Fig. 6.1
a Segmentation of a 1-day-old infant prenatally diagnosed with complex DORV, uncommitted VSD, d-malposed great vessels, and coarctation. CT was obtained with 0.2 mSv radiation dose. b 3D digital rendering and printed cardiac model (performed using Mimics software, Materialise NV, Plymouth, MI)
Aside from radiation, other unique considerations, both risks and benefits, are involved in performing cardiac CTs on pediatric patients. A major benefit of the increased temporal resolution of cardiac CT is that the need for sedation is minimized. Children over the age of 3 years can usually remain still for the brief time needed for CT acquisition. Breath-holding may not be required for volumetric or high-pitch scanners, though it is still needed for imaging acquired over several heartbeats or coronary artery imaging [39, 43]. At our institution, for 3D printing purposes, we typically administer at least moderate sedation to patients under 3 years to avoid blurring from motion and minimize stair-step artifact on our step-and-shoot CT scanner. In neonates, in whom anatomic structures are small and spatial resolution is important, we will consider intubation, paralysis, and suspending ventilation to acquire very high-quality datasets for prototyping. Often, neonates facing the prospect of complex surgical repair requiring 3D modeling are critically ill and may be already intubated in the neonatal intensive care unit.
As previously mentioned, cardiac CT does not require the use of gadolinium, but does require intravenous injection of iodinated contrast at doses of about 1–2 mL/kg. Though not associated with neurotoxicity, CT contrast can induce nephropathy in patients with decreased renal function. This is often a concern in children with CHD and pediatric patients who have undergone a heart transplant. Patients may benefit from pre-hydration and treatment with protective agents like n-acetyl cysteine [44, 45]. Contrast extravasations are rare, even when using a power injector in a 24-gauge IV in a neonate [46]. Risk of iodinated contrast allergy is higher than that of gadolinium-based agents but is typically less than 0.5% in children [47, 48]. In patients with aortopulmonary shunts or a Fontan palliation, it is particularly important to tailor contrast injection protocols to a patient’s specific anatomy and circulation pathways. When imaging a patient after Fontan palliation, some groups suggest simultaneous contrast administration through upper and lower extremity intravenous lines to opacify the entire pathway, though delaying imaging after contrast injection may also achieve the same effect [49, 50].
Other benefits of cardiac CT include its ability to simultaneously image extracardiac structures such as the airway and lung parenchyma with high-spatial resolution. It is also ideal for patients with metallic devices such as pacemakers or stents that are either contraindicated in MRI or may cause significant artifact. CT is optimal for critically ill patients, who may not be stable enough to undergo long MRI examinations [37]. The relatively high heart rates of pediatric patients are not a significant limitation to obtaining high-quality CT images unless coronary imaging is a critical component of a 3D model. If coronary imaging is needed, beta blockers or calcium channel blockers may be administered when indicated in order to reduce the heart rate to less than 70, and ideally less than 60, beats per minute to achieve adequate image quality. Our group recently reported that using an intravenous phenylephrine infusion in conjunction with these medications can further decrease heart rates even in young children by supporting blood pressure as well as causing reflex bradycardia [51]. Such low heart rates may not be safely achievable in very young patients, however [39].
3D Echocardiography
Regardless of the increasing popularity of cardiac MRI and CT, echocardiography remains the first-line bedside imaging modality in children with CHD. It is less resource-intensive, typically does not require sedation, and does not involve exposure to ionizing radiation or intravenous contrast agents. Recent advances in 3D echocardiography have improved our ability to obtain 3D echo images in young children and have made it increasingly possible to obtain volumetric DICOM datasets that can be used for rapid prototyping.
While 3D echo technology has been in use for several decades, more advanced real-time 3D echo systems have become commercially available in recent years, and smaller, high-frequency 3D echo probes that can be used in pediatric patients have been introduced [52]. 3D transesophageal echocardiography has also been introduced; however, the large size of the 3D TEE probe generally limits its use to children weighing at least 20 kg [53]. 3D echo has been shown to be particularly useful for delineating atrioventricular valve morphology and function, details of atrial and ventricular septal defects, and complex intracardiac relationships such as the ones often highlighted by 3D printing [54].
Obtaining high-quality 3D echocardiographic datasets is more operator-dependent than cardiac MRI or CT and can present some challenges in the pediatric population. Movement artifact can pose an issue in young children, and sedation may be required in some patients. Highspatial resolution and frame rates are also needed, which can be difficult to achieve in children with small cardiac structures and high heart rates [54]. Using live 3D imaging can overcome this limitation by providing true real-time imaging, though it limits the size of the field of view that can be acquired. Alternatively, a full volume acquisition can be obtained that provides a considerably larger imaging field but requires imaging over multiple heartbeats and may therefore results in “stitch artifact” from combining information from multiple cardiac cycles [55].
Olivieri and colleagues recently reported successfully 3D printing accurate cardiac models of patients with ventricular septal defect and periprosthetic aortic valve leaks based on 3D echocardiographic datasets [56]. The group noted that image acquisition was optimized by using a high-frequency 3D transducer, minimizing image depth, acquiring images only in diastole, and minimizing image gains to decrease blood pool noise. They also applied noise reduction filters to the imaging data in order to further minimize the blood pool and emphasize anatomic details prior to segmentation. This technique produced high-quality, highly accurate defect depictions (Fig. 6.2). However, as the authors noted, their methodology may not be as applicable to segmenting 3D echo images of valves and other cardiac structures involving tissue rather than spaces created by defects.


Fig. 6.2
Segmentation of 3D echocardiogram depicting a membranous ventricular septal defect; reproduced with permission of the publisher from J Am Soc Echocardiogr. 2015 Apr;28(4):392–7
Which Imaging Modality Should Be Used?
As technologic advances provide us with more options for sourcing 3D datasets for rapid cardiac prototyping, it can be difficult to choose the most appropriate option for one’s patient. Careful consideration must be made in order to choose the optimal study for the patient and perform it optimally. Patient age and size, developmental stage, clinical indication, comorbidities, available technology, ensuring patient safety, and the overall goals of 3D printing must be taken into account. At our institution, for example, low-radiation cardiac CT is preferred to maximize spatial resolution in small infants and avoid the need for general anesthesia needed for MRI in young children. Table 6.1 summarizes some of the benefits and optimal uses of cardiac MRI, CT, and 3D echocardiography, respectively, in pediatric patients with CHD.
Table 6.1
Comparative benefits of cardiac imaging modalities used in children to create 3D heart models
Cardiac MRI | Cardiac CT | 3D Echocardiography |
---|---|---|
• Ideal for patients who do not require sedation and can tolerate a 1+ h study • Avoids radiation exposure • Provides additional ventricular function, volume, and/or flow data • CT contrast allergy • Poor echocardiography windows | • Submillimeter spatial resolution needed (e.g., in small infants) • Coronary artery imaging needed • Implanted metallic devices that may preclude or limit MRI imaging • Only anatomic imaging needed • Avoids general anesthesia (e.g., age <7 years, increased risk of anesthesia complications) • Poor echocardiography windows • Patient unable to fit in MRI due to morbid obesity or claustrophobia | • Rapid, easily accessible bedside imaging needed • Detailed cardiac valve imaging needed • Renal insufficiency, contrast allergy, or other contraindication to intravenous contrast agents • Avoids IV placement • Avoids sedation and radiation • Patient unable to fit in MRI due to morbid obesity or claustrophobia |
References
1.
2.
Ntsinjana HN, Hughes ML, Taylor AM. The role of cardiovascular magnetic resonance in pediatric congenital heart disease. J Cardiovasc Magn Reson. 2011;13:51.CrossRefPubMedPubMedCentral
3.
Krishnamurthy RCT. Principles and practice of cardiac magnetic resonance in congenital heart disease: form, function, and flow (Fogel MA, editor). Chichester, West Sussex, UK; Hoboken, NJ: Wiley-Blackwell; 2010. xii, 464 p.
4.
Fratz S, Chung T, Greil GF, Samyn MM, Taylor AM, Valsangiacomo Buechel ER, et al. Guidelines and protocols for cardiovascular magnetic resonance in children and adults with congenital heart disease: SCMR expert consensus group on congenital heart disease. J Cardiovasc Magn Reson. 2013;15:51.CrossRefPubMedPubMedCentral
5.
6.
Hartung MP, Grist TM, Francois CJ. Magnetic resonance angiography: current status and future directions. J Cardiovasc Magn Reson. 2011;13:19.CrossRefPubMedPubMedCentral
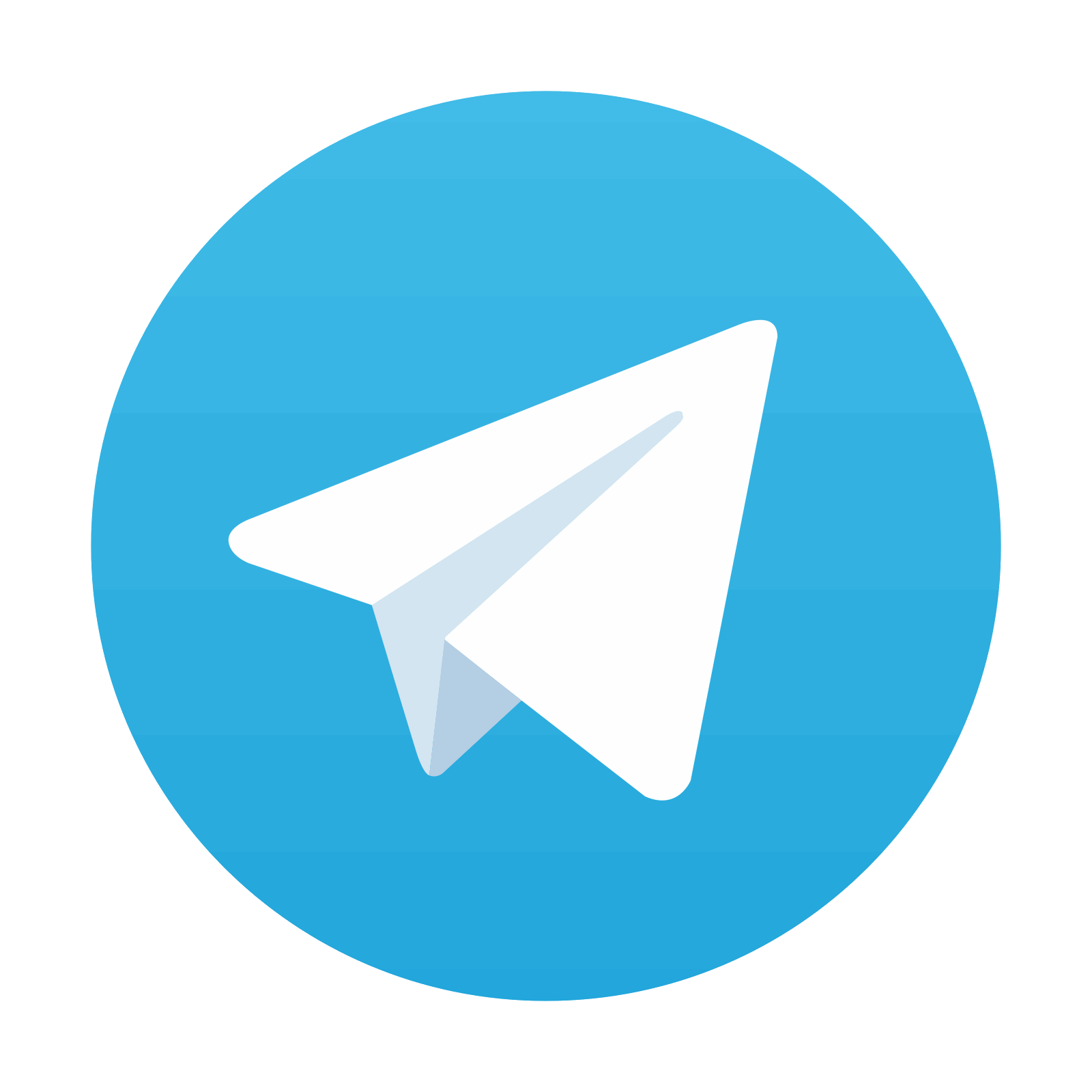
Stay updated, free articles. Join our Telegram channel

Full access? Get Clinical Tree
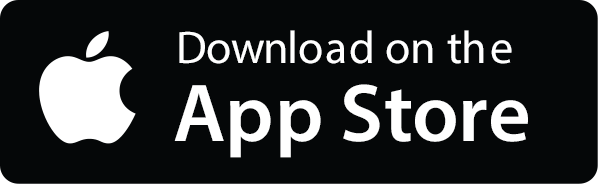
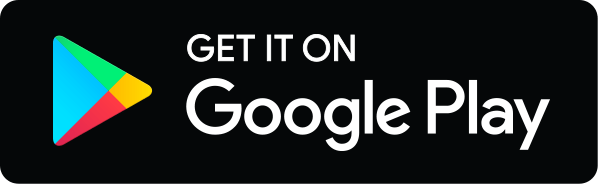