Fig. 22.1
Irreversible defect in the infero-postero-lateral wall on a [99mTc]-tetrofosmin myocardial perfusion SPECT scan (a), indicating myocardial infarction. Infarction in the inferior wall and basal infero-septal region on a [13N]NH3 PET scan but preserved global perfusion reserve in the remaining tissue (b)
Recent developments in both soft- and hardware led to the implementation of hybrid systems, combining SPECT and PET myocardial perfusion imaging with CT. Contrast-enhanced angiography using CT can be an attractive alternative to (invasive) coronary angiography (CAG), also providing anatomical information (Schroeder et al. 2008). In addition, the quantity of coronary artery calcium load, as determined with CT, coined as ‘Agatston’ or ‘calcium score’, is used for risk stratification for future cardiac events (Greenland et al. 2007). The clinical use of hybrid imaging (either myocardial perfusion imaging with calcium score or CT angiography with calcium score) provides higher accuracy and faster diagnosis of CAD, especially in cases with equivocal results of separate tests (Flotats et al. 2011).
22.5.1 Imaging of Effects of Emotional of Stress Responses on Myocardial Ischemia and Autonomic Function, Using PET and SPECT
An early report on imaging techniques to assess nervous control over myocardial ischemia studied the effect of mental arithmetic tasks on myocardial perfusion (Deanfield et al. 1984). During the mental stress tasks, executed in patients with CAD, size and location of perfusion defects on myocardial perfusion scintigraphy were comparable to perfusion defects induced during an exercise performance. Patients with CAD suffered from mental stress more often than patients without CAD; especially, they may suffer from hostility, depression and anxiety, psychological conditions that all are capable to induce myocardial infarction (MI) (Das and O’Keefe 2008). In this respect, it is worth notifying that depression is an independent risk factor for mortality (Serrano et al. 2011).
Actually, it is reported that various types of anxiety disorders have different impact on the outcomes after MI. In this regard, a generalized anxiety disorder appears to predict a superior 5-year outcome after MI. The authors hypothesized that these patients are expected to seek early help after the manifestation of somatic symptoms and are also more willing to participate in rehabilitation programmes (Parker et al. 2011). On the other hand, panic disorders often co-occur with CAD (Soh and Lee 2010; Fleet et al. 2000). Post-traumatic stress disorders (PTSD) – which is no longer classified as an anxiety disorder but according to the Diagnostic and Statistical Manual of Mental Disorders (DSM-V, American Psychiatric Association 2013) criteria considered to be a ‘trauma- and stressor-related disorder’ – has also been associated with CAD (Ahmadi et al. 2011). The underlying mechanism of the association of panic disorder with CAD is not yet clear. Reduced heart rate variability (HRV), which is common in both panic disorder and PTSD patient groups, was not significantly different in CAD populations with and without panic disorder. This suggests that reduced HRV may not be the underlying explanation of increased cardiovascular morbidity and mortality in panic disorder patients (Lavoie et al. 2004). Other anxiety disorders, according to the recent DSM-V including inter alia social anxiety disorder, separation anxiety disorder and selective mutism, are not associated with CAD.
22.5.2 Imaging Myocardial Ischemia in Patients with Mental Stress
Myocardial perfusion imaging is often used in patients with mental stress (Fig. 22.2). In fact, the early studies using PET were designed to identify mental stress-induced ischemia (Deanfield et al. 1984). In the latter study, myocardial perfusion imaging with PET showed signs of ischemia in 12 out of 16 patients, though perfusion defects induced by mental stress (arithmetic task) were less pronounced when compared to perfusion defects following physical exercise.
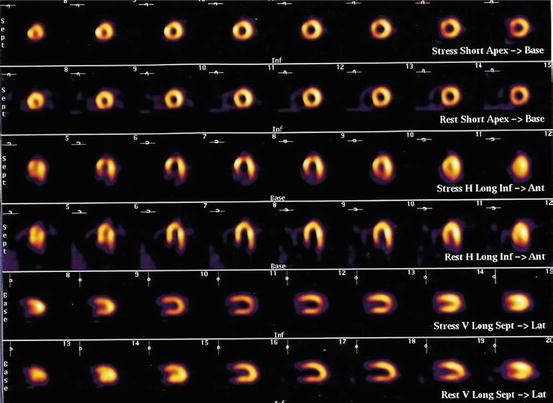
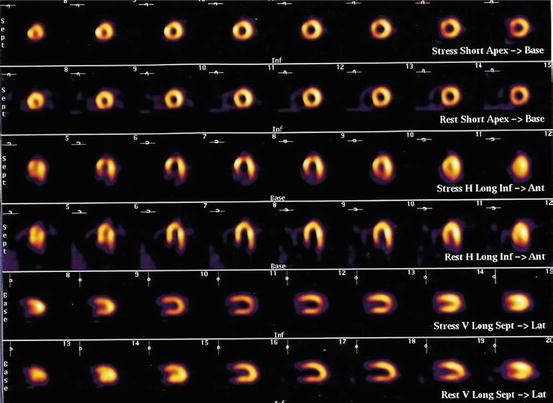
Fig. 22.2
Reversible defect in the apex on a [99mTc]-tetrofosmin myocardial perfusion scan, consistent with mental stress-induced ischemia (Reprinted from Vashist et al. (2004) with permission from Elsevier)
As mentioned before, myocardial perfusion imaging can be performed after physical exercise as well as with pharmacological stress. However, neither of the two methods can be considered to represent emotional or mental stress. In the setting of a laboratory, several mentally demanding and emotionally provocative tasks for inducing stress have been evaluated. The best reproducibility results were observed during anger recall and speaking in public (Burg et al. 1993; Kim et al. 2003). Still, no major clinical trial has been performed to investigate which method to stress is the most reliable to demonstrate mental stress-induced myocardial ischemia.
Mental stress tests activate the sympathetic nervous system which is known to cause vasodilatation in healthy coronary arteries and vasoconstriction in case of endothelial dysfunction. Comparison with other sympathetic tests should, however, be interpreted with caution since different responses between the tests have been described (Monnink et al. 2002). Still, at the cardiac level, mental stress causes an increased coronary vascular resistance and ensuing myocardial ischemia (Arrighi et al. 2002). The latter was reported in a study in which ten patients and five control subjects underwent both a CAG and a myocardial perfusion imaging using [13N]NH3 PET scans. PET was performed at rest, during mental stress and during pharmacological stress with dipyridamole. Coronary flow reserve was calculated as the ratio of stress to rest blood flow. A narrowing of >50 % in a coronary artery revealed by CAG was considered significant. As expected, during dipyridamole infusion myocardial blood flow and coronary flow reserve were less in the regions with significant CAD, when compared to the regions without disease. However, during mental stress the opposite pattern was found: coronary flow reserve was less in regions without significant stenosis, than in those with significant stenosis. Even after correction for individual rate-pressure products, this was present in regions without narrowing of a coronary artery, when compared to regions with stenosis (0.8 ± 0.3 vs 1.1 ± 0.3, p = 0.001). The authors hypothesize that during mental stress, regions with significant stenosis show an increase in absolute myocardial blood flow, which increase is not found in regions without coronary artery stenosis. Also, the regions with lower flow reserve during mental stress showed a paradoxical increase in coronary microvascular resistance. In the control subjects, myocardial blood flow increased during both mental and pharmacological stress. Furthermore, coronary flow reserve in the controls was higher than in patients with CAD, also when normalized for rate-pressure products.
This study demonstrates reduction of myocardial blood flow during mental stress in regions without significant coronary artery stenosis. The investigators therefore conclude that microvascular endothelial dysfunction has a crucial role in this form of ischemia. Their findings were confirmed by others (Schöder et al. 2000). In the latter study, 17 patients with documented stable CAD and a comparable number of age-matched healthy control subjects underwent myocardial perfusion imaging with PET, using [13N]NH3. After acquiring images in rest, all participants were encouraged to relax during 45 min. Thereafter, mental stress was induced by asking each participant to perform arithmetic tasks. Every 2 min the tasks became more difficult, until a plateau in increased heart rate and blood pressure was established. At that time a second scan was performed. In both patients and healthy controls, the increase in rate-pressure product was similar (2,760 ± 794 vs 2,391 ± 1,196), as was the increase in serum epinephrine and norepinephrine blood levels. However, the increase in myocardial blood flow during mental stress was less in patients with known CAD than in healthy controls (14 ± 17 % vs 29 ± 14 %, p = 0.01).
Finally, other cofactors contributing to the relationship between mental stress and myocardial ischemia are probably a misbalance in sympathetic and parasympathetic tone (leading to diminished heart rate variability), changes in platelet activation and high levels of cortisol (Serrano et al. 2011).
22.5.3 Imaging of the Cardioneural Axis
Making use of [15O]-labelled water as a tracer for PET, regional cerebral blood flow changes were studied in 12 patients with significant coronary artery disease, following dobutamine-induced angina, for the first time about two decades ago (Rosen et al. 1994). Since cortical activation is required for the awareness of pain, the investigators proposed that the changes in blood flow in the lower brain areas represent pathways for perception of angina. Furthermore, the authors suggest that with H2[15O] labelling, PET research can be executed to study inconsistencies in visceral pain perception, such as during silent myocardial ischemia and cardiac syndrome X. And indeed this group and others performed PET studies in patients with cardiac syndrome X and showed that chest pain and ECG changes, not accompanied with left ventricular dysfunction on echocardiography; however, they reported changes in neural processing of afferent signals, specifically in the right insula (Rosen et al. 2002). In addition, in patients with cardiac syndrome X, high trait anxiety was shown to be associated with an increase in myocardial ischemia on myocardial perfusion imaging (Vermeltfoort et al. 2009).
In another study cortical evoked potentials were recorded, following peripheral stimulation in 16 patients with cardiac syndrome X, and the outcomes were compared to matched controls with and without coronary artery disease. A reduced habituation to repetitive noxious stimuli was observed in the patients with cardiac syndrome X, which reduction is thought to be responsible for the altered clinical characteristics of angina in these patients (Valeriani et al. 2005). Electrical neurostimulation has been demonstrated to normalize this disturbed abnormal cortical processing of pain (Sestito et al. 2008). Using PET and [15O]-labelled water, we reported on regional cerebral flow changes following electrical neuromodulation (Hautvast et al. 1997). Our findings corroborate the earlier study by Rosen et al. in identifying corresponding areas in the brain associated with cardiovascular control as Rosen et al. reported, following active electrical neuromodulation. Finally, to study the neurocardiac axis at the cardiac level and unravel the underlying mechanism of electrical neuromodulation, we have performed a PET study comparing active electrical neuromodulation with PET tracings after withholding neurostimulation. We found a more homogeneous distribution of perfusion, following active neuromodulation (Hautvast et al. 1996).
22.6 Conclusions
As mentioned previously, anxiety should be distinguished from emotional disorders. It is known that different forms of mental disorders, especially panic disorder and PTSD, are associated with a worse outcome of comorbid CAD. In subjects with a general anxiety disorder, this association was not found. Furthermore, mental and emotional stress can provoke myocardial ischemia and myocardial infarction. The role of heart rate variability is not yet clear but may not be as important as thought before. Finally, PET has been found to be a very relevant and reliable tool in the assessment of both, unravelling and understanding the underlying mechanism in the field of neurocardiology.
22.7 Future Perspectives
In the last three decades, many studies have been performed concerning mental stress-induced ischemia. Especially anxiety and depressive disorders seem to be associated with activation of cardioneural sympathetic pathways, resulting in myocardial ischemia, in patients with and without known CAD. Furthermore, the approach of these patients is different from those with conventional inducible ischemia. Still the exact pathophysiology needs further investigations.
The introduction of hybrid camera systems combining PET with magnetic resonance imaging (MRI) provides the clinician with additional information on perfusion and functional parameters of the heart (Slart et al. 2012). PET-MRI is a powerful tool for the evaluation of regional changes in perfusion and contractility after myocardial infarction. However, the combined use of these modalities in patients with mental stress-induced myocardial ischemia has not been reported. PET-MRI of the heart may be able to provide additional insight in its pathophysiology.
A novel field of interest concerns the use of SPECT and PET tracers for cardiac sympathetic and parasympathetic innervation imaging. Especially iodine-123-labelled metaiodobenzylguanidine ([123I]-MIBG) and carbon-11-labelled meta-hydroxyephedrine ([11C]-mHED) are established tracers for imaging cardiac sympathetic innervation. These analogues of norepinephrine bind to adrenergic receptors. Myocardial [123I]-MIBG uptake is diminished in patients with autonomic failure. For example, [123I]-MIBG can be used to discriminate idiopathic Parkinson’s disease from multisystem atrophy. Also in patients with Lewy body disease (LBD), myocardial [123I]-MIBG uptake is low (Suzuki et al. 2006). Therefore, the presence of cardiac sympathetic innervation abnormalities is able to distinguish LBD from fronto-temporal dementia (Novellino et al. 2010). However, [123I]-MIBG uptake levels in patients with LBD were not associated with a clinical profile of depression and anxiety (Kobayashi et al. 2010). This would still be an interesting field to explore, despite the fact that sympathetic tone appeared not to change in patients with stable CAD with and without concurrent diabetes (Fricke et al. 2007, 2008).
Finally, the therapeutic options in patients suffering from conventional myocardial ischemia are well established. However, the effect of calcium-channel blockers and ß-blockers in patients with mental stress-induced myocardial ischemia is limited (Andersson et al. 2011; Bairey et al. 1991). Therefore, future treatments may focus on behavioural intervention, either with or without antidepressant drugs such as dual-action antidepressant (e.g. mirtazapine). These interventions may improve outcome after myocardial infarction, possibly by reducing the stress-related induction of myocardial ischemia. However, major trials thus far consistently suggest no outcome benefits from depression treatment. Future studies should focus more in depth on monitoring treatment response, for example, in a subpopulation of patients that experience reductions in stress-induced myocardial ischemia. Furthermore, little is known about receptor expression and ligand binding during mental stress-induced myocardial ischemia and after successful treatment of this ischemic response. Also little information is available on the biological variability based on genetic polymorphisms. Myocardial perfusion imaging with SPECT or PET has the potential to develop into a helpful tool in treatment monitoring and may differentiate responders from non-responders.
References
Ahmadi N, Hajsadeghi F, Mirshkarlo HB et al (2011) Post-traumatic stress disorder, coronary atherosclerosis, and mortality. Am J Cardiol 108:29–33PubMedCrossRef
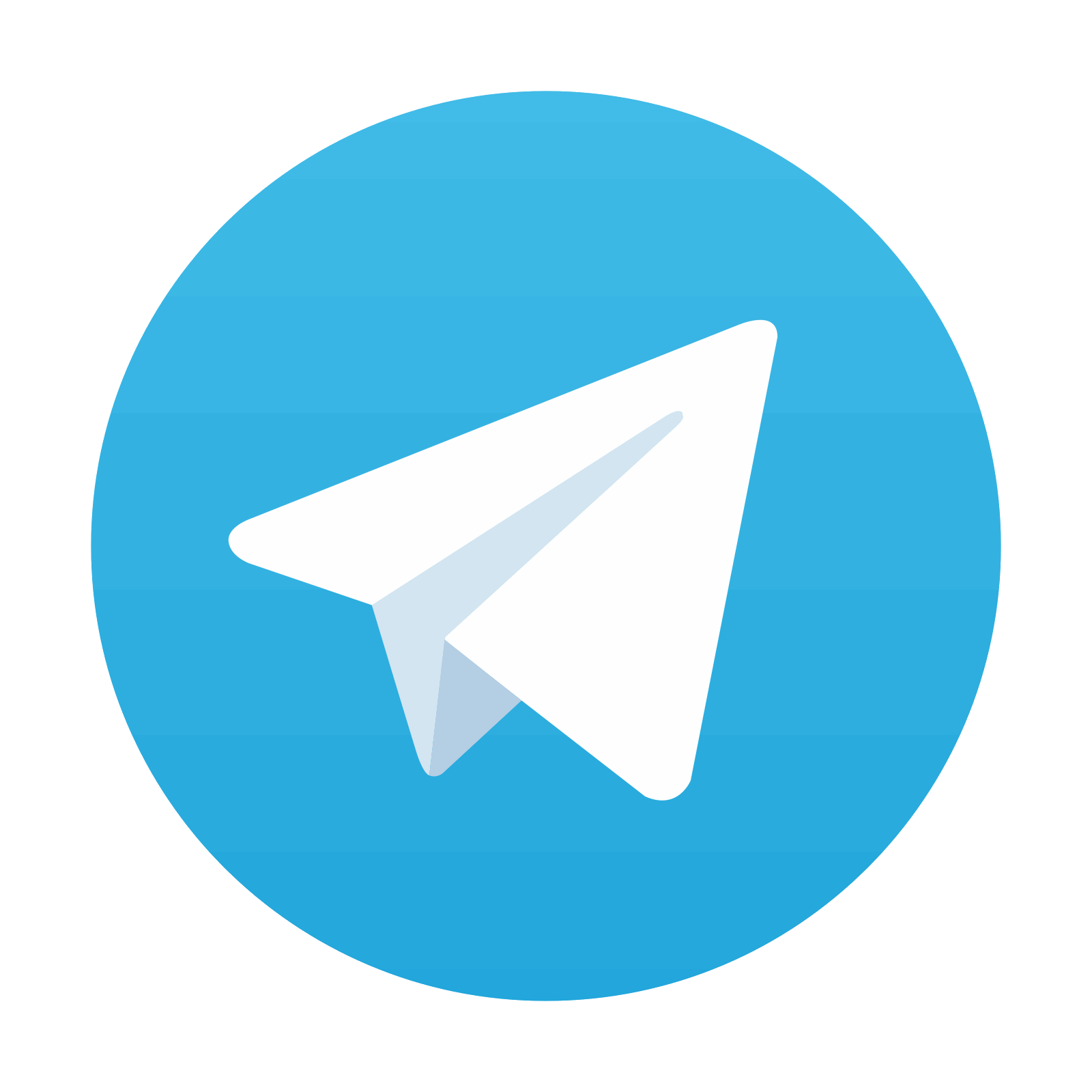
Stay updated, free articles. Join our Telegram channel

Full access? Get Clinical Tree
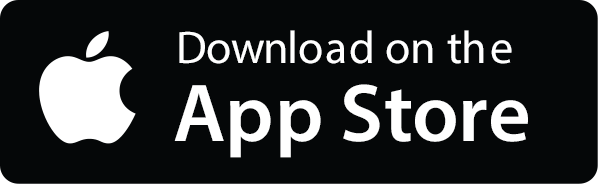
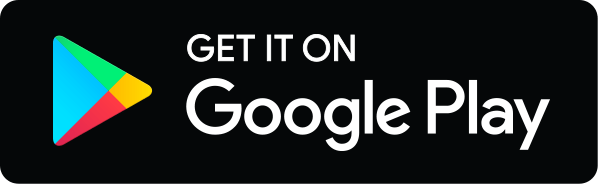