coronary artery disease, and HF. Only a few studies have shown a significant effect of LBBB on overall mortality, but this might be related to the limited number of patients and events in these studies. Those who developed LBBB at a younger age (<45 years) and who were free from cardiovascular risk factors did much better than those who developed LBBB during or after their fifth decade and had associated risk factors. Thus, it seems that LBBB can result from either intrinsic conduction system degeneration or an extrinsic insult from a variety of cardiovascular diseases, and the outcome of these 2 distinct populations with LBBB is divergent. In the absence of consensus guidelines on how to evaluate these patients, a noninvasive assessment for structural heart disease and ischemia is reasonable, especially in patients with known cardiovascular risk factors.
Epidemiology and Natural History
LBBB is rare in those <50 years of age and almost never occurs in those <35 years of age, suggesting that it is an acquired disorder. In population studies, its prevalence increases steadily from <1% at age 50 to ∼6% by 80 years. Factors that have been associated with its development include LV hypertrophy on the electrocardiogram, an increased cardiac volume, hypertension, valvular heart disease, cardiomyopathies, myocarditis, and coronary artery disease. Some, however, develop LBBB in the absence of any of these risk factors. What is of significant interest is whether a newly diagnosed LBBB, in the presence or absence of these risk factors, is associated with increased mortality or morbidity, because that will affect the approach in taking care of a patient with a newly discovered LBBB who is otherwise asymptomatic.
Well-conducted, population-based, longitudinal studies of those with LBBB have shown an increase or a trend toward increased cardiovascular mortality, sudden cardiac death, coronary artery disease, and HF. Only a few studies have shown a significant effect of LBBB on overall mortality, but this might be related to the limited number of patients and events in these studies. Those who developed LBBB at a younger age (<45 years) and who were free from cardiovascular risk factors did much better than those who developed LBBB during or after their fifth decade and had associated risk factors. Thus, it seems that LBBB can result from either intrinsic conduction system degeneration or an extrinsic insult from a variety of cardiovascular diseases, and the outcome of these 2 distinct populations with LBBB is divergent. In the absence of consensus guidelines on how to evaluate these patients, a noninvasive assessment for structural heart disease and ischemia is reasonable, especially in patients with known cardiovascular risk factors.
Acute Myocardial Infarction
The prevalence of LBBB in patients who present to the emergency department with clinical suspicion for AMI is relatively low and varies from 1% to 9%. Furthermore, <50% of these patients actually have an occluded epicardial coronary artery and/or elevated biomarkers. Even in patients with LBBB who present with an occluded epicardial coronary artery, the LBBB often might not be caused by the AMI. It is very rare for an anterior AMI to cause complete LBBB, given the dual supply from both the right and the left coronary arteries, suggesting that in many patients the LBBB is not caused by the AMI but was present before the acute event. This could partly explain why patients with AMI presenting with LBBB on their electrocardiogram usually receive delayed, inadequate, and less aggressive treatment than those with ST-segment elevation MI, although patients with LBBB and AMI have greater short- and long-term mortality than those with ST-segment elevation MI and a greater incidence of HF.
One reason cited for delayed reperfusion therapy in patients with AMI and LBBB is the presence of a nondiagnostic electrocardiogram. In patients with LBBB, impulse conduction traverses the septum from right to left. This interferes with the early vectors of ischemia, thus rendering the electrocardiogram ineligible for ischemia evaluation. The current guidelines from the American College of Cardiology and American Heart Association recommend reperfusion therapy for patients presenting to the emergency room with chest pain and new or presumed to be new LBBB. These guidelines were based on the beneficial effect of reperfusion therapy (compared to placebo in randomized trials) in patients with LBBB with suspected AMI and the obscuring effect of LBBB on ischemia detection. Because of the emphasis placed on early reperfusion in those with AMI, this has received considerable interest, and several criteria have been suggested for detecting AMI by electrocardiography in the presence of LBBB.
Of these, the Sgarbossa criteria have been the most widely used ( Figure 2 ). With LBBB, the ST-T changes are generally in the opposite direction (discordant) to the QRS complex. Thus, ST-segment elevation in the same direction as the QRS complex (concordant) is indicative of acute coronary occlusion in the presence of LBBB. Similarly, ST depression in V 1 to V 3 reflects myocardial ischemia and should not be attributed to LBBB ( Figure 2 ). The Sgarbossa criteria were initially validated in 45 patients with LBBB who had AMI and were enrolled in the Global Utilization of Streptokinase and Tissue Plasminogen Activator for Occluded Coronary Arteries (GUSTO-1). In the original report, these criteria had a low sensitivity for identifying AMI (31% and 72% for patients with discordant and concordant ST-segment elevation, respectively) but a high specificity (>90% for both concordant and discordant ST-segment elevation). Of the 3 criteria listed in Figure 2 , the highest score is given to concordant ST-segment elevation ≥1 mm, because it has been shown to have the greatest positive likelihood ratio for AMI. In contrast, discordant ST-segment elevation ≥5 mm is a relatively poor predictor and is given the lowest score. A total score >3 gives a 90% specificity for the diagnosis of AMI. Although patients with concordant ST-segment elevation are far more likely to have an occluded culprit vessel and elevated biomarkers than those without, the universal application of the Sgarbossa criteria for guiding reperfusion therapy in the setting of LBBB will result in missing a significant number of patients with AMI because of the low sensitivity ( Table 1 ). An analysis by Shlipak et al on a community-based cohort of patients with chest pain and LBBB suggested that treatment of all patients with LBBB and thrombolysis rather than the use of the Sgarbossa criteria would result in 10 more lives saved per 1,000 patients treated even after accounting for the risk of hemorrhagic stroke. Thus, the decision to proceed with reperfusion therapy (especially fibrinolysis) based solely on these criteria cannot be recommended.
Trial | Patients (n) | Inclusion Criteria | Myocardial Infarction (%) | Diagnostic Criteria | |||
---|---|---|---|---|---|---|---|
Sensitivity | Specificity | LR (+) | LR (−) | ||||
Shlipak et al, 1999 | 103 | CP + LBBB | 30 | 23% (10–41) | 82% (71–90) | 1.3 (0.6–2.9) | 0.9 (0.8–1.2) |
Li et al, 2000 | 190 | CP + LBBB | 13 | 20% (7–41) | 93% (88–96) | 3 (1–7) | 0.9 (0.7–1) |
Kontos et al, 2001 | 182 | CP + LBBB | 13 | 46% (28–65) | 93% (88–96) | 6.5 | 0.47 |
Gunnarsson et al, 2001 | 158 | CP + LBBB | 48 | 17% (8–26) | 94% (89–99) | 3 | 0.9 |
Gula et al, 2003 | 414 | AMI | NA ∗ | 44.5% | 61.2% | 1.2 (1.0–1.3) | 0.9 (0.8–1.0) |
Jain et al, 2011 | 36 | CP + LBBB | 39 | 14.2% | 88% | 1.2 | 0.97 |
∗ Gula et al studied 414 electrocardiograms with LBBB from a large cohort of patients with AMI (7.4% of total) and 85 electrocardiograms with LBBB not in the setting of acute coronary syndromes.
The current recommendations and guidelines pertaining to reperfusion in patients with LBBB were determined from trials done during the fibrinolysis era. In the present era, if primary percutaneous coronary intervention is performed, a definitive diagnosis of an occluded epicardial artery in patients with LBBB and suspected AMI can be made, rather than relying on the cardiac biomarkers, which can be elevated in several other disease processes. Recent studies have suggested that the prevalence of true AMI in patients with LBBB presenting to the emergency department with chest pain might even be lower than previously thought. Thus, triaging all patients with LBBB and a suspicion of AMI to receive reperfusion therapy might lead to more frequent “false-positive” cardiac catheterization laboratory activation in hospitals with primary percutaneous coronary intervention capabilities and in unwarranted risk in hospitals that depend on fibrinolytic therapy. Recently, Neeland et al suggested that a strategy for transfer to primary percutaneous coronary intervention centers might be superior to on-site fibrinolytic therapy for patients with LBBB, despite a delay in reperfusion. Although this approach is attractive and appears sound, it must be noted that currently no data are available to support it.
In summary, in the absence of any single electrocardiographic criteria that clearly distinguishes patients having AMI in the presence of LBBB, all patients with new or presumed to be new LBBB and strong clinical suspicion of AMI should undergo prompt reperfusion therapy, preferably with percutaneous coronary intervention, if this is available in a timely manner.
Left Ventricular Dyssynchrony
LBBB results in an altered pattern of LV activation and subsequent contraction. Under normal circumstances, impulse conduction spreads rapidly down the His bundle branches, followed by the Purkinje system and most of the LV endocardial surface is activated synchronously or within 40 ms. This results in efficient contraction at the expense of minimal energy. These dynamics are altered in the presence of LBBB, because conduction through the myocardium occurs at a slower pace than by the specialized conduction tissue, and the septal parts of the left ventricle are activated much earlier than the lateral wall, leading to electrical dyssynchrony. This electrical dyssynchrony leads to mechanical dyssynchrony, such that some areas of the left ventricle contract early and others later. Mechanical dyssynchrony, in turn, results in significantly lower dP/dT, a greater LV end-systolic volume, and energy inefficient contraction, a combination that is detrimental in patients with HF, who already have compromised LV systolic function. The discordant mechanical stretch leads to altered cellular Ca ++ transport, resulting in a pro-arrhythmic state. All these factors probably contribute to the increased mortality observed in patients with HF and LBBB compared to those without LBBB.
Cardiac Resynchronization Therapy
Large, randomized, multicenter trials have shown symptom and mortality benefit with CRT in patients with advanced HF and LV dyssynchrony as measured by a prolonged QRS duration ( Table 2 ). Current guidelines give a class I recommendation for CRT for patients who have a LV ejection fraction of ≤35%, a QRS duration of ≥0.12 second, sinus rhythm, and New York Heart Association functional class III or ambulatory class IV HF symptoms with optimum recommended medical therapy. Recently, the Resynchronization Reverses Remodeling in Systolic Left Ventricular Dysfunction (REVERSE), Resynchronization–Defibrillation for Ambulatory Heart Failure Trial (RAFT), and Multicenter Automatic Defibrillator Implantation Trial with Cardiac Resynchronization Therapy (MADIT-CRT) demonstrated the benefits of CRT in patients with milder HF symptoms. In line with these results, Medicare approved CRT-implantable cardioverter defibrillator placement for patients with a LV ejection fraction <30%, New York Heart Association class I or II symptoms, and LBBB with a QRS duration >130 ms. Patients with New York Heart Association class I or II HF symptoms are given a class IIb indication for CRT only if they are anticipated to need frequent ventricular pacing for other conditions. However, this might be revised in the upcoming guidelines based on the findings from the aforementioned trials. Major CRT trials have included patients according to the QRS duration and have failed to consider QRS morphology. In the initial trials, patients with right bundle branch block were underrepresented. More recent studies have shown that the benefit of CRT is far less in patients with right bundle branch block than in those with LBBB ( Table 3 ). A recent meta-analysis of major CRT trials did not show a mortality benefit for patients with non-LBBB QRS morphology. This difference in response to CRT probably reflects the presence of more dyssynchrony in LBBB than in right bundle branch block and also activation of the LV free wall, which is delayed in LBBB but is synchronous with the septum in right bundle branch block.
Clinical Trial | Patients (n) | Follow-Up (mo) | Patient Characteristics | Randomization | Primary End Point | Results | Comment | ||
---|---|---|---|---|---|---|---|---|---|
NYHA | LVEF | QRS (ms) | |||||||
MIRACLE | 453 | 6 | III–IV | <35% | >130 | CRT + OMT vs OMT | NYHA class | + | |
QOL score | + | ||||||||
6MWT | + | ||||||||
MIRACLE ICD | 369 | 6 | III–IV | <35 | >130 | CRT-D on vs off | NYHA class | + | |
QOL score | + | ||||||||
6MWT | − | ||||||||
CONTAK CD | 581 | 6 | II–IV | <35% | >120 | ICD vs CRT + ICD | HF hospitalization, mortality, and ICD therapies | − | +(QOL, LVEF and 6MWT) |
COMPANION | 1,520 | 16 | III–IV | <35% | >120 | OMT vs CRT vs CRT-D (1:2:2) | Mortality and hospitalization | − | |
CARE-HF | 813 | 18 | III–IV | <35 | >120 ∗ /150 | OMT + CRT vs OMT | Mortality + CV hospitalization | − | |
RAFT | 1,617 | 40 | II–III | <30% | >120 | ICD + CRT vs ICD | Mortality + HF hospitalization | − | ++If QRS >150 ms |
REVERSE | 610 | 12 | I–II | <40% | >120 | CRT on vs off | HF clinical composite response | − | +↓ (LVESVI) |
MADIT-CRT | 1,820 | 29 | I–II | <30% | >130 | CRT + ICD vs ICD (3:2) | Mortality + nonfatal HF events | − | Driven by HF events ++If QRS >150 ms |
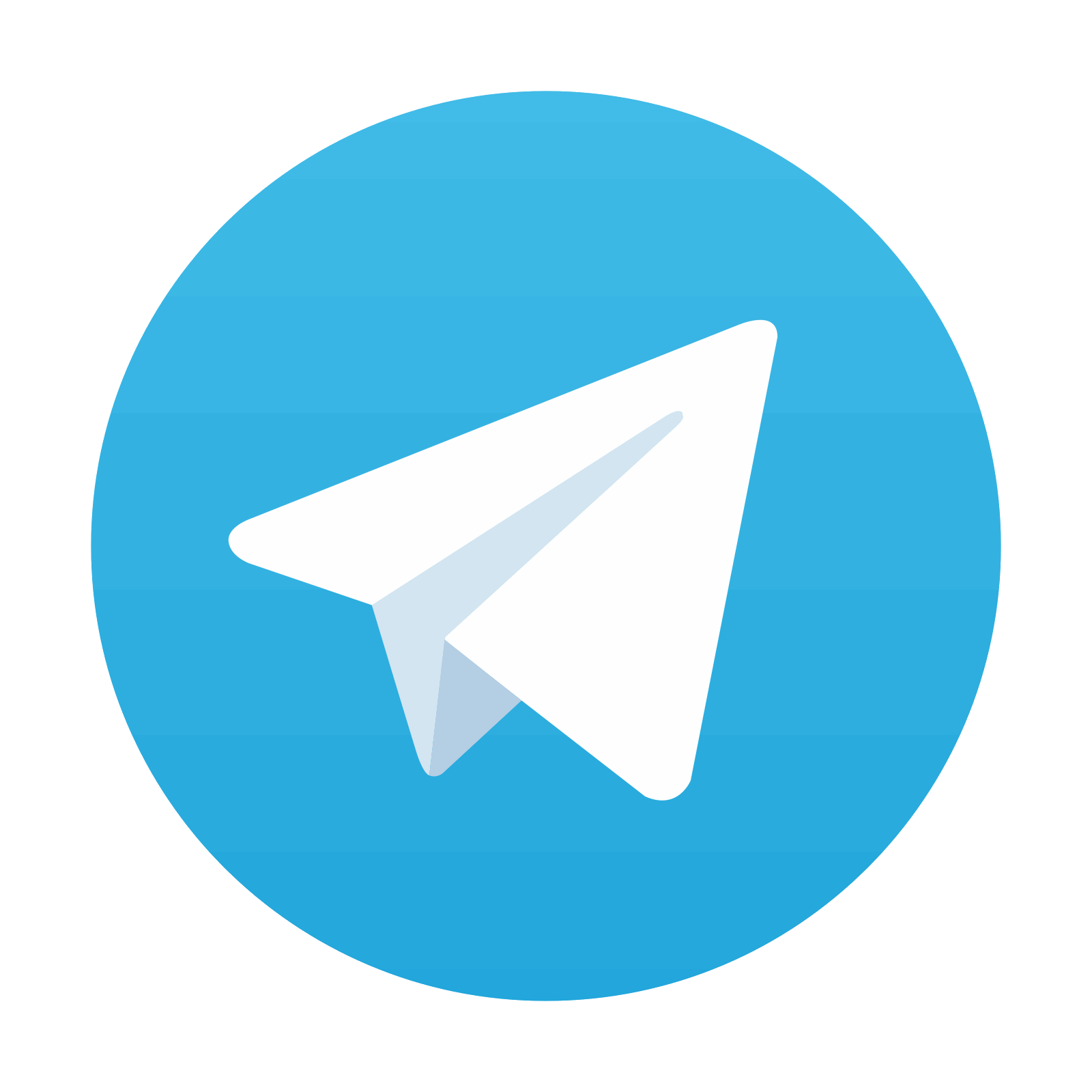
Stay updated, free articles. Join our Telegram channel

Full access? Get Clinical Tree
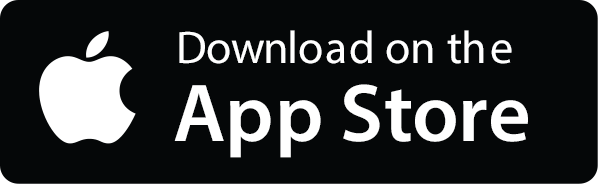
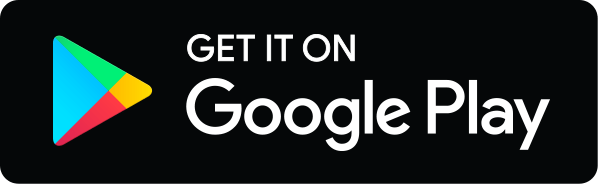
