Obesity is epidemic in congenital heart disease, with reported rates of 16% to 26% in children and 54% in adults. The aim of this study was to evaluate the impact of obesity on ventricular function and size in patients after initial repair for tetralogy of Fallot (TOF). Cardiac magnetic resonance studies in normal-weight (body mass index percentile <85th) and obese (body mass index percentile ≥95th) children and adults with repaired tetralogy of Fallot were reviewed. The left ventricular ejection fraction, the right ventricular ejection fraction, left and right ventricular end-diastolic volumes indexed to actual body surface area, to height, and to body surface area using ideal body weight were evaluated in 36 obese patients and 72 age-matched normal-weight patients. Compared with normal-weight patients, obese patients had lower right ventricular ejection fractions (mean 46 ± 9% vs 51 ± 7%, p = 0.003) and left ventricular ejection fractions (mean 57 ± 9% vs 61 ± 6%, p = 0.017), higher right ventricular end-diastolic volumes indexed to height (mean 160 ± 59 vs 135 ± 41 ml/m, p = 0.015) and left ventricular end-diastolic volumes indexed to height (mean 86 ± 25 vs 70 ± 20 ml/m, p = 0.001), and higher right ventricular end-diastolic volumes indexed to ideal body weight (mean 166 ± 55 vs 144 ± 38 ml/m 2 , p = 0.020) and left ventricular end-diastolic volumes indexed to ideal body weight (mean 90 ± 22 vs 75 ± 15 ml/m 2 , p <0.001). In conclusion, obesity is a modifiable risk factor associated with worsened biventricular systolic function and biventricular dilation in patients with repaired tetralogy of Fallot. The standard method of indexing ventricular volumes using actual body surface area may underestimate volume load in obese patients.
The prevalence of obesity in patients with congenital heart disease has been well documented, with obesity rates in patients with congenital heart disease being similar to those in the general population. The impact of obesity on patients with repaired tetralogy of Fallot (TOF) has not been specifically studied. Obesity may affect biventricular size and function and thereby affect the timing of pulmonary valve replacement. Furthermore, right ventricular (RV) size is typically indexed to body surface area, which may underrate the degree of RV dilation in obese patients. We hypothesized that patients with repaired TOF and obesity would have lower RV ejection fractions (RVEFs) and left ventricular ejection fractions (LVEFs) as assessed by cardiac magnetic resonance compared with normal-weight subjects. We also hypothesized that patients with repaired TOF and obesity would have larger RV end-diastolic volumes (RVEDVs) and larger left ventricular end-diastolic volumes (LVEDVs) compared with normal-weight subjects and that this finding would be heightened when indexing ventricular chamber size to height or to body surface area using ideal body weight rather than to actual body surface area. We sought to decrease the impact of sudden changes in weight on the assessment of cardiac status and hence on clinical decision making.
Methods
We performed a retrospective review of our radiology database from January 2004 through May 2011 for patients with TOF or TOF/pulmonary atresia who underwent cardiac magnetic resonance as part of routine clinical care after initial TOF repair, before subsequent intervention on the pulmonary valve or branch pulmonary artery. We excluded patients aged <2 years at the time of cardiac magnetic resonance, those without height and/or weight measurements at the time of cardiac magnetic resonance, those with absent pulmonary valve syndrome, and those with known genetic syndromes. To correct for the differential effect of obesity on the basis of age, we frequency matched each obese patient with 2 normal-weight patients on the basis of 5 categories of age at cardiac magnetic resonance. Within age category, normal-weight patients were selected at random. In patients with serial exams, we gathered data from the most recent cardiac magnetic resonance exam before an intervention on the pulmonary valve or on a branch pulmonary artery.
Height and weight at the time of cardiac magnetic resonance were used to calculate body mass index (BMI). BMI percentile (BMI%) was calculated using the most recent growth charts of the Centers for Disease Control and Prevention, which include data for subjects aged 2 to 20 years. For patients aged ≤20 years, those with BMI% ≥95th percentile were classified as obese, and patients with BMI% <85th percentile were classified as normal weight. Patients aged >20 years with BMIs ≥30 kg/m 2 were classified as obese, while those with BMIs <25 kg/m 2 were classified as normal weight. For the purposes of descriptive analysis of BMI%, BMI was converted to BMI% on the basis of available normative data.
All cardiac magnetic resonance studies were performed using a 1.5-T system (Achieva/Intera; Philips Medical Systems, Best, The Netherlands). Volumetric analyses were performed using a ViewForum workstation (Phillips Medical Systems) using balanced steady-state free precession acquisitions in the short-axis plane. The endocardium was traced, excluding the papillary muscles, on each of 12 to 14 slices on end-diastolic and end-systolic. Phase contrast flow in the main pulmonary artery, right pulmonary artery, and left pulmonary artery were collected. Despite technological advances during the study period, our methods in acquiring these data did not change significantly during the study period. We indexed RVEDV and LVEDV to actual body surface area (RVEDVi and LVEDVi) calculated using the Haycock formula, to height in meters (RVEDVh and LVEDVh), and to body surface area calculated using ideal body weight (RVEDVibw and LVEDVibw). Ideal body weight was calculated using the BMI method, because it is considered the most reproducible in patients of varying ages and at the extremes of BMI. The BMI method uses a patient’s height to calculate his or her ideal body weight for age. Indexing a measurement to body surface area calculated using ideal body weight eliminates the weight variable but includes the height variable. We chose this method in addition to indexing volumes to height to provide volumetric measurements that are applicable to patients with repaired TOF. Current data indicate that in asymptomatic patients with repaired TOF, indications for pulmonary valve replacement include, among multiple other factors, significant elevation of RVEDV and impaired RV or LV systolic function. The suggested RVEDV threshold for pulmonary valve replacement varies from 150 to 170 ml/m 2 . We dichotomized patients into those with RVEDVi and RVEDVibw <160 ml/m 2 and those with RVEDVi <160 ml/m 2 but RVEDVibw ≥160 ml/m 2 , to identify the group of patients who may meet criteria for pulmonary valve replacement on the basis of indexing RVEDV to body surface area calculated using ideal body weight to those who would not. We also dichotomized patients into those with or without RV or LV dysfunction (RVEF <45%, LVEF <55%). We evaluated flow-versus-time diagrams derived from phase contrast data for the presence of restrictive physiology, as defined by a distinct peak of forward flow immediately before but distinct from the upstroke of the systolic forward flow in the main pulmonary artery.
Descriptive data are reported using proportions, median (range) for data not normally distributed, and mean ± SD for normally distributed data. Patient and surgical characteristics were compared across BMI categories using Fisher’s exact test for categorical variables. For continuous variables, t tests were used for normally distributed data, and Mann-Whitney U tests were used for nonparametric data. Correlation of continuous data sets was performed using Pearson’s r for normally distributed data and Spearman’s ρ for data that were not normally distributed. All statistical analyses were performed using SPSS version 19.0 (SPSS, Inc., Chicago, Illinois). This study was performed with the approval of the Baylor College of Medicine Institutional Review Board.
Results
We included 36 obese patients in our study. Of these, 2 (6%) were aged 2 to 6 years at the time of cardiac magnetic resonance, 3 (8%) were aged 6 to 10 years, 19 (53%) were aged 11 to 20 years, 6 (17%) were aged 21 to 30 years, and 6 (17%) were aged ≥31 years. Demographic and clinical characteristics, including pulmonary regurgitant fraction, the presence of restrictive physiology, and Nakata index, are listed in Table 1 . To provide an estimate of pulmonary arterial caliber independent of obesity, we included Nakata index calculated using true body surface area and Nakata index calculated using ideal body weight.
Variable | Normal Weight (n = 72) | Obese (n = 36) | p Value |
---|---|---|---|
Age at initial repair (yrs) | 1.1 (0.02–8.5) | 1.6 (0.33–9.9) | 0.38 |
Staged repair | 24 (33%) | 10 (28%) | 0.36 |
Male gender | 39 (54%) | 25 (69%) | 0.13 |
Race | 0.50 | ||
White | 42 (58%) | 21 (58%) | |
Black | 12 (17%) | 2 (6%) | |
Hispanic | 8 (11%) | 6 (17%) | |
Other | 7 (10%) | 5 (14%) | |
Pulmonary atresia | 3 (4%) | 0 (0%) | 0.29 |
Prograde diastolic flow | 24/59 (41%) | 9/26 (35%) | 0.39 |
Main pulmonary artery regurgitant fraction (%) | 42 ± 16 | 37 ± 19 | 0.18 |
Nakata index (mm 2 /m 2 ) | 347 ± 149 | 285 ± 117 | 0.06 |
Nakata index calculated using ideal body weight (mm 2 /m 2 ) | 343 ± 149 | 347 ± 143 | 0.90 |
Analysis of obesity and outcomes is listed in Table 2 . The mean RVEF of the obese group (46 ± 9%) was significantly lower than that of the normal-weight group (51 ± 7%) (p = 0.003; Figure 1 ). The mean LVEF of the obese group (57 ± 9%) was also significantly lower than that of the normal-weight group (61 ± 6%) (p = 0.017; Figure 1 ). There was no difference in RVEDVi or LVEDVi between normal-weight and obese patients. We did find a difference in RVEDVh between normal-weight (135 ± 41 ml/m) and obese (160 ± 59 ml/m) patients (p = 0.015; Figure 2 ), in LVEDVh between normal-weight (70 ± 20 ml/m) and obese (86 ± 25 ml/m) patients (p = 0.001; Figure 2 ), in RVEDVibw between normal-weight (144 ± 38 ml/m 2 ) and obese (166 ± 55 ml/m 2 ) patients (p = 0.02; Figure 2 ) and in LVEDVibw between normal-weight (74 ± 17 ml/m 2 ) and obese (90 ± 22 ml/m 2 ) patients (p <0.001; Figure 2 ). There was no association between obesity and main pulmonary artery regurgitant fraction (p = 0.16). There was a positive correlation between RVEF and LVEF (r = 0.46, p <0.001).
Variable | Normal Weight (n = 72) | Obese (n = 36) | p Value |
---|---|---|---|
RVEF (%) | 51 ± 7 | 46 ± 9 | 0.003 |
RVEF <45% | 13 (18%) | 14 (39%) | 0.018 |
RVEDVi (ml/m 2 ) | 147 ± 40 | 136 ± 43 | 0.189 |
RVEDVh (ml/m) | 135 ± 41 | 160 ± 59 | 0.015 |
RVEDVibw (ml/m 2 ) | 144 ± 38 | 166 ± 55 | 0.020 |
LVEF (%) | 61 ± 6 | 57 ± 9 | 0.017 |
LVEF <55% | 10 (14%) | 11 (31%) | 0.038 |
LVEDVi (ml/m 2 ) | 76 ± 18 | 74 ± 18 | 0.593 |
LVEDVh (ml/m) | 70 ± 20 | 86 ± 25 | 0.001 |
LVEDVibw (ml/m 2 ) | 75 ± 15 | 90 ± 22 | <0.001 |
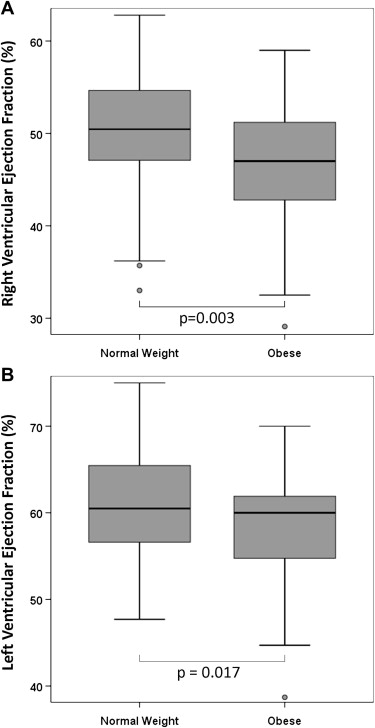
