Cardiac resynchronization therapy (CRT) has been shown to induce a spectacular effect on left ventricular (LV) function in certain patients. Our aim was to analyze and characterize the super-responders (SRs) to CRT using echocardiography in 186 patients with a conventional indication according to the European Society Cardiology guidelines. The investigation took place before and 6 months after implantation. CRT-SRs were defined by an improvement of the New York Heart Association functional class and LV ejection fraction to ≥50% in absolute values associated with a relative LV end-systolic volume reduction of ≥15%. Of the 186 patients, 18 (9.7%) were identified as CRT-SRs and had a significantly lower prevalence of ischemic etiology (11%), lower LV dimensions, lower left atrial volume, and greater global longitudinal strain at baseline. Receiver operating characteristics curves identified global longitudinal strain as the strongest parameter for predicting CRT-SRs, with a cutoff value of −12% (area under the curve 0.87, sensitivity 71%, and specificity 85%, p <0.01). In conclusion, in the present retrospective study, only a left atrial volume <55 ml and global longitudinal strain ≤−12% were independent predictors of CRT-SRs.
Cardiac resynchronization therapy (CRT) has significantly improved the symptoms and survival of patients with severe refractory heart failure, New York Heart Association functional class III or IV, prolonged QRS duration, and low left ventricular (LV) ejection fraction (EF). Randomized clinical trials have demonstrated that about 70% of the patients respond favorably to CRT with a reduction in New York Heart Association functional class and a significant effect on LV remodeling. The expected relative improvement of LV volumes and LVEF after CRT has ranged from 15% to 22%. However, CRT has been shown to dramatically improve the New York Heart Association functional class in certain patients, significantly affect the LVEF, and result in inverted remodeling with nearby normalization of LV contraction. These improvements were especially marked in patients with nonischemic cardiomyopathy presenting with a LV end-diastolic volume of <180 ml and LVEF of 30% to 35% before implantation. Left bundle branch block is considered a reversible cause of nonischemic dilated cardiomyopathy with severe heart failure and is also known as LV dyssynchrony-induced cardiomyopathy. Using echocardiographic analysis, including a LV 2-dimensional strain evaluation, the present retrospective multicenter study sought to identify and characterize CRT super-responders (CRT-SRs) at 6 months, defined as patients with an improvement in New York Heart Association functional class and absolute LVEF of ≥50%, associated with a relative reduction of LV end-systolic volume of ≥15%. Echocardiography was performed before and 6 months after implantation.
Methods
The study group consisted of patients with refractory heart failure, who had been referred for CRT to 2 cardiology centers (Bordeaux and Rennes, France), from September 2005 to July 2007. They fulfilled the criteria for CRT device implantation as defined by the European Society of Cardiology guidelines (LVEF of ≤35%, QRS duration ≥120 ms, and New York Heart Association functional class III or IV, despite optimal pharmacologic therapy), with clinical and echocardiographic data available 6 months after CRT. The exclusion criteria were potential reversible causes of cardiomyopathy, in particular, chronic alcoholic consumption, thyroid dysfunction, or suspected myocarditis and poor window quality.
CRT was provided in the standard fashion with the insertion of 3 transvenous leads. The right atrial and ventricular (apical site) leads were positioned in a conventional manner. Electrophysiologists placed the LV lead into the epicardial veins through the coronary sinus according to routine protocol; it was placed into the lateral or posterolateral vein in 80% of the cases. In the other cases, it was implanted into the anterolateral or middle cardiac vein. The position of the lead was determined by the coronary sinus angiographic data. After implantation, atrioventricular delay optimization was programmed individually to reach the optimal diastolic filling using the Doppler mitral inflow before discharge. For all patients, V-V timing was programmed to be simultaneous. All devices were systematically interrogated just before hospitalization discharge and within 6 months after the CRT procedure to ascertain their proper functioning.
Baseline echocardiography was performed for each patient before CRT implantation using Vivid 7 systems (GE-Vingmed, Horten, Norway). Digital routine gray-scale 2-dimensional, Doppler, and tissue Doppler imaging cine-loops from 3 consecutive cardiac cycles were obtained from the LV long-axis view, basal LV short-axis view, and apical views. Off-line analyses were performed blindly on digitally stored images (EchoPac, version BT08, GE-Vingmed) by an independent echocardiographic core center laboratory. The echocardiographic examination was conducted according to the American Society of Echocardiography guidelines. The LV end-diastolic and end-systolic diameters and volumes were quantified, and the LVEF was calculated using the biplane Simpson equation. The left atrial end-systolic volume was quantified using the biplane area–length method, and mitral valve regurgitation was quantified using the proximal isovelocity surface area method. LV diastolic function was evaluated by mitral inflow early-diastolic wave (E, cm/s), end-diastolic wave (A, cm/s), E deceleration time (ms), isovolumic relaxation time (ms), pulsed tissue Doppler imaging at the lateral annulus (peak E′ wave, cm/s), and mitral velocity propagation in color M-mode (Vp, cm/s). Combined criteria reflecting LV filling pressures (E/E′ and E/Vp) were calculated. The rate of pressure increase in systole (mm Hg/s), systolic pulmonary arterial pressure, and right ventricular function by peak S′ (cm/s) wave on pulsed tissue Doppler imaging at the lateral free wall were quantified.
Subsequently, conventional parameters of dyssynchrony were recorded using a multiparametric approach. The multiparametric approach consisted of cumulating the individual parameters of dyssynchrony (none, 1, 2, 3, or ≥4) to evaluate the probability level of a CRT response. Interventricular dyssynchrony was measured as the time difference between pulmonary and aortic pre-ejection delays >40 ms, and atrioventricular dyssynchrony was defined as the LV filling time/RR interval duration of <40%. Intra-LV dyssynchrony was measured. In the radial axis (parasternal view), the septal-to-posterior wall delay in M-mode of ≥130 ms and radial end-systolic inferolateral overlap in M-mode versus mitral valve opening were assessed. In the longitudinal axis (in the 3 apical views), the maximum difference between the time to onset on tissue Doppler imaging on the 6 basal segments (electromechanical delay) of ≥65 ms was measured, as was the maximum differences between the interval to peak on tissue Doppler imaging on the 6 basal segments (electrosystolic delay) of ≥65 ms, longitudinal end-systolic anterolateral overlap in M-mode versus mitral valve opening, and aortic pre-ejection delay of ≥140 ms.
An analysis of the 3 components of myocardial deformation was performed using 2-dimensional speckle tracking imaging. The speckles are natural acoustic markers equally distributed within the myocardium and can be detected and tracked on standard gray-scale 2-dimensional images. The images were analyzed off-line using Echopac PC software (GE, Horten, Norway). A minimum frame rate of 50 Hz was required (mean 65 ± 10 Hz). Myocardial strain was calculated by measuring the change of the speckles’ position within a myocardial segment along the cardiac cycle. The initial contour of the endocardial border was delineated manually at end-systole and was tracked automatically with the myocardial wall, frame by frame. The quality of the tracking could be verified by the operator, and the contour, as well as the region of interest, could be readjusted for optimal evaluation. Segmental and global circumferential and radial end-systolic strains were quantified using a parasternal mid-level short-axis view, and the longitudinal and transversal strains were obtained from the apical views.
The patients’ clinical status was evaluated at baseline and after 6 months. The 6-month echocardiogram included the LVEF, LV diameters, and LV volumes, rate of pressure increase in systole, and mitral regurgitation grade. To define CRT-SRs, previously described criteria were used, including improvement of the New York Heart Association functional class and absolute LVEF of ≥50% after 6 months and a relative reduction of LV end-systolic volume of ≥15%. Conventional responders to CRT (CRT-CR) were defined by a relative reduction of ≥15% of the LV end-systolic volume at 6 months and a LVEF of <50%. When the LV end-systolic volume reduction at 6 months was <15%, patients were considered to nonresponders to CRT.
Statistical Package for Social Sciences, version 11.5 (SPSS, Chicago, Illinois), was used for statistical evaluation. All group data are presented as the mean ± SD and were compared using the 2-way analysis of variance test. The Pearson chi-square test was used to compare percentages from independent series, and Fisher’s exact test was used to compare 2 percentages. Using the area under the receiver operating characteristic curve, the optimal cutoff value, sensitivity, and specificity were determined for each parameter. Univariate analysis was also performed to determine the odds ratios for CRT-SR. To identify the risk factors directly related to events, multivariate logistic regression analysis was conducted. A p value <0.05 was considered significant.
Results
The study population included 186 patients with heart failure (mean age 64.4 ± 12.1 years, 45 women, 92% with New York Heart Association class III, and 48% with ischemic etiology), meeting the inclusion criteria and with 6-month data available for study. The QRS duration was 157 ± 23 ms (range 120 to 195 ms). Table 1 lists the patient demographics and clinical and echocardiographic characteristics before implantation (baseline). The baseline echocardiographic findings from the 186 patients was characterized by a mean LVEF of 25.6 ± 7.7% (range 10% to 35%), LV end-diastolic volume of 179 ± 63 ml, LV end-systolic volume of 134 ± 54 ml, and a 10% prevalence of severe mitral regurgitation. The combined criteria (E/E′ and E/Vp) suggested a relative increase in LV filling pressures. Right ventricular function was moderately altered (peak S′ on tissue Doppler imaging).
Characteristic | All (n = 186) | CTR-SRs (n = 18) | CRT-CRs (n = 98) | CRT-NRs (n = 70) |
---|---|---|---|---|
Age (years) | 64.4 ± 12.1 | 63.9 ± 11 | 65.8 ± 9.6 | 64.5 ± 13.4 |
Men | 75% | 72% | 79% | 74% |
New York Heart Association functional class | ||||
III | 92% | 100% | 94% | 87% |
IV | 8% | 0% | 6% | 13% |
Ischemic etiology | 48% | 11% ⁎ (n = 2) | 54% | 50% |
QRS duration (ms) | 157 ± 23 | 150 ± 12 | 159 ± 21 | 151 ± 26 |
Medications | ||||
Diuretics | 88% | 89% | 88% | 89% |
Angiotensin-converting enzyme inhibitors | 78% | 77% | 76% | 81% |
β Blockers | 86% | 94% | 85% | 88% |
Spironolactone | 39% | 40% | 40% | 39% |
Angiotensin II receptor blockers | 25% | 25% | 25% | 26% |
Amiodarone | 15% | 17% | 9% | 28% |
Left ventricular ejection fraction (%) | 25.6 ± 7.7 | 25.5 ± 9.0 | 25.6 ± 8.0 | 25.7 ± 7.2 |
Left ventricular end-diastolic diameter (mm) | 68.2 ± 9.4 | 64.4 ± 8.5 ⁎ | 69.2 ± 9.6 | 69.7 ± 10 |
Left ventricular end-systolic diameter (mm) | 59.2 ± 8.5 | 53.7 ± 6.9 ⁎ | 58.8 ± 9.1 | 59.8 ± 7.3 |
Left ventricular end-diastolic volume (ml) | 179 ± 63 | 149 ± 46 ⁎ | 183 ± 68 | 174 ± 52 |
Left ventricular end-systolic volume (ml) | 134 ± 54 | 117 ± 42 ⁎ | 137 ± 59 | 130 ± 43 |
Mitral valve regurgitation Grade ≥3 | 10% | 5% | 9% | 11% |
Rate of pressure increase in systole (mm Hg/s) | 642 ± 320 | 796 ± 312 ⁎ | 689 ± 256 | 595 ± 227 † |
Left atrial end-systolic volume (ml) | 79.7 ± 39.4 | 50.4 ± 28 ⁎ | 77.3 ± 41.5 | 85.8 ± 33.8 |
E mitral wave deceleration time (ms) | 183 ± 66 | 185 ± 67 | 163 ± 70 | 185 ± 62 |
Isovolumic relaxation time (ms) | 104 ± 46 | 106 ± 43 | 109 ± 50 | 96 ± 38 |
E/E′ | 14.5 ± 7.1 | 13.8 ± 4.4 | 14.1 ± 7.1 | 15.1 ± 7.0 |
E/Vp | 2.2 ± 0.7 | 2.6 ± 0.4 | 2.4 ± 0.9 | 2.3 ± 0.6 |
Systolic pulmonary artery pressure (mm Hg) | 37.5 ± 10.8 | 34.5 ± 5.5 | 37.4 ± 10.7 | 37.8 ± 11.2 |
Right ventricular S′ wave tissue Doppler (cm/s) | 9.0 ± 3.1 | 9.8 ± 7.5 | 9.1 ± 3.3 | 8.9 ± 2.5 |
Global longitudinal strain (%) | −9.2 ± 2.5 | −12.6 ± 2.3 ⁎ | −9.6 ± 2.6 | −8.4 ± 1.9 † |
Basal longitudinal strain (%) | −10.9 ± 3.3 | −14.0 ± 3.4 ⁎ | −11.1 ± 3.3 | −10.2 ± 3.7 |
Mid-longitudinal strain (%) | −8.7 ± 3.0 | −12.6 ± 3.2 ⁎ | −9.0 ± 3.2 | −8.1 ± 2.5 |
Apical longitudinal strain (%) | −7.9 ± 4.1 | −11.6 ± 6.2 ⁎ | −8.2 ± 4.3 | −7.3 ± 3.8 |
Global radial strain (%) | 13.4 ± 6.6 | 13.4 ± 6.5 | 14.5 ± 7.1 | 11.9 ± 5.7 † |
Global transverse strain (%) | 14.4 ± 10.1 | 15.8 ± 8.3 | 16.6 ± 7.7 | 13.2 ± 7.1 |
Global circumferential strain (%) | −8.2 ± 3.5 | −8.1 ± 1.9 | −8.8 ± 3.2 | −7.8 ± 3.7 |
⁎ p <0.05 between CRT-SRs and CRT-CRs;
After 6 months, the New York Heart Association functional class had improved from 3.0 ± 0.4 to 1.9 ± 0.8 (p <0.05), LVEF had improved by 36% (from 25.6 ± 7.7% to 34.8 ± 11%, p <0.0001), LV end-systolic volume by 17% (from 134 ± 53 to 110 ± 51 ml, p <0.001), and LV rate of pressure increase in systole by 20% (from 642 ± 320 to 802 ± 327 mm Hg/s). At 6 months after CRT, 18 (9.7%) of the 186 patients with dilated cardiomyopathy were identified as CRT-SRs, demonstrating functional recovery and concomitant “near normalization” of the LVEF. Of the 186 patients, 98 (52.7%) were identified as CRT-CRs and 70 (37.6%) as nonresponders to CRT. Table 2 lists the clinical and echocardiographic parameters before (baseline) and 6 months after implantation for the CRT-SRs, CRT-CRs, and nonresponders to CRT.
Variable | CRT-SRs | CRT-CRs | CRT-NRs | |||
---|---|---|---|---|---|---|
Baseline | 6 Months | Baseline | 6 Months | Baseline | 6 Months | |
New York Heart Association class | 2.9 ± 0.26 | 1.8 ± 0.44 ⁎ | 3.1 ± 0.33 | 1.8 ± 0.58 ⁎ | 3.0 ± 0.43 | 2.03 ± 0.92 |
Left ventricular ejection fraction (%) | 25.5 ± 9.0 | 55.3 ± 4.5 ⁎ | 25.6 ± 8.0 | 39.4 ± 11 ⁎ | 25.7 ± 7.2 | 26.4 ± 6.4 |
Left ventricular end-diastolic diameter (mm) | 64.4 ± 8.5 † | 56.6 ± 8.7 ⁎ † | 69.2 ± 9.6 | 64.3 ± 9.3 ⁎ | 69.7 ± 9.6 | 68.1 ± 7.1 |
Left ventricular end-systolic diameter (mm) | 53.7 ± 6.9 † | 49.8 ± 7.4 | 58.8 ± 9.1 | 53.7 ± 9.7 ⁎ | 59.8 ± 7.3 | 59.3 ± 6.5 ‡ |
Left ventricular end-diastolic volume (ml) | 149 ± 46 † | 119 ± 38 ⁎ † | 183 ± 68 | 154 ± 62 ⁎ | 174 ± 52 | 185 ± 53 ‡ |
Left ventricular end-systolic volume (ml) | 117 ± 42 † | 54 ± 20 ⁎ † | 137 ± 59 | 95 ± 49 ⁎ | 130 ± 43 | 136 ± 44 ‡ |
Mitral regurgitation grade | 1.1 ± 0.7 | 0.4 ± 0.1 ⁎ | 1.2 ± 0.9 | 0.8 ± 0.8 ⁎ | 1.3 ± 0.9 | 1.2 ± 0.9 |
Rate of pressure increase in systole (mm Hg/s) | 796 ± 312 † | 1,317 ± 486 ⁎ † | 689 ± 256 | 882 ± 380 ⁎ | 595 ± 226 ‡ | 689 ± 208 ‡ |
⁎ p <0.05 between baseline and 6 months;
† p <0.05 between CRT-SRs and CRT-CRs;
No differences were observed at baseline regarding age, gender distribution, New York Heart Association functional status, QRS duration, or medical treatment between the CRT-SRs and CRT-CRs ( Table 1 ). No significant difference was found among the 3 groups concerning the existence of rate-controlled atrial fibrillation (17% of CRT-SRs, 24% of CRT-CRs, and 24% of nonresponders to CRT. However, ischemic etiology was significantly less frequent in CRT-SRs than in CRT-CRs and nonresponders to CRT (p <0.05). CRT-SRs presented with LV dimensions and left atrial volumes that were significantly lower than those in CRT-CRs ( Table 1 ). No significant differences were found regarding the baseline LVEF, E/E′, E/Vp, pulmonary artery pressure, and right ventricular S′ on tissue Doppler imaging.
The feasibility of strain analysis by speckle tracking echocardiography in this population was 99% for longitudinal strain, 78% for transverse strain, 72% for circumferential strain, and 81% for radial strain. Moreover, longitudinal strain was less affected by intra- and interobserver measurement variability in this population (11% and 13% vs 28% and 30% for transverse strain, 20% and 57% for radial strain, and 33% and 50% for circumferential strain, respectively).
With respect to 2-dimensional strain analysis, CRT-SRs had significantly greater longitudinal strain values than CRT-CRs (−12.6 ± 2.3% vs −9.6 ± 2.6%, p <0.001); however, no significant differences were observed for the other strain components. Significant differences in longitudinal strain were observed at each LV level ( Table 1 ).
N o statistically significant differences were found between CRT-SRs and CRT-CRs concerning dyssynchrony parameters. However, radial spatial dyssynchrony and aortic pre-ejection delay >140 ms were observed more frequently in CRT-SRs than in CRT-CRs, and electrosystolic and electromechanical delays were more frequent in CRT-CRs than in CRT-SRs. Nonresponders to CRT presented with an aortic pre-ejection delay >140 ms less often than the other 2 groups.
The CRT-SRs showed, not only improvement in New York Heart Association functional class, but also a greater degree of LV end-systolic volume reduction (−54% vs −30% for CRT-CRs, p <0.01), LVEF gain (+117% vs +54% for CRT-CRs, p <0.01), and improvement in other echocardiographic parameters, such as LV end-diastolic volume and LV diameters, mitral regurgitation severity, and the rate of pressure increase in systole ( Table 2 ).
The areas under the receiver operating characteristic curves were analyzed for each parameter to identify the predictors of LVEF normalization. The optimal cutoff value, sensitivity, and specificity were determined. Using receiver operating characteristic curves, global longitudinal strain was the strongest predictor of CRT-SRs, with a cutoff of −12% (area under the curve 0.87, sensitivity 71%, and specificity 85%, p <0.01; Table 3 ). Table 4 lists the results of univariate analysis of CRT-SR predictors. Global longitudinal strain of ≤−12% (odds ratio 14.1, p <0.0001), left atrial end-systolic volume <55 ml (odds ratio 7.1, p <0.001), nonischemic etiology, LV end-diastolic diameter <69 mm, LV end-systolic diameter <55 mm, LV end-diastolic volume <180 ml, and LV end-systolic volume <96 ml were all significantly associated with an increased probability of LVEF reverse remodeling. Other clinical parameters, such as LVEF level, mitral regurgitation grade, and rate of pressure increase in systole, were not significant predictors of CRT-SR. Concerning the individual parameters of dyssynchrony, univariate analysis was not significant in the prediction of a super-response in contrast to the multiparametric analysis with p values close to statistical significance ( Table 4 ). On multivariate analysis, logistic regression revealed only global longitudinal strain of ≤−12% and left atrial volume <55 ml to be independent predictors of CRT-SRs (adjusted risk 8.3, p <0.01; and adjusted risk 2.1, p <0.05, respectively; Table 5 ). Figure 1 shows an example of global longitudinal strain analysis.
Parameter | AUC | Cutoff | Sensitivity | Specificity | p Value | Asymptomatic 95% CI |
---|---|---|---|---|---|---|
Left ventricular end-diastolic diameter (mm) | 0.654 | 69 | 80% | 52% | 0.08 | 0.624–0.824 |
Left ventricular end-systolic diameter (mm) | 0.709 | 55 | 61% | 74% | 0.07 | 0.702–0.838 |
Left ventricular end-diastolic volume (ml) | 0.63 | 180 | 78% | 58% | 0.07 | 0.549–1.012 |
Left ventricular end-systolic volume (ml) | 0.772 | 96 | 50% | 83% | 0.098 | 0.548–0.996 |
Left ventricular ejection fraction (%) | 0.599 | 30 | 50% | 68% | 0.615 | 0.110–0.818 |
Rate of pressure increase in systole (mm Hg/s) | 0.547 | 680 | 52% | 53% | 0.515 | 0.212–0.890 |
Aortic time-velocity integral (cm) | 0.605 | 16 | 66% | 60% | 0.221 | 0.169–0.870 |
Mitral time-velocity integral (cm) | 0.421 | 15 | 45% | 54% | 0.504 | 0.178–0.661 |
Left atrial volume (ml) | 0.795 | 55 | 64% | 87% | 0.01 | 0.577–0.916 |
S′ right ventricle (cm/s) | 0.526 | 6 | 60% | 67% | 0.110 | 0.210–0.873 |
Global longitudinal strain (%) | 0.871 | −12 | 71% | 85% | 0.009 | 0.722–0.995 |
Basal longitudinal strain (%) | 0.790 | −12.5 | 71% | 89% | 0.02 | 0.699–0.902 |
Mid-longitudinal strain (%) | 0.850 | −11 | 69% | 94% | 0.003 | 0.689–0.930 |
Apical longitudinal strain (%) | 0.735 | −10 | 71% | 82% | 0.107 | 0.620–0.890 |
Variable | Sensitivity | Specificity | p Value | OR | 95% CI |
---|---|---|---|---|---|
Men | 72.2% | 23.9% | 1 | 0.9 | 0.12–2.35 |
Age (years) | |||||
<50 | 20% | 17.4% | 1 | 0.9 | 0.25–6.25 |
50–60 | 30% | 26.1% | 0.9 | 2.4 | 0.34–4.3 |
60–70 | 25% | 28% | 0.8 | 1.3 | 0.25–3.1 |
>70 | 26% | 33% | 1 | 2.01 | 0.44–8.29 |
Nonischemic etiology | 89% | 45% | 0.017* | 3.7* | 1.31–10.6 |
Diuretics | 100% | 15.4% | 0.5 | 1.12 | 1.04–1.21 |
Angiotensin-converting enzyme inhibitors | 78% | 23.7% | 1 | 1.00 | 0.19–5.25 |
Angiotensin II receptor blockers | 25% | 74.6% | 1 | 0.83 | 0.16–4.31 |
β Blockers | 94% | 21.7% | 0.2 | 0.49 | 0.08–1.76 |
Spironolactone | 33% | 66% | 1 | 0.73 | 0.17–3.14 |
Amiodarone | 0% | 88.9% | 0.34 | 0.88 | 0.81–0.95 |
Left ventricular end-diastolic diameter <69 mm | 78% | 52% | 0.04* | 3.6* | 1.0–14 |
Left ventricular end-systolic diameter <55 mm | 61.5% | 74% | 0.02* | 3.7* | 1.31–10.6 |
Left ventricular end-diastolic volume <180 ml | 78% | 58% | 0.03* | 2.8* | 1.1–8.4 |
Left ventricular end-systolic volume <96 ml | 50% | 68% | 0.05* | 2.3* | 0.9–6.4 |
Left ventricular ejection fraction | |||||
<20% | 22.2% | 21.1% | 1 | 0.92 | 0.6–1.6 |
20–25% | 21.6% | 23.8% | 1 | 0.99 | 0.4–4.4 |
25–30% | 23% | 21.8% | 1 | 0.98 | 0.3–4.9 |
30–35% | 33.3% | 33.8% | 0.6 | 1.4 | 0.2–3.4 |
Left atrial volume <55 ml | 86% | 87% | 0.001* | 7.1* | 2.6–18.8 |
Mitral regurgitation grade ≥3 | 7% | 91% | 0.4 | 1.7 | 0.2–12 |
Atrioventricular dyssynchrony | 31% | 67% | 0.9 | 0.9 | 0.12–3.4 |
Interventricular dyssynchrony | 41% | 64.5% | 1 | 1.11 | 0.84–2.45 |
Septal-to-posterior wall delay >130 ms | 46% | 58.6% | 0.4 | 1.21 | 0.38–3.79 |
Radial end-systolic overlap | 14% | 85.5 | 0.6 | 0.98 | 0.4–3.3 |
Longitudinal spatial electromechanical delay >65 ms | 54% | 32.8% | 0.08 | 2.5 | 0.68–9.21 |
Longitudinal spatial electrosystolic delay >65 ms | 45.5% | 34% | 0.1 | 0.39 | 0.11–1.402 |
Longitudinal end-systolic overlap | 60% | 42.6% | 0.7 | 1.2 | 0.4–3.3 |
Aortic pre-ejection delay >140 ms | 50% | 58.7% | 0.6 | 1.42 | 0.53–3.78 |
Presence of 2 criteria of dyssynchrony | 82% | 20% | 1 | 0.8 | 0.2–2.8 |
Presence of 3 criteria of dyssynchrony | 30% | 55% | 0.051* | 2.5 | 0.9–6.8 |
Presence of ≥4 criteria of dyssynchrony | 6% | 88.4 | 0.058* | 4.6 | 0.6–13 |
Global longitudinal strain ≤−12% | 71% | 85% | 0.0001* | 14.1* | 3.8–25.3 |
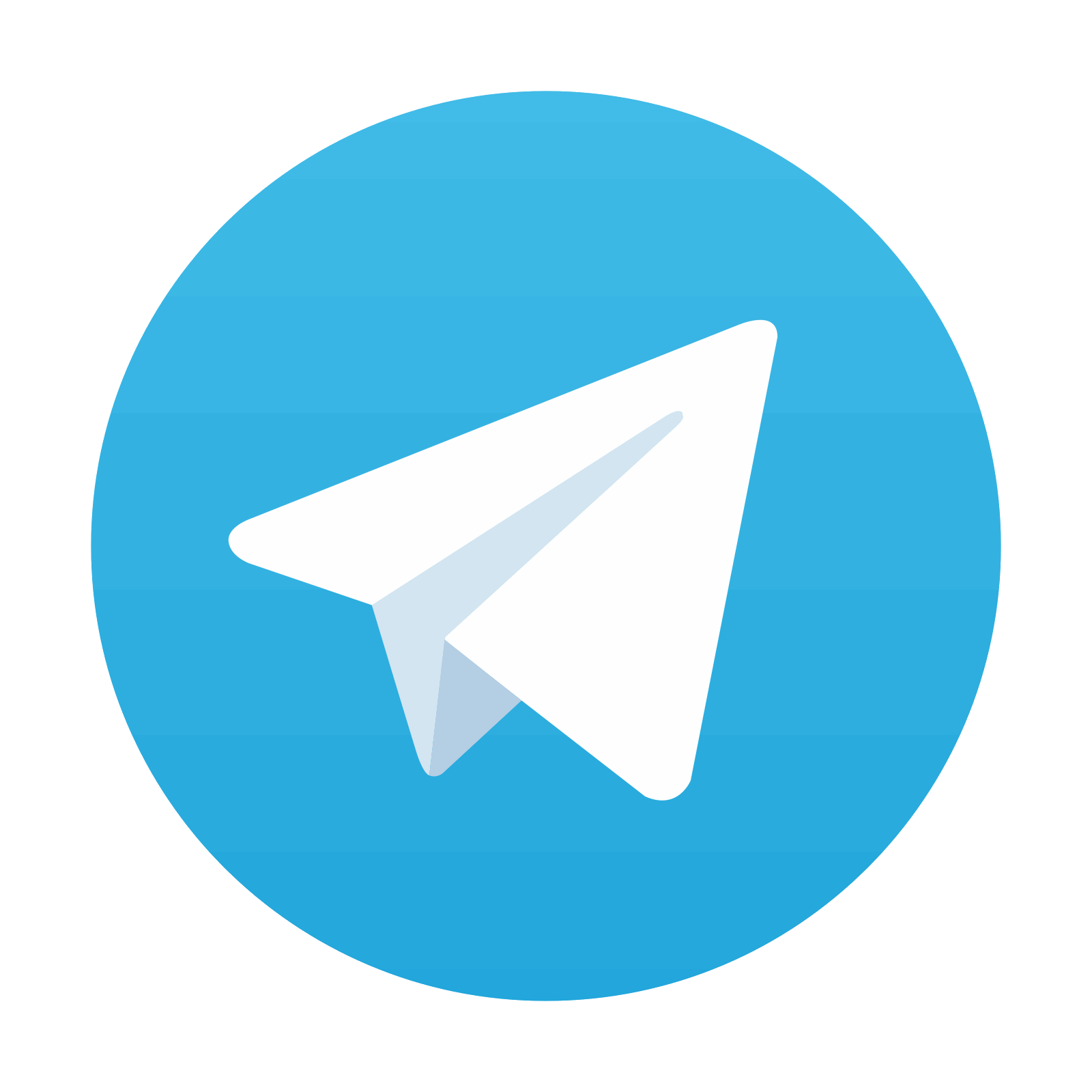
Stay updated, free articles. Join our Telegram channel

Full access? Get Clinical Tree
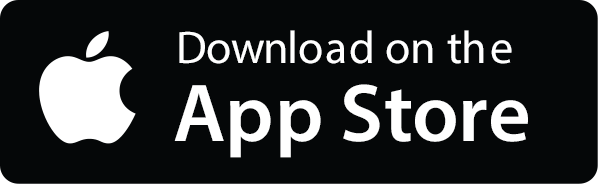
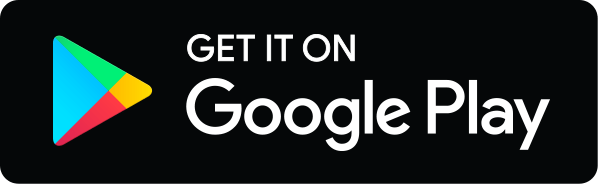
