Fig. 4.1
Image types used for 3D modeling. a MRI 3D-SSFP. b MRA. c MRI 3D-IR. d CTA. e 3D echo
Modern digital imaging systems store images primarily in DICOM format. Regardless of modality, digital images are displayed as a matrix of pixels. Pixels are two-dimensional (2D) boxes that vary in brightness or attenuation based on the characteristics of the tissue they represent. The intensity of a particular tissue depends on the imaging modality used. For example, blood is bright on contrast-enhanced CT images, dark on echo images, and on MRI varies by the type of sequence used.
2D image slices are stacked in order to form a 3D volumetric dataset. 3D pixels are known as voxels. Isotropic voxels have equal or nearly equal size in the x, y, and z dimensions. The cubical nature of isotropic or near-isotropic voxels allows for the image to be reconstructed in any plane, including oblique views, without distortion.
Cardiac Imaging Modalities
Computed Tomography
Contemporary multidetector-row CT scanners acquire stacks of thin axial slices with isotropic resolution. CT angiography (CTA) employs intravenously administered contrast to improve visualization of blood-filled structures. CTA images obtained on modern high-pitch scanners allow for excellent visualization of cardiac tissue, vasculature, and extracardiac structures. CT has the advantage of high spatial and temporal resolution. Scan time is very short, resulting in increased availability and the ability to scan younger patients with minimal or no sedation. Two main disadvantages of CT are radiation exposure and the use of iodinated contrast, which has important nephrotoxic and allergic side effects [3].
Magnetic Resonance Imaging
Cardiac MRI examinations employ a wide variety of imaging sequences. Most sequences are obtained as bright-blood cine or black blood still images in specific cardiac planes. These images are typically non-isotropic and vary in thickness from 3 to 10 mm. However, high-resolution electrocardiogram (ECG)-gated 3D balanced steady-state free precession (3D-SSFP) and 3D inversion recovery (3D-IR) sequences can be obtained isotropically. Like CT, 3D-SSFP and 3D-IR datasets allow for high-resolution visualization of cardiac tissue and vasculature. Contrast-enhanced 3D magnetic resonance angiogram (MRA) sequences can also be reconstructed isotropically and tend to produce superior vascular imaging compared to 3D-SSFP and 3D-IR sequences. They do, however, suffer in delineation of intracardiac anatomy due to lack of ECG gating. Compared to CT, MRI does not employ ionizing radiation, not all sequences require contrast, and the contrast used has a lower rate of idiosyncratic reactions. MRI suffers from decreased spatial resolution compared to CT. Imaging takes longer and often requires sedation and general anesthesia in younger patients. Images are also susceptible to ferromagnetic and flow-related artifacts [4].
3D Echocardiography
Modern commercial echocardiographic equipment now routinely has the capability to obtain 3D volumetric datasets using both transthoracic and transesophageal probes. Images can be acquired in real time or can be stitched together over multiple heartbeats to increase temporal resolution and the size of the image volume. In general, US has high temporal and spatial resolution that allows for excellent visualization of intracardiac anatomy, particularly in pediatric patients. In particular, imaging of heart valves is superior, and imaging of extracardiac vascular structures inferior, to CT and MRI. US suffers from limitations due to poor acoustic windows, limited penetration, and a relatively small field of view. Compared to CT and MRI, US is low-cost, portable, widely available, does not require radiation or contrast, and does not always require sedation in young patients. [5–7]
Traditionally, images from a single modality have been used in preparing individual models. More recently, groups have begun to describe hybrid models making use of CT or MRI data for the bulk of intracardiac anatomy, combined US data focused on the heart valves [8].
Regardless of modality, images used for 3D modeling should be isotropic or near-isotropic and high-resolution. Accurate and efficient modeling is also dependent on uniform and clear image contrast. Thinner slices generally allow for higher spatial resolution and decreased partial volume effects; however, they may suffer from decreased signal-to-noise ratio and also take longer to process.
Basic Principles of Segmentation
In order to create a 3D model, image data to be used in the final model must be separated from extraneous data. This is achieved through the process of segmentation. Segmentation involves assigning labels to individual pixels within a DICOM image. Pixels representing a particular tissue (e.g., blood pool, myocardium) can be grouped together into masks, thus separating them from pixels representing tissue that is not of interest (e.g., bone, lung).
Successful segmentation relies on images with high spatial and contrast resolution. Contrast resolution is the ability to differentiate between different tissue types. Blood pool must be differentiated from cardiac tissues, and cardiac tissues from adjacent structures such as thymus, chest wall, lung, diaphragm, and liver. Image contrast differs between imaging modalities, and within modalities based on imaging technique and sequence. For example, CTA may yield higher blood–myocardium contrast than 3D-SSFP, yet 3D-SSFP may have higher myocardium–liver contrast. Individual CTA examinations may differ in myocardium–blood contrast due to differences in technical factors such as radiation dose, as well as timing, and density of contrast administration.
Several approaches to segmentation exist and vary based on the intended purpose of the model (Fig. 4.2). Vascular only models tend to be the easiest and most efficient to produce, as they only require segmentation of the vascular lumen. Segmentation here is simplified by the relatively high contrast between blood and extravascular tissue, particularly on CTA, 3D-SSFP, 3D-IR, and MRA sequences.


Fig. 4.2
Segmentation techniques. a Source image, axial CTA. b Myocardial segmentation. Note how with myocardial segmentation the border between the epicardium and extracardiac tissue is difficult to distinguish, particularly anteriorly and posteriorly. c Blood pool segmentation. d Errors in segmentation. Arrows point to unmasked areas of atrial and ventricular septum. Once modeled, these “bridges” will appear as septal defects
The generation of models depicting intracardiac anatomy poses a different set of challenges. Segmentation of cardiac tissue and myocardium is possible but can be difficult and time-consuming, primarily due to lack of contrast between myocardium and adjacent epicardial structures. This finding is true for both CT and MR images.
One commonly employed method to circumvent this problem is the “hollow shell” method, which relies on blood pool segmentation rather than myocardial segmentation (Fig. 4.3). This method is based on the principle that the outer contour of an intracardiac blood pool mask is the negative impression of the endocardial surface, much in the same way that a cast is an impression of the inner surface of the mold. If a shell (or “mold”) is created around the outer surface of the blood pool (“cast”), then the inner surface of the shell will replicate the endocardial surface. The blood pool mask is then removed to reveal this surface. It is important to note that because the thickness of the shell is applied arbitrarily and uniformly, it does not provide a true representation of the actual wall of the heart and the vessels. However, the general shape of the heart and vessel wall is maintained, and this creates an aesthetically pleasing representation of the surface of the heart.


Fig. 4.3
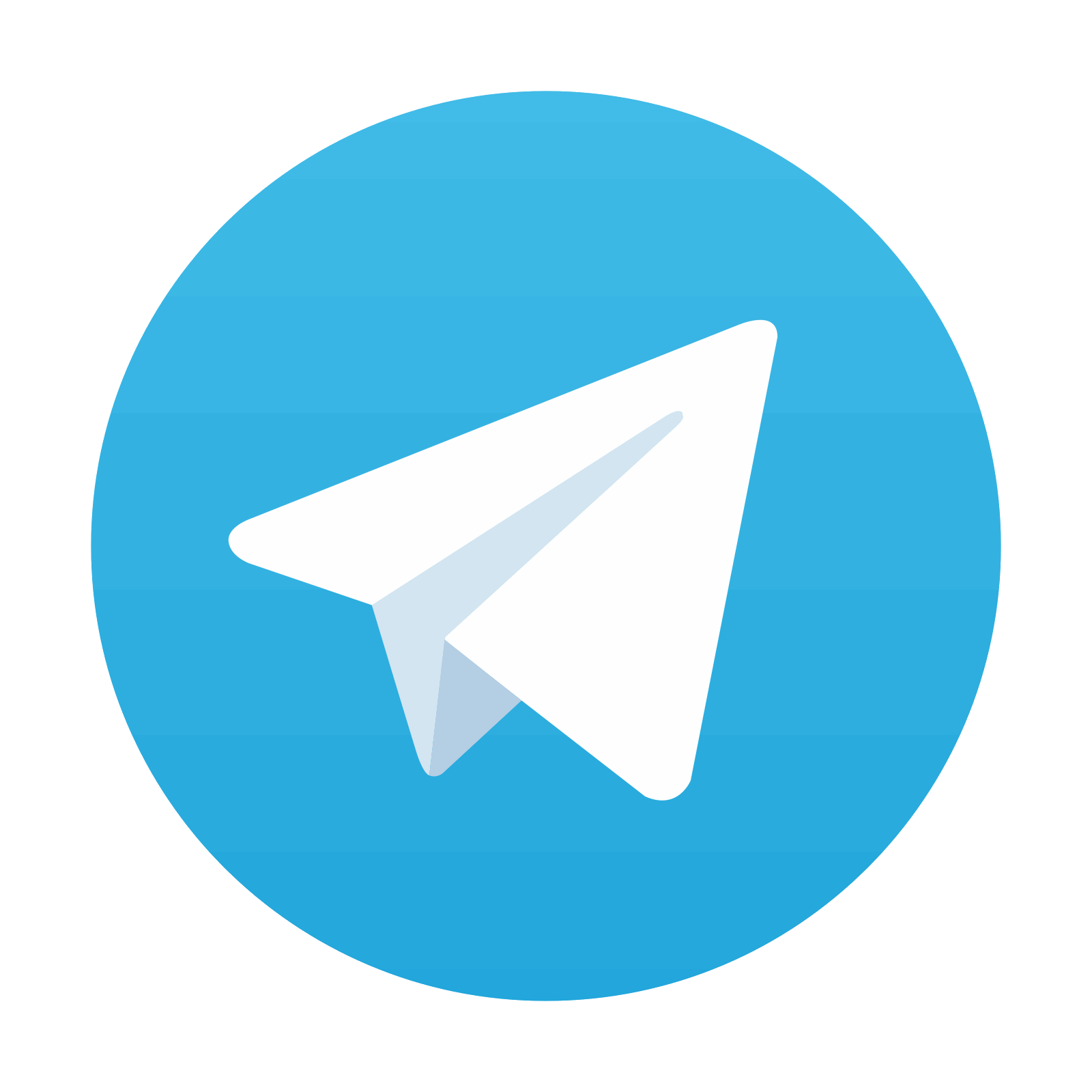
“Hollow shell” intracardiac segmentation method. a STL model of the blood pool mask in a patient with TGA and VSD. The blood pool is viewed from a posterior angle. b A shell of arbitrary thickness is applied to the outer surface of the blood pool. c The blood pool mask is removed. The inner surface of the shell represents the endocardial surface, note the VSD*. Also note that the exterior surface of the shell does not represent the true epicardial surface
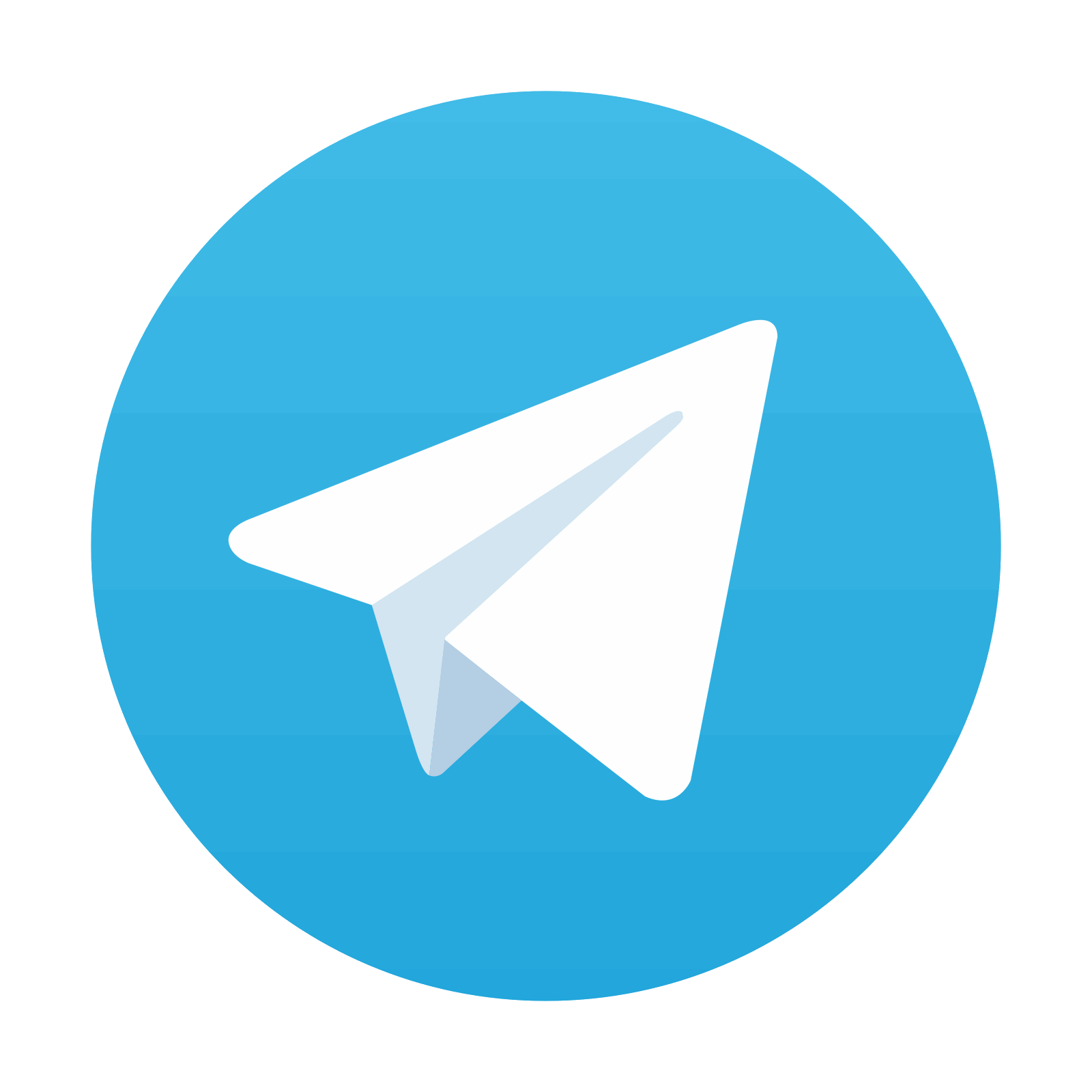
Stay updated, free articles. Join our Telegram channel

Full access? Get Clinical Tree
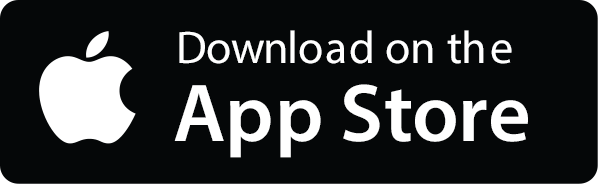
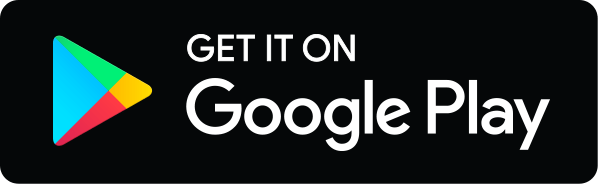
