There is uncertainty and debate regarding whether ischemic mitral regurgitation (MR) is a secondary epiphenomenon resulting from left ventricular (LV) dysfunction or confers an independent effect on exercise capacity and outcomes. We tested whether ischemic MR negatively affects exercise capacity and cardiovascular morbidity and mortality in patients with coronary artery disease (CAD) and those with inferior wall motion abnormality independent of LV dysfunction. Clinical follow-up over 5 years was obtained in 77 patients (64 ± 10 years old, LV ejection fraction 54 ± 11%) with at least mild ischemic MR from CAD and evidence of inferior wall motion abnormality who had exercise stress testing with perfusion imaging within 24 hours of echocardiography. Patients with active heart failure, ischemia, intrinsic valve disease, pulmonary and vascular diseases were excluded. Exercise capacity (METs, peak double product) was tested for relation to MR (vena contracta [VC] and jet area), LV size and function, and pulmonary pressures. Cox proportional hazards analysis assessed whether MR predicted cardiovascular events including hospitalization for heart failure, acute coronary syndrome, and myocardial infarction and cardiovascular and total mortalities. Univariate correlation identified MR with VC (r = −0.674, p <0.0001) and MR jet area (r = −0.575, p <0.0001) as determinants of decreased functional capacity evaluated by METs, with VC the stronger predictor. MR VC >2 mm (moderate ischemic MR) and age were independent predictors of cardiovascular events and death (hazard ratio 6.72 for MR, p = 0.04). In conclusion, in patients with CAD and LV inferior wall motion abnormality, MR negatively affects exercise capacity and is associated with increased cardiovascular morbidity and mortality. This effect appears independent of degree of LV dysfunction.
Evaluation of functional capacity by exercise testing is widely used in the diagnosis and functional evaluation of coronary artery disease (CAD) and is a strong predictor of prognosis. The direct influence of ischemic mitral regurgitation (MR) on exercise capacity, however, is unknown. An independent effect of MR on functional capacity in patients with CAD would strengthen the case for the clinical importance of the lesion and have a significant impact on clinical decision making, providing a rationale for treating this vexing valvular problem. Therefore, the purpose of this study was to test the hypothesis that ischemic MR has an effect on exercise capacity independent of severity of segmental and global measurements of left ventricular (LV) dysfunction and an independent effect on cardiovascular morbidity and mortality. We examined patients with inferior wall motion abnormalities because this population allows examination of a homogenous group of patients with similar LV function and remodeling characteristics.
Methods
We reviewed the echocardiographic database from the previous 5 years for patients with stable CAD and evidence of inferior wall motion abnormality who underwent exercise stress testing with perfusion imaging within 24 hours of echocardiography. Patients with inferior wall motion abnormality were selected because, based on the mechanism of ischemic MR, these patients are prone to develop ischemic MR; importantly, therefore, they provide a population that allows testing the relation of MR to exercise capacity in the absence of global LV dysfunction or active heart failure. Patients with active heart failure, ischemia, intrinsic mitral valve disease, significant concomitant aortic valve disease, congenital heart disease, valve prostheses, vegetations, and poor image quality were excluded (n = 5). Patients with significant pulmonary disease, peripheral vascular disease, and renal failure were also excluded (n = 7).
Transthoracic echocardiograms at rest were recorded according to routine laboratory protocol using a Vivid 7 system (GE Healthcare, Milwaukee, Wisconsin) or a Philips ie33 system (Philips Medical Systems, Andover, Massachusetts). Standard 2-dimensional parasternal and apical views were obtained using a 2.5-MHz transducer with harmonic imaging for optimal penetration and image quality. Data were recorded in digital format and stored on optical or digital video disks from apical 2-chamber and 4-chamber views for off-line analysis (Xcelera, Philips Medical Systems). All measurements were averaged over ≥3 cardiac cycles. Echocardiographic measurements were made blind to results of exercise.
End-diastolic (largest dimension of the LV cavity at onset of the QRS complex) and end-systolic (smallest dimension of the LV cavity) LV volumes and ejection fraction (EF) were calculated by the Simpson biplane method of disks from the apical 2-chamber and 4-chamber views according to recommendations of the American Society of Echocardiography. LV end-diastolic and end-systolic diameters were assessed from parasternal long-axis views. LV global remodeling was quantified by calculation of the LV sphericity index as the ratio of the end-diastolic LV major axis to the minor axis in the apical 4-chamber view as previously described. Left atrial volume was measured by the biplane area–length method. Right ventricular systolic pressure was estimated from the systolic transtricuspid pressure gradient (millimeters of mercury) using the simplified Bernoulli equation (Transtricuspid Pressure = 4V 2 , where V = maximal tricuspid regurgitant velocity in meters per second).
Diastolic function was evaluated from the mitral inflow recorded at the mitral leaflet tips. E-wave (early diastolic flow), A-wave (late diastolic flow), E-wave deceleration time, and E/A velocity ratio were measured. Peak early diastolic mitral annular velocities were obtained by pulse-wave tissue Doppler imaging from the apical 4-chamber view using the septal and lateral sites. Averaged e′ was used to calculate the ratio of peak early diastolic transmitral flow velocity E to e′ to estimate LV filling pressures.
LV outflow tract stroke volume was calculated by multiplying the LV outflow tract area by the LV outflow tract velocity–time integral measured by pulse-wave Doppler and LV outflow tract diameter was measured in the parasternal long-axis view with a zoom on the aorta.
Quantification of MR was performed by vena contracta (VC) width, a central and direct measurement of the jet emerging from the regurgitant orifice, which was measured from the apical long-axis view ( Figure 1 ). Color flow imaging frame rate was maximized by selecting the narrowest sector angle and regional image expansion was used to maximize its visualization. The largest VC diameter was averaged over 3 cardiac cycles. As a correlative method, we also used regurgitant color jet area/left atrial area, a measurement that is well-suited to the central (noneccentric) jets of ischemic MR. Left atrial area and regurgitant jet area were measured by planimetry from the apical 4-chamber and 2-chamber views and then averaged to calculate their ratio. The valvular tenting area enclosed between the annular line and the mitral leaflets was obtained from the apical 4-chamber view in midsystole.
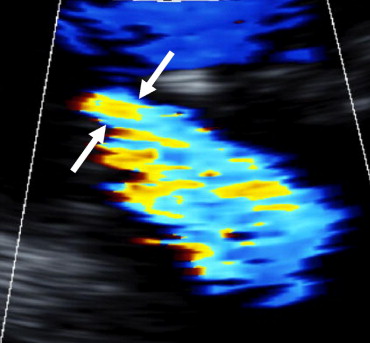
LV regional contractility was scored using 3 short-axis views. The left ventricle was divided into 20 standard segments and a score was allocated to each segment according to its contractility as (0) normokinetic, (+1) hypokinetic, (+2) akinetic, or (+3) dyskinetic. A global wall motion score was calculated by summing these values and the wall motion score index was obtained by dividing wall motion score by number of scored segments. The 20-segment model is 1 of several wall motion score models proposed and is 1 that has been used at our institution for >25 years. The advantage of a 20-segment model is a more precise mapping of apical function. Furthermore, consistency in the type of model is more important than the actual wall motion model employed.
Exercise testing with sestamibi single-photon emission computed tomography for detecting ischemia was performed within 24 hours of echocardiography. Beta-adrenergic blocking agents were stopped 24 hours before the test. Symptom-limited treadmill exercise was performed using the Bruce protocol. Electrocardiograms were continuously monitored during exercise and for an additional 5 minutes in recovery. Blood pressures were continuously taken during the last 30 seconds of each exercise stage and then each minute during the 5 minutes of recovery. Patients were encouraged to perform a maximal exercise or stopped for dyspnea, exhaustion, or ischemia. Peak exercise capacity was evaluated as METs and as peak double product (heart rate multiplied by systolic blood pressure). Patients with inducible ischemia at perfusion imaging were excluded from this analysis to exclude this confounding factor.
Overall follow-up over a maximum of 5 years extended from baseline evaluation until last available contact. The following adverse events were recorded: hospitalization for heart failure including new onset of heart failure, acute coronary syndrome, myocardial infarction, stroke, and cardiovascular and total mortalities. During follow-up patients were monitored by their personal physicians. Events were ascertained by our database of medical records.
Continuous variables are summarized as mean ± SD. Categorical data are reported as number (percentage). Each echocardiographic and Doppler data point represents the average of 3 to 4 beats. The relation among hemodynamic parameters, MR severity, and functional capacity (METs) was tested by linear regression. To identify potentially determinants of exercise capacity all variables were subjected to a forward stepwise variable selection method. At each step variables were entered until there was no significant improvement in the value of r 2 . VC and MR jet area/left atrial area ratio were not used together because they are collinear. In analysis of determinants of exercise capacity LVEF was entered as a continuous variable and alternatively stratified as a categorical variable, with patients categorized into 3 groups: those with severe LV dysfunction (EF ≤35%), mild to moderate LV dysfunction (EF 36% to 49%), and normal LV function or mild LV dysfunction (EF ≥50%). Cox proportional hazards analysis was used to assess whether MR predicted events (hospitalization for heart failure, acute coronary syndrome, myocardial infarction, stroke, and cardiovascular and total mortalities): we also used correlation analysis by the Pearson method to evaluate the relation between demographic and clinical parameters, LV geometry and function, MR severity, and functional capacity (METs and peak double product). Univariate and multivariate analyses of time to events were performed using Cox proportional hazards multivariate models with VC as an independent variable in continuous and categorical formats with a cut-off value of 2 mm, including lower cut-off values for patients with ischemic MR than for those with normal LV function. The 2-mm cutoff was chosen because it was the median value of the VC distribution in this study. For multivariable analyses of events we used predefined Cox proportional hazards multivariable models that included covariates considered to have potential prognostic impact (age, LV end-diastolic volume, LVEF, and VC >2 mm). A p value <0.05 was considered statistically significant. Data were analyzed with SAS 9.1 (SAS Institute, Cary, North Carolina).
Results
Eligibility criteria were fulfilled by 77 patients (mean age 64 ± 11 years, 59 men, 77%). Twelve patients (15%) had atrial fibrillation. Mean EF was 54 ± 11% ( Table 1 ). Mean LV end-diastolic volume was 110 ± 37 ml (from 44.5 to 233 ml), and mean LV end-systolic volume was 51 ± 23 ml (from 15 to 130 ml). Six patients (7.8%) had EF ≤35% and 18 (23%) had an EF from 36% to 49%. Mean MR was mild to moderate (mean VC 2.0 ± 1.4 mm, MR jet area/left atrial area ratio 15 ± 13%). Heart rate and systolic blood pressure increased from rest to peak exercise (65 ± 11 vs 124 ± 25 beats/min, 123 ± 22 vs 155 ± 27 mm Hg). Mean duration of exercise was 426 ± 185 seconds and mean METs were 8.1 ± 3.2. Other echocardiographic and stress test characteristics are listed in Table 1 . Mean overall duration of follow up was 29.5 ± 13.0 months (from 9 to 61). Ten events were recorded including 5 deaths (4 cardiac deaths).
Parameters | Total Population | Patients Without Events | Patients With Events | p Value |
---|---|---|---|---|
(n = 77) | (n = 67, 87%) | (n = 10, 13%) | ||
Left ventricular end-diastolic volume (ml) | 110 ± 37 | 108 ± 37 | 119 ± 41 | 0.373 |
Left ventricular end-systolic volume (ml) | 51 ± 23 | 49 ± 22 | 61 ± 28 | 0.138 |
Left ventricular ejection fraction (%) | 54 ± 11 | 55 ± 11 | 50 ± 10 | 0.215 |
Left ventricular end-diastolic diameter (mm) | 49 ± 6 | 49 ± 5 | 54 ± 9 | 0.013 |
Left ventricular end-systolic diameter (mm) | 35 ± 7 | 34 ± 6 | 41 ± 8 | 0.004 |
Right ventricular systolic pressure (mm Hg) | 36 ± 14 | 34 ± 12 | 47 ± 21 | 0.011 |
Left atrial volume (ml) | 70 ± 27 | 67 ± 26 | 89 ± 44 | 0.027 |
Tenting area (mm 2 ) | 0.73 ± 0.42 | 0.66 ± 0.34 | 1.20 ± 0.60 | <0.0001 |
E/e′ lateral | 9 ± 5 | 8 ± 3 | 13 ± 9 | 0.003 |
E/e′ septal | 7 ± 2 | 11 ± 6 | 12 ± 5 | 0.695 |
E/averaged e′ | 10 ± 5 | 10 ± 5 | 14 ± 7 | 0.019 |
E deceleration time (ms) | 232 ± 75 | 235 ± 77 | 209 ± 60 | 0.323 |
Wall motion score index (score) | 0.41 ± 0.3 | 0.40 ± 0.3 | 0.47 ± 0.31 | 0.537 |
Vena contracta (mm) | 2 ± 1.4 | 1.8 ± 1.3 | 3.1 ± 2.1 | 0.08 |
Mitral regurgitation jet area/left atrial area ratio (%) | 15 ± 13 | 13 ± 12 | 23 ± 19 | 0.024 |
MR quantification (p <0.001) and LV end-diastolic volume (p = 0.03) were significantly correlated with METs when EF was used as a categorical variable (severe, moderately, or mildly decreased as categorized in Methods). Stepwise multiple linear regression analysis identified MR with VC (beta coefficient −18.014, SR 2.336, p <0.0001) and LV end-diastolic volume (beta coefficient 0.132, SE 0.052, p = 0.015) as independent determinants of decreased functional capacity evaluated by METs. This effect of VC was present as a categorical variable (>2 mm) and as a continuous variable in the regression model. Univariate regression identified MR with VC (r = −0.674, p <0.0001; Figure 2 ) and MR jet area (r = −0.575, p <0.0001) as determinants of decreased functional capacity evaluated by METs with VC the stronger predictor. Tenting area showed a trend toward significance (p = 0.053). There was no difference in the relation between decrease in METs and increase in VC in patient groups with LVEF mildly, moderately, or severely impaired.
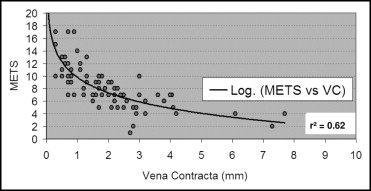
LV end-diastolic and LV end-systolic diameters were inversely significantly correlated with exercise capacity evaluated by peak double product (r = −0.315, p = 0.005; r = −0.27, p = 0.016, respectively, for LV end-diastolic and end-systolic diameters). There was no correlation when METs were used ( Table 2 ). There was a significant correlation between age, male gender, atrial fibrillation, changes in heart rate, E/e′ lateral, averaged E/e′, tenting area, right ventricular systolic pressure, and wall motion score index and exercise capacity evaluated by METs. E deceleration time was also correlated with right ventricular systolic pressure (r = −0.308, p = 0.008).
Variables | METs | Peak Double Product | ||
---|---|---|---|---|
r | p Value | r | p Value | |
Demographic data | ||||
Age | −0.255 | 0.025 | −0.007 | 0.955 |
Male gender | 0.293 | 0.010 | 0.040 | 0.727 |
Clinical data | ||||
β Blockers | 0.168 | 0.145 | −0.030 | 0.794 |
Atrial fibrillation | −0.343 | 0.002 | 0.099 | 0.392 |
Diabetes | −0.145 | 0.207 | −0.080 | 0.487 |
Smoking | −0.043 | 0.71 | 0.175 | 0.128 |
Hypertension | −0.081 | 0.486 | 0.030 | 0.794 |
Dyslipidemia | 0.111 | 0.335 | −0.112 | 0.732 |
History of coronary artery disease | 0.233 | 0.042 | 0.151 | 0.190 |
Heart rhythm changes | 0.623 | <0.001 | 0.703 | <0.001 |
Left ventricular geometry and function | ||||
Left ventricular end-diastolic volume | 0.235 | 0.04 | −0.059 | 0.611 |
Left ventricular end-systolic volume | 0.070 | 0.542 | −0.162 | 0.160 |
Left ventricular ejection fraction | 0.159 | 0.703 | 0.233 | 0.041 |
Left ventricular end-diastolic diameter | 0.099 | 0.39 | −0.315 | 0.005 |
Left ventricular end-systolic diameter | −0.50 | 0.66 | −0.276 | 0.016 |
E/e′ lateral | −0.352 | 0.003 | −0.280 | 0.019 |
E/e′ medial | −0.221 | 0.062 | −0.024 | 0.843 |
E/averaged e′ | −0.341 | 0.003 | −0.232 | 0.048 |
E deceleration time | −0.313 | 0.006 | 0.160 | 0.166 |
Wall motion score index | −0.245 | 0.032 | −0.418 | <0.001 |
Sphericity index | −0.047 | 0.686 | 0.201 | 0.082 |
Cardiac output at rest | 0.002 | 0.987 | 0.161 | 0.163 |
Mitral regurgitation severity | ||||
Vena contracta | −0.674 | <0.001 | −0.260 | 0.022 |
Mitral regurgitation jet area/left atrial area ratio | −0.575 | <0.001 | −0.223 | 0.052 |
Tenting area | −0.430 | <0.001 | −0.147 | 0.202 |
Right ventricular systolic pressures | −0.424 | <0.001 | −0.253 | 0.031 |
Left atrial volume | −0.168 | 0.143 | −0.074 | 0.520 |
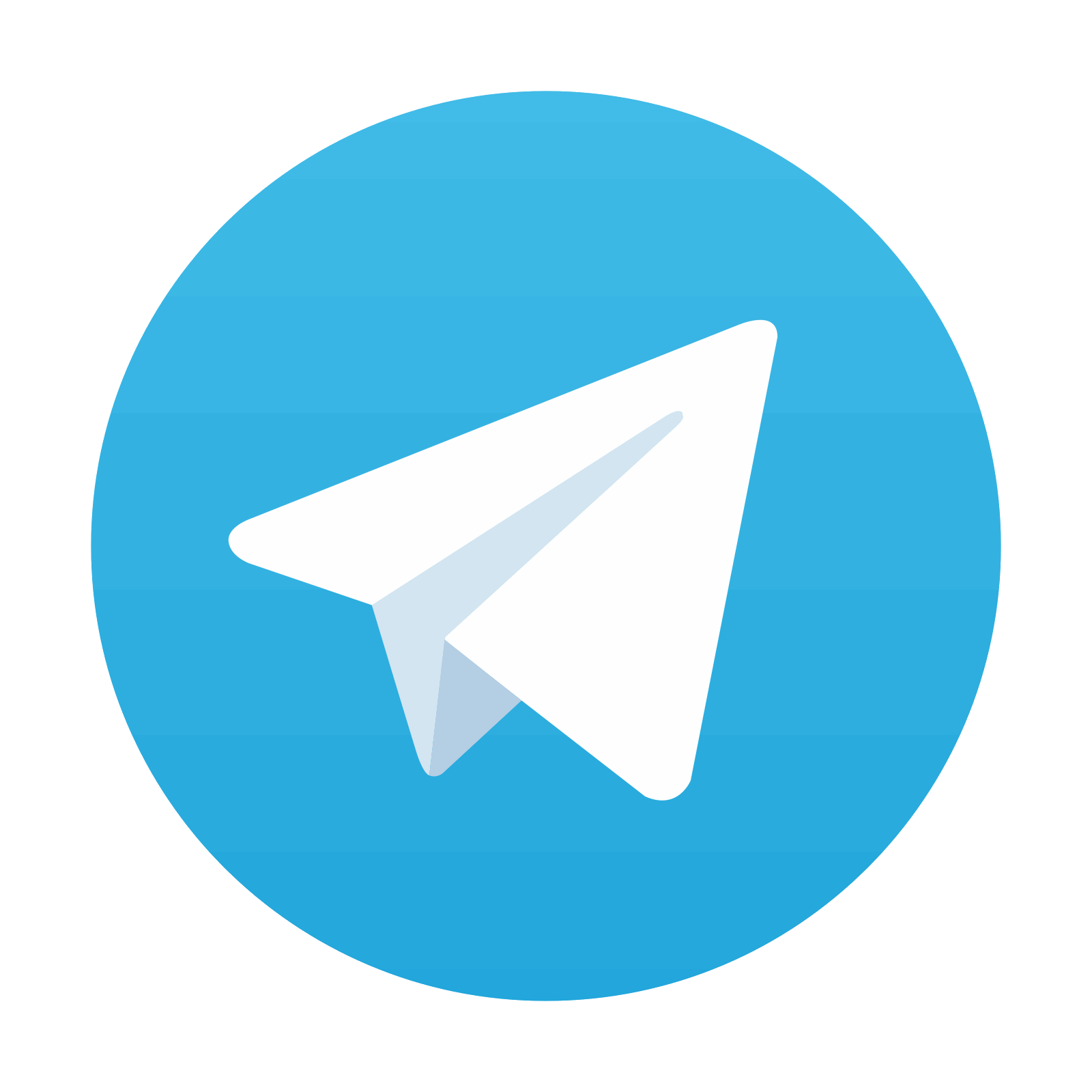
Stay updated, free articles. Join our Telegram channel

Full access? Get Clinical Tree
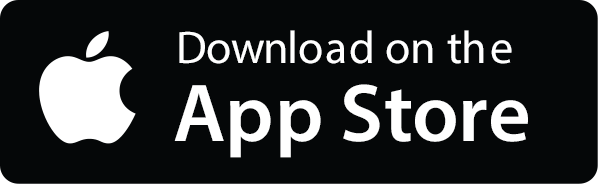
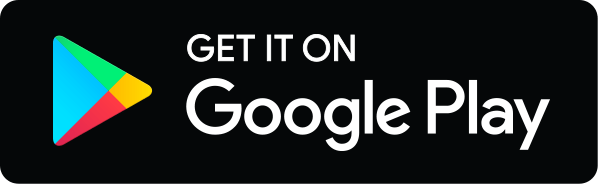
