Fig. 16.1
An example of an abdominal subcutaneous fat aspirate exposing amyloid deposits, stained with Congo red. (a) Viewed in normal light: amyloid is stained red. Bar length is 200 μm. (b) Viewed in polarised light: amyloid shows apple-green birefringence (collagen is bluish white)
The prognosis of cardiac amyloidosis is worse compared to other manifestations of the disease. Cardiac AL amyloidosis is often rapidly progressive, and, in patients with ventricular septum thickness >15 mm, left ventricular ejection fraction (LVEF) <40 % and symptoms of heart failure, the median survival is less than 6 months (Ronsyn et al. 2011). No specific treatment exists for cardiac amyloidosis or restrictive cardiomyopathy. However, heart failure should be treated with diuretics, and cardiac transplantation might be considered in selected cases. Early detection of cardiac involvement is essential as the presence and severity of cardiac amyloidosis clearly influence the treatment options to stop progression of the disease and, even more importantly, directly affect prognosis.
16.1.1 The Role of Non-scintigraphic Imaging Modalities
A detailed outline on the role of non-scintigraphic imaging modalities to assess potential cardiac amyloidosis is not in the scope of this book chapter. In short, transthoracic echocardiography and cardiac magnetic resonance imaging with gadolinium enhancement are discussed.
Transthoracic echocardiography plays an important role in the evaluation of cardiac manifestation of amyloidosis. Nowadays, it is the modality of choice for the evaluation of amyloid deposition in the heart (Falk et al. 1987). The most common finding is left ventricular wall thickening due to amyloid deposition in the myocardium. This is often associated with right ventricular wall thickening, diffuse valvular infiltration, dilated atria and pericardial effusion (Klein et al. 1990). Although echocardiography plays a major role, the diagnosis of cardiac amyloidosis is often only possible when the disease has reached an advanced stage, where irreversible functional and structural myocardial changes have occurred. There is an obvious need for methods that detect cardiac amyloidosis in an earlier and preferentially presymptomatic phase.
If echocardiography is inconclusive, cardiac magnetic resonance imaging with gadolinium enhancement might be useful. Gadolinium is an extracellular fluid tracer which accumulates in expanded interstitial place. Usually, in the intact myocardium, the distribution of gadolinium is very low, and therefore gadolinium enhancement is absent. However, in the case of myocardial interstitial space expansion, such as in amyloidosis due to extracellular amyloid infiltration, gadolinium concentration may increase within myocardial tissue. Global subendocardial late gadolinium enhancement can be found in approximately two-thirds of patients with systemic amyloidosis (Maceira et al. 2005). However, the value of MRI for the early detection of cardiac involvement is not clear.
16.1.2 The Role of Scintigraphic Imaging Modalities
Scintigraphy, using labelled serum amyloid P component (SAP), provides not only information on different organ distributions, but serial scans can provide evidence of progression and regression of the disease (Hawkins 2002). Unfortunately, these SAP scans are unsuitable for detecting amyloid deposition in the myocardium, due to size of the tracer, heart movement, blood-pool content and residual tracer uptake in the spleen.
Autonomic innervation abnormalities resulting in impaired gastric emptying is fairly common in patients with hereditary ATTR amyloidosis, in which disease a TTR mutation causes amyloid deposition (Wixner et al. 2012). Scintigraphic gastric emptying studies can play a role in identifying gastric retention due to ATTR amyloidosis. This same modality had been used to evaluate the effect of liver transplantation on gastric emptying. It showed that liver transplantation eventually had no effect on the gastric emptying time in these patients (Suhr et al. 2003).
Myocardial adrenergic denervation, using iodine-123 meta-iodobenzylguanidine ([123I]-MIBG), has been shown present in patients with amyloidosis (Nakata et al. 1995; Tanaka et al. 1997; Delahaye et al. 1999). Indirectly, [123I]-MIBG visualises the effect of amyloid deposition in the myocardium. This technique might be able to detect early cardiac denervation before ongoing deposition of amyloid leads to actual heart failure. Table 16.1 provides an overview of the diagnostic criteria for the assessment of cardiac amyloidosis, using the above-mentioned imaging modalities. The purpose of this book chapter is to discuss the role of [123I]-MIBG in the assessment of cardiac amyloidosis.
Table 16.1
Diagnostic criteria for cardiac amyloidosis
Imaging modality | Findings in cardiac amyloidosis | Remarks |
---|---|---|
Endomyocardial biopsy | Positive Congo red staining | Gold standard, however invasive method |
Transthoracic echocardiography | Main findings: | Modality of choice, however often only positive in advanced stage of disease |
Left ventricular wall thickening | ||
Highly refractile (sparkling cardiac echoes) | ||
Associated findings: | ||
Right ventricular wall thickening | ||
Diffuse valvular infiltration | ||
Dilated atria | ||
Pericardial effusion | ||
Magnetic resonance imaging | Global subendocardial late gadolinium enhancement | Aspecific finding. No role in early stage of disease |
[123I]-MIBG scintigraphy | Planar views: | Restricted to AL and ATTR patients. Positive test results before abnormalities on echocardiography |
Low heart-to-mediastinum ratio (HMR, <1.6) at 4 h post injection | However, inferior wall defect on SPECT can be false positive | |
High wash-out rate (>20 %) at 4 h post injection | ||
SPECT: | ||
Segmental defect on polar map view |
16.2 Consequences of Impaired Sympathetic Innervation in Cardiac Amyloidosis
Cardiac amyloidosis is a form of restrictive cardiomyopathy, due to the progressive deposition of amyloid fibrils in myocardium and direct depression of diastolic function. Usually this occurs in both left and right ventricles, causing biventricular heart failure. But cardiac amyloidosis often presents itself as severe right-sided heart failure only. Eventually, systolic dysfunction leads to congestive heart failure. This occurs only in late stages because the left ventricular ejection fraction (LVEF) remains (nearly) normal until late in disease. Symptoms caused by heart failure are dyspnoea, orthopnoea, peripheral oedema and sometimes, in late stages of the disease, ascites.
Amyloid deposition in the atria can cause atrial fibrillation (AF) that causes complaints of fast and irregular heart action. Also, AF is associated with the development of thromboembolism. A poor LVEF and amyloid infiltration can contribute to the complications of embolisms (e.g. cerebral infarction).
Furthermore, microvascular disease does not only cause complaints of angina due to myocardial ischemia (Mueller et al. 2000), it also often leads to syncope (Chamarthi et al. 1997). The development of syncope seems to be based on multiple factors. First, it may be a consequence of bradycardia due to amyloid infiltration in the conduction system. Secondly, a syncope can be a result of sustained ventricular tachycardia. Third, it may be caused by hypotension due to autonomic neuropathy or forward failure, sometimes aggravated by overuse of diuretic drugs. Finally, it may be the onset of sudden cardiac death due to electromechanical dissociation rather than ventricular dysrhythmias (Falk et al. 1984).
16.3 Use of Planar Images of [123I]-MIBG in Amyloidosis
The use of [123I]-MIBG is studied most intensively in patients with hereditary ATTR amyloidosis with polyneuropathy, formerly called familial amyloidotic polyneuropathy. The first reported case of severe peripheral neuropathy due to hereditary ATTR, in which 111 MBq [123I]-MIBG was used, did not show any definite myocardial activity in all cardiac regions on either early (30 min post injection (pi)) or late images (4 h pi) (Nakata et al. 1995); see also an example of a normal and abnormal [123I]-MIBG scan in Figs. 16.2 and 16.3. Although the cardiac walls were markedly thickened by amyloid, the left ventricular ejection fraction was normal on radionuclide ventriculography, as well as myocardial perfusion using thallium-201 ([201Tl]). Analysis of heart rate variability (HRV) suggested highly damaged vagal and sympathetic activities. Thus, the defects on the [123I]-MIBG scan were considered to represent impaired sympathetic nerve endings in the heart due to amyloid deposition.
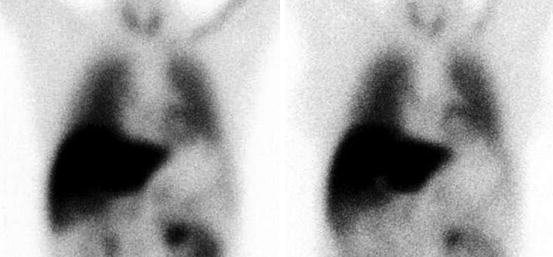
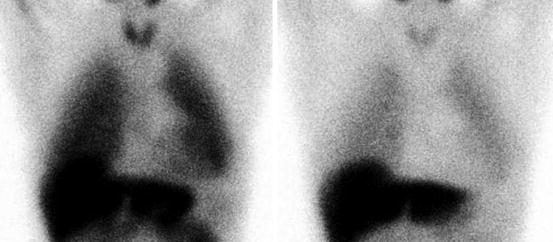
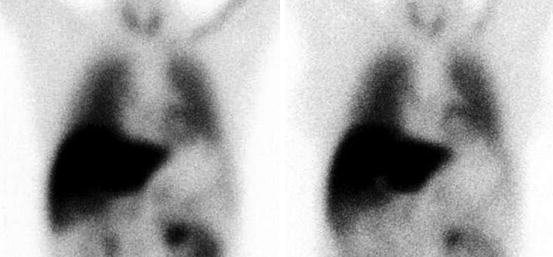
Fig. 16.2
An example of normal [123I]-MIBG uptake. HMR on early (left) image 2.50, HMR on late (right) image 2.50, wash-out 0 %
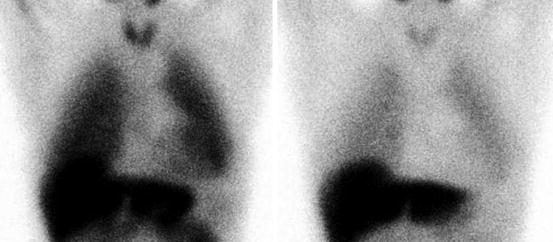
Fig. 16.3
An example of abnormal [123I]-MIBG uptake in a patient with amyloid deposition. HMR on early (left) image 1.89, HMR on late (right) image 1.37, wash-out 27 %
The lack of [123I]-MIBG uptake in myocardial tissue was also seen in a second case report (Arbab et al. 1997). In this patient with hereditary ATTR, a [123I]-MIBG scan was performed, which showed no uptake in the heart, indicating severe impairment of cardiac sympathetic function. Also several other investigations were performed, including technetium-99m-labelled dimercaptosuccinic acid ([99mTc]-DMSA), [201Tl] and iodine-123-labelled 15-(p-iodophenyl)-3-(R,S)-methyl-pentadecanoic acid ([123I]-BMIPP) studies. These studies showed myocardial involvement of amyloidosis ([99mTc]-DMSA), normal myocardial perfusion ([201Tl]) and normal fatty acid metabolism ([123I]-BMIPP), respectively.
In the first clinical study, 12 patients with hereditary ATTR and polyneuropathy were prospectively followed, using [123I]-MIBG and comparing it to echocardiography, and to [201Tl] and [99mTc]-labelled pyrophosphate ([99mTc]-PYP) imaging studies (Tanaka et al. 1997). All 12 patients suffered from biopsy-proven cardiac amyloidosis. Four mCi (148 MBq) [123I]-MIBG was administered and scans were performed 30 min and 3 h post injection (pi). In 8 out of these 12 patients no myocardial uptake of [123I]-MIBG was found on either the early or the late images. The remaining four patients showed only limited uptake in the anterior wall on both early and late images. Four patients had left ventricle (LV) wall thickening on echocardiography, with otherwise normal results. There was no significant correlation found between the prevalence of decreased uptake of [123I]-MIBG and LV wall thickness and results of [99mTc]-PYP scans. All 12 patients had normal myocardial perfusion on [201Tl] scan. So, in conclusion, patients with hereditary ATTR amyloidosis and polyneuropathy were found to have a high incidence of myocardial adrenergic denervation with viable myocardium, which can be found early in cardiac amyloidosis in the absence of clinically apparent heart disease.
In the second clinical study, 17 patients with hereditary ATTR amyloidosis and polyneuropathy were analysed before liver transplantation (Delahaye et al. 1999). All patients had biopsy-proven ATTR amyloid by specimens from either rectal mucosa or peripheral nerves. These patients underwent [123I]-MIBG (300 MBq) scanning at 20 min and 4 h pi, heart rate variability analysis, coronary angiography, radionuclide ventriculography, rest [201Tl] scan, echocardiography and measurement of plasma catecholamine levels. [123I]-MIBG scans were also performed in 12 age-matched control subjects. Planar [123I]-MIBG images were analysed using HMR and wash-out rate, defined as percent change in activity from early to late images within the LV. No patients showed evidence of coronary artery disease, perfusion defects or diminished LVEF. However, cardiac [123I]-MIBG uptake was dramatically decreased in ATTR patients compared to the age-matched control population, on both early and late images (HMR at 4 h: 1.36 ± 0.26 vs. 1.98 ± 0.35, p < 0.001). The wash-out rate was not significantly different. On the other hand, cardiac [123I]-MIBG uptake at 4 h correlated with the severity of polyneuropathy. In concordance to the results of the former mentioned study, these patients with ATTR amyloidosis had sympathetic denervation as assessed by [123I]-MIBG imaging, despite normal LV systolic function and myocardial perfusion.
In continuation of these findings a subsequent study in 31 patients with hereditary ATTR amyloidosis and polyneuropathy was performed after liver transplantation (Delahaye et al. 2006). The purpose of this study was to evaluate the outcomes of cardiac sympathetic innervation and amyloid infiltration after liver transplantation. Cardiac sympathetic innervation was assessed in the same manner as the study published in 1999 by the same authors: 300 MBq [123I]-MIBG, scans at 20 min and 4 h pi and the use of HMR and wash-out rates. A similar age-matched control population was used for normal values of HMR and wash-out rate. All patients also underwent a [201Tl] scan at rest, heart rate variability analysis, echocardiography, and right heart catheterisation. Sympathetic denervation was found in patients before liver transplantation compared to the control population (HMR 1.45 ± 0.29 vs. 1.98 ± 0.35, p < 0.001) After liver transplantation, there was no significant change in global [123I]-MIBG HMR (1.46 ± 0.28). This may implicate that progression of cardiac sympathetic denervation stops after liver transplantation and that early re-innervation cannot be measured within 2 years after liver transplantation. There was no correlation found between age and echocardiographic findings. However, conduction disturbances, ventricular arrhythmias and LV wall thickening were associated with low [123I]-MIBG uptake and progressed after liver transplantation. This may implicate progression of cardiac amyloid infiltration after liver transplantation (Haagsma et al. 2007). Low cardiac [123I]-MIBG uptake was also in this study associated with severity of polyneuropathy, which worsened after liver transplantation. The authors conclude that [123I]-MIBG imaging can provide an objective measurement of cardiac sympathetic innervation, which could help to guide the indications for liver transplantation in patients with early stage of hereditary ATTR amyloidosis and polyneuropathy.
Although symptoms and consequences of cardiac amyloid deposition in AL amyloidosis are often more frequent and severe than in ATTR amyloidosis (causing more frequently fatal dysfunction), the use of [123I]-MIBG in this type of disease has hardly been studied. In fact only one major study has been performed in which the presence of impaired myocardial sympathetic innervation was related to clinical autonomic abnormalities and congestive heart failure in AL amyloidosis (Hongo et al. 2002). In this study 25 patients with biopsy-proven cardiac manifestation of AL amyloidosis underwent autonomic function tests, echocardiography, heart rate variability analysis and [123I]-MIBG scanning. The [123I]-MIBG scans were performed using 111 MBq [123I]-MIBG with uptake at 30 min and 3 h pi. Myocardial uptake and wash-out rates were calculated using HMR. Furthermore, 20 of 25 patients underwent [201Tl] scan at rest for myocardial perfusion. Of the 25 patients, 9 suffered from autonomic dysfunction and 16 did not. Five of 9 patients with autonomic dysfunction and 10 of 16 without had congestive heart failure. Between the two groups with and without autonomic dysfunction, no significant difference was found in amyloid deposition in the right and left ventricular wall based on echocardiographic thickness. None of the patients had myocardial perfusion defects. In patients with autonomic dysfunction, HMR (1.37 ± 0.05 vs. 1.66 ± 0.09 after 30 min, p < 0.001, and 1.29 ± 0.05 vs. 1.53 ± 0.06 after 3 h, p < 0.001) and wash-out rates (30.8 ± 4.0 % vs. 41.5 ± 4.8 %) were significantly decreased compared to the patients without autonomic dysfunction. In both groups, HMR was significantly decreased and wash-out rate increased in patients with heart failure (10 of 16 without autonomic dysfunction, 5 of 9 with autonomic symptoms) compared to the patients without heart failure. Therefore, myocardial uptake and turnover of [123I]-MIBG in patients with AL amyloidosis are heterogeneous and seem to depend on the presence of both congestive heart failure and cardiac autonomic dysfunction.
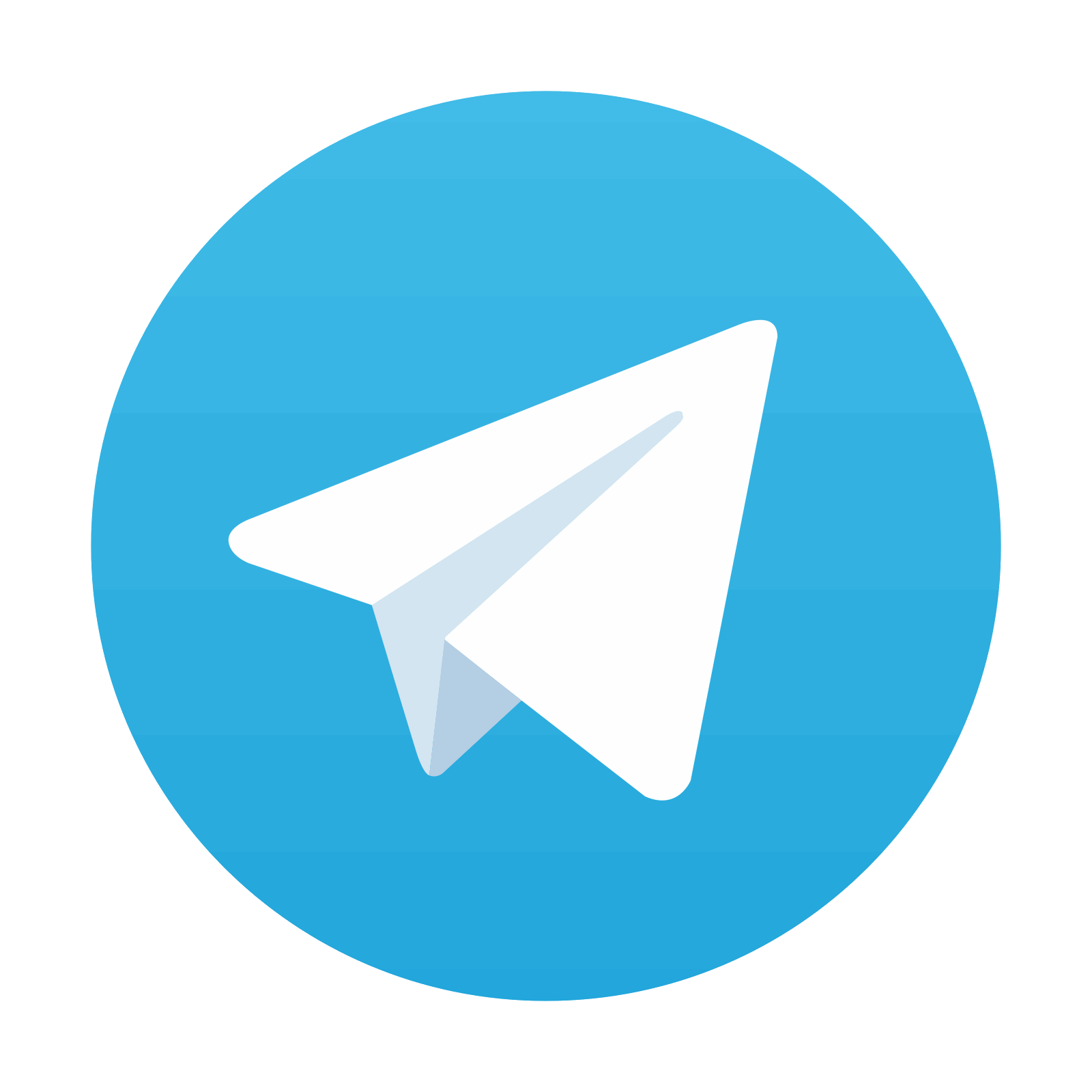
Stay updated, free articles. Join our Telegram channel

Full access? Get Clinical Tree
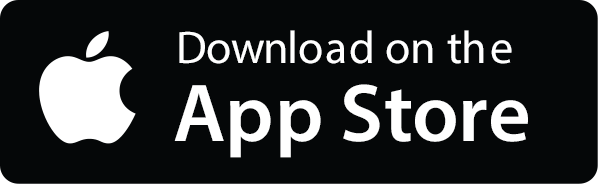
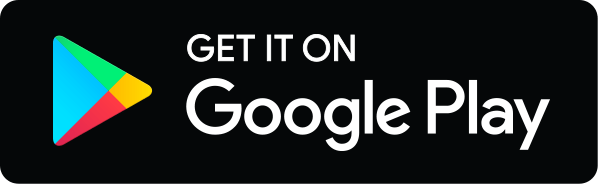