Fig. 1
High mortality associated with late pulmonary hypertension (PH) in BPD. As illustrated in this schematic, mortality is higher in the severe PH group than BPD infants with lower levels of PH (Adapted from reference [15])
The Abnormal Pulmonary Circulation in BPD
The lung circulation in infants with BPD is characterized by abnormalities of vascular tone and reactivity, structure, and growth, which include a reduction of small pulmonary arteries and a “dysmorphic” pattern of vessel branching within the lung interstitium [5, 9] (Fig. 2). Impaired vascular growth, or angiogenesis, is associated with disruption of alveolarization and reduces the alveolar–capillary surface area, which impairs gas exchange, increases the need for prolonged oxygen and ventilator therapy, causes poor exercise tolerance, and increases the risk for developing PH [17–22].


Fig. 2
Schematic illustration of the pathogenesis and pathobiology of pulmonary vascular disease in BPD (From reference [22])
Mechanisms that coordinate normal vascular growth and alveolarization during development or cause abnormal lung growth in BPD are poorly understood. Disruption of key signals between airway epithelium and endothelial cells can alter vascular and alveolar growth, resulting in decreased arterial and airspace development. Experimental studies have shown that early antenatal or postnatal injury to the developing lung can impair angiogenesis, which further contributes to decreased alveolarization and simplification of distal lung airspace (the “vascular hypothesis” [23–25]). Angiogenic signaling pathways, including those involving vascular endothelial growth factor (VEGF) and nitric oxide are important mediators of normal pulmonary vascular development [25]. Disruption of vascular growth and signaling markedly reduces alveolar–capillary surface area, which contributes to impaired lung diffusion capacity in BPD [26]. Placental overproduction of soluble VEGF receptor-1, which inhibits VEGF signaling, mainly causes maternal endothelial dysfunction and plays a central role in the pathogenesis of pre-eclampsia and can impair pulmonary vascular growth in utero and throughout infancy, even without hyperoxia, mechanical ventilation, or other postnatal insults [27–30]. Intrauterine growth restriction is another condition that has been associated with the disruption of VEGF signaling [25, 31] and an increased risk for BPD and PH in preterm infants [32]. Thus, abnormalities of the lung circulation in BPD are not only related to the presence or absence of PH, but more broadly, PVD, after premature birth as manifested by decreased vascular growth and structure, also contributes to the pathogenesis and abnormal cardiopulmonary physiology of BPD.
These laboratory concepts have recently been demonstrated in a prospective clinical study in which early echocardiogram findings of PVD at day 7 of life was strongly associated with the subsequent diagnosis of BPD and poor respiratory outcomes [13]. Past clinical studies have suggested that sustained elevations of pulmonary artery pressure as assessed by serial echocardiograms may be associated with increased risk for BPD [13, 14, 33–36], supporting the hypothesis that PVD or PH in premature newborns may be an early clinical marker for predicting BPD. Early echocardiographic signs of PVD in preterm infants have now been associated with increased risk for both BPD and late PH as well as with prolonged oxygen treatment [13, 14]. Sustained evidence of elevated right ventricular pressure through the first week after birth may reflect early pulmonary vascular injury that increases risk for BPD. Whether these changes are secondary to delayed transition to extrauterine life, injury due to excessive hemodynamic stress from PDA or other shunts as has been previously reported, or other forms of vascular injury remains to be determined. Understanding the drivers behind these early vascular changes will be crucial to developing novel intervention strategies to prevent both BPD and PH in these infants.
In addition to early hemodynamic indicators of PVD, clinical factors associated with late PH in most studies include lower gestational age, birth weight, and longer periods of respiratory support. Patent ductus arteriosus, infection, oligohydramnios, small for gestational age, and low birth weight z-score have also been identified as risk factors for PH in infants born preterm. Further examination of clinical factors associated with PH, including prenatal risks, along with translational investigations and rigorous screening of infants, will help elucidate how these clinical factors impair normal pulmonary vascular development and lead to BPD and PH.
Early injury to the lung circulation leads to the rapid development of PH after premature birth. Abnormalities of the pulmonary circulation in BPD include increased vascular tone and vasoreactivity, hypertensive remodeling, and decreased growth. Physiological abnormalities of the pulmonary circulation in BPD include elevated PVR and abnormal vasoreactivity, as evidenced by the marked vasoconstrictor response to acute hypoxia [37]. Cardiac catheterization studies have shown that even mild hypoxia can cause marked elevations in pulmonary artery pressure in some infants with BPD, including infants with only modest basal elevations of PH [37]. Increased pulmonary vascular tone contributes to high PVR even in older children with BPD without hypoxia, suggesting that abnormal vascular function persists even late in the course [38].
Abnormal pulmonary vascular structure also contributes to high PVR due to increased smooth muscle cell hyperplasia and altered vascular compliance caused by increased production of an abnormal extracellular matrix. Growth of the distal lung circulation is impaired in infants with severe BPD, and decreased arterial growth reduces vascular surface area that further impairs gas exchange and increases the risk for the development of PH and impaired exercise capacity in older children. Prominent bronchial or other systemic-to-pulmonary collateral vessels were noted in early morphometric studies of infants with BPD, and can be readily identified in many infants during cardiac catheterization. Although these collateral vessels are generally small, large collaterals may contribute to significant shunting of blood flow to the lung, causing edema and need for higher FiO2.
In addition, recent autopsy studies suggest the presence of striking intrapulmonary anastomotic, or “shunt,” vessels that link the distal pulmonary and bronchial vessels, and may contribute to poor oxygenation [39]. Past clinical studies have further shown that metabolic function of the pulmonary vasculature is impaired in BPD, as reflected by the lack of pulmonary clearance of circulating norepinephrine during passage through the lung, which may contribute to left ventricular dysfunction and systemic hypertension [40]. Reduced vascular surface area implies that even relatively minor increases in left-to-right shunting of blood flow through a patent foramen ovale, atrial septal defect (ASD), or patent ductus arteriosus may induce a far greater hemodynamic injury in infants with BPD than in infants with normal lung vascular growth.
Persistent abnormalities of pulmonary vascular growth and/or failure of the lung vasculature to “catch-up” to infants born at term may contribute to PVD that becomes increasingly symptomatic later in life. BPD infants have reduced pulmonary diffusing capacity compared to age-matched term controls, suggesting that BPD infants have decreased alveolar surface area available for gas exchange [26]. These differences were also found in children at 11 years of age who had been born extremely preterm and in adult survivors of BPD. Thus, reduced arterial number, structural abnormalities of the vessel wall, and abnormal vascular function, together with impairments in respiratory mechanics and cardiac function, can contribute to increased PVR and PVD in BPD, leading to significant morbidity and mortality.
Diagnostic Approach to PH in BPD
PH is not only a marker of more severe BPD, but high PVR can also cause right ventricular dysfunction, impaired cardiac output, limited oxygen delivery, increased pulmonary edema, and possibly a higher risk for sudden death. Recent studies suggest that between 15 and 25 % of preterm infants may develop echocardiogram signs of PH or PVD, which strongly relates to disease severity [8, 41, 42] (Fig. 3). Prospective data regarding the precise incidence and natural history of PH in BPD are relatively limited, and most information on diagnostic and therapeutic strategies are based on clinical observations, rather than rigorous, randomized clinical trials.


Fig. 3
Relationship of pulmonary hypertension to severity of BPD. As shown, three distinct studies show that the incidence of PH by echocardiogram is highest in infants with severe BPD (From reference [22])
In general, it is recommended that early echocardiograms be obtained for the diagnosis of PH in preterm infants with severe RDS who require high levels of ventilator support and supplemental oxygen, especially in the setting of oligohydramnios and intrauterine growth restriction (IUGR) ([16]; Table 1; Fig. 4). Infants with more severe prematurity (<26 weeks) are at highest risk for late PH, and infants with a particularly slow rate of clinical improvement, as manifested by persistent or progressively increased need for high levels of respiratory support, should be assessed for PH. In the setting of established BPD, preterm infants, who, at 36 weeks postconceptual age, still require positive pressure ventilation support, are not weaning consistently from oxygen, have oxygen needs at levels disproportionate to their degree of lung disease, or have recurrent cyanotic episodes warrant screening for PH or related cardiovascular sequelae. Other clinical markers often associated with more severe disease include feeding dysfunction and poor growth, recurrent hospitalizations, and elevated PaCO2. High PaCO2, is a marker of disease severity and reflects significant airways obstruction, abnormal lung compliance with heterogeneous parenchymal disease, or reduced surface area, and is an indication for PH screening. Another strategy would be to use echocardiograms to screen every patient at 36 weeks of age who is diagnosed with moderate or severe BPD, but how often clinically significant PH would be missed in patients with milder BPD is uncertain.

Table 1
Diagnostic approach to infants with pulmonary hypertension in BPD
Evaluation of underlying lung disease |
Prolonged monitoring of O2 (awake, asleep, feeds) |
PaCO2 contribution to PH or marker of disease severity, need for chronic (effective) ventilation? |
Chronic aspiration (barium swallow, swallowing study, pH probe, impedance study) |
Sleep study |
Structural airway disease: flexible bronchoscopy |
Reactive airway disease |
Chest CT scan |
Cardiac catheterization |

Fig. 4
Algorithm suggesting a clinical strategy for applying echocardiograms in the care of infants with BPD (From reference [22])
Serial electrocardiograms have inadequate sensitivity and positive predictive value for identification of right ventricular hypertrophy (RVH) as a marker of PH. As a result, obtaining serial echocardiograms for screening for PH in patients with BPD is generally recommended. Estimated systolic pulmonary artery pressure (sPAP) derived from the tricuspid regurgitant jet (TRJV) measured by echocardiogram has become one of the most utilized findings for evaluating PH. Past studies have shown excellent correlation coefficients (r values between 0.93 and 0.97) when compared with cardiac catheterization measurements in children < 2 years old with congenital heart disease [43]. However, these studies evaluated echocardiogram and cardiac catheterization performed simultaneously under the same hemodynamic conditions, and the utility of echocardiograms in predicting disease severity as applied in the clinical setting is less clear.
The utility of echocardiogram assessments of PH in infants with BPD has been compared with subsequent cardiac catheterization measurements of pulmonary artery pressure [44]. Systolic PAP could be estimated in only 61 % of studies, and there was poor correlation between echocardiogram and cardiac catheterization measures of sPAP in these infants. Echocardiogram estimates of sPAP correctly identified the presence or absence of PH in 79 % of these studies, but the severity of PH was correctly assessed in only 47 % of those studies. Seven of 12 children (58 %) without PH by echocardiogram had PH during subsequent cardiac catheterization. In the absence of a measurable TRJV, qualitative echocardiogram findings of PH, including right atrial enlargement, right ventricular hypertrophy, right ventricular dilation, pulmonary artery dilation, and septal flattening, either alone or in combination have relatively poor predictive value. Factors associated with chronic lung disease, specifically marked pulmonary hyperinflation, expansion of the thoracic cage, and alteration of the position of the heart, adversely effect the ability to detect and measure TRJV. As used in clinical practice, echocardiography often identifies PH in infants with BPD, but estimates of sPAP were not obtained consistently and were often not reliable for determining disease severity. Other measures of right ventricular strain and PH, including AT/ET ratio and the Tei index, could be helpful in the absence of a measurable TRJV, but have not been fully evaluated in infants with BPD. Despite its limitations, echocardiography remains the best available screening tool for PH in BPD patients.
In patients with PH by echocardiogram, we generally recommend cardiac catheterization for patients with BPD who: (1) have persistent signs of severe cardiorespiratory disease or clinical deterioration not directly related to airways disease; (2) are suspected of having significant PH despite optimal management of their lung disease and associated morbidities; (3) are candidates for chronic PH drug therapy; (4) have unexplained, recurrent pulmonary edema; or (4) respond poorly to PAH-targeted therapies (Table 2).
Table 2
Guidelines for PH management in BPDa
Screening for PH by echocardiogram is recommended in infants with established BPD (Class I, Level B) |
Evaluation and treatment of lung disease, including assessments for hypoxemia, aspiration, structural airways disease, and the need for changes in respiratory support, is recommended in infants with BPD and PH before initiation of PAH-targeted therapy (Class I, Level B) |
Evaluation for chronic therapy for PH in infants with BPD should follow recommendations for all children with PH and include cardiac catheterization to diagnose disease severity and potential contributing factors such as LV diastolic dysfunction, anatomical shunts, pulmonary vein stenosis, and systemic collaterals (Class I, Level B) |
Supplemental oxygen therapy is reasonable to avoid episodic or sustained hypoxemia and with the goal of maintaining O2 saturations between 92 and 95 % in patients with established BPD and PH (Class IIa, Level C) |
PAH-targeted therapy can be useful for infants with BPD and PH on optimal treatment of underlying respiratory and cardiac disease (Class IIa, Level C) |
The goals of cardiac catheterization are to: assess the severity of PH; exclude or document the severity of associated anatomical cardiac lesions; define the presence of systemic–pulmonary collateral vessels; pulmonary venous obstruction; or left heart dysfunction; and to assess pulmonary vascular reactivity in patients who fail to respond to oxygen therapy alone (Table 2). Other critical information can be acquired during cardiac catheterization that may significantly aid in the management of infants with BPD. In particular, assessment of shunt lesions, especially atrial septal defects; the presence, size, and significance of bronchial or systemic collateral arteries; determining the presence of pulmonary artery stenosis; and structural assessments of the pulmonary arterial and venous circulation by angiography, including pulmonary vein stenosis, are among several key factors that may affect cardiopulmonary function [45].
Most importantly, elevated pulmonary capillary wedge or left atrial pressure may signify left-sided systolic or diastolic dysfunction. LV diastolic dysfunction can contribute to PH, recurrent pulmonary edema, or poor iNO responsiveness in infants with BPD, and measuring changes in PCWP and LAP during acute vasoreactivity testing may help with this assessment. In addition to high pulmonary vascular tone, abnormal vasoreactivity, hypertensive vascular remodeling, decreased surface area, and left ventricular diastolic dysfunction (LVDD) can also contribute to high pulmonary artery pressure in infants with BPD [46]. Up to 25 % of BPD infants with PH who were evaluated by cardiac catheterization had hemodynamic signs of LVDD in one retrospective study [47]. Some infants with LVDD present with persistent requirements for frequent diuretic therapy to treat recurrent pulmonary edema, even in the presence of only mild PH.
Treatment of PH in BPD
The initial clinical strategy for the management of PH in infants with BPD begins with treating the underlying lung disease. This includes an extensive evaluation for chronic reflux and aspiration, structural airway abnormalities (such as tonsillar and adenoidal hypertrophy, vocal cord paralysis, subglottic stenosis, tracheomalacia, and other lesions), assessments of bronchoreactivity, improving lung edema and airway function, and others. Periods of acute hypoxia, whether intermittent or prolonged, are common causes of late PH in BPD (Tables 1 and 2). Brief assessments of oxygenation (“spot checks”) are not sufficient for decisions on the level of supplemental oxygen needed. Targeting oxygen saturations to 92–95 % should be sufficient to prevent the adverse effects of hypoxia in most infants, without increasing the risk of additional lung inflammation and injury. A sleep study may be necessary to determine the presence of noteworthy episodes of hypoxia and whether hypoxemia has predominantly obstructive, central, or mixed causes.
Additional studies that may be required include flexible bronchoscopy for the diagnosis of anatomical and dynamic airway lesions (such as tracheomalacia) that may contribute to hypoxemia and poor clinical responses to oxygen therapy. Upper gastrointestinal series, pH or impedance probe, and swallow studies may be indicated to evaluate for gastroesophageal reflux and aspiration that can contribute to ongoing lung injury. For patients with BPD and severe PH who fail to maintain near-normal ventilation or require high levels of FiO2 despite conservative treatment, consideration should be given to chronic mechanical ventilatory support. Despite the growing use of pulmonary vasodilator therapy for the treatment of PH in BPD, data demonstrating efficacy are extremely limited, and the use of these agents should only follow thorough diagnostic evaluations and aggressive management of the underlying lung disease. Current therapies used for PH therapy in infants with BPD generally include inhaled NO, sildenafil, endothelin-receptor antagonists (ERA), prostacyclin analogs, and calcium channel blockers.
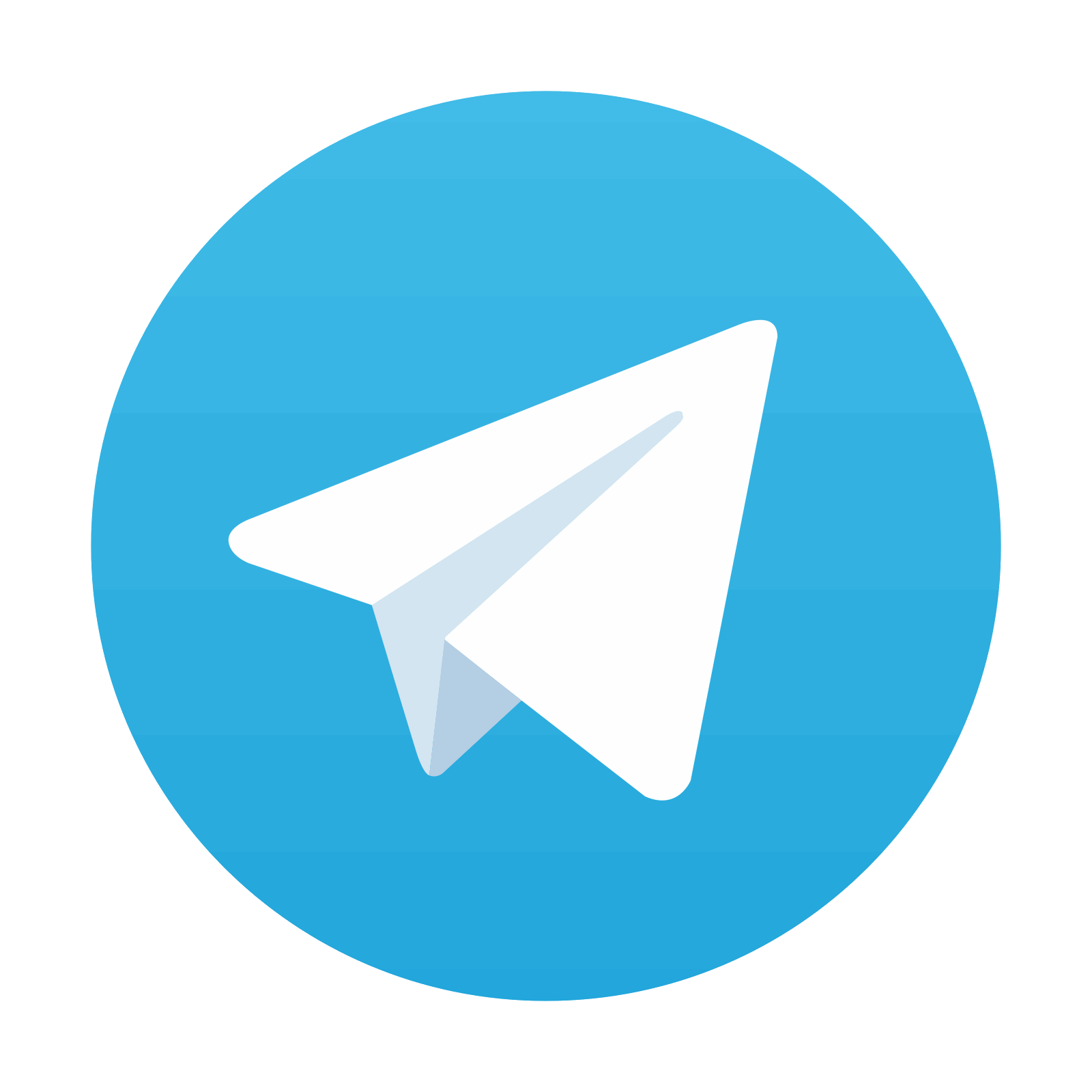
Stay updated, free articles. Join our Telegram channel

Full access? Get Clinical Tree
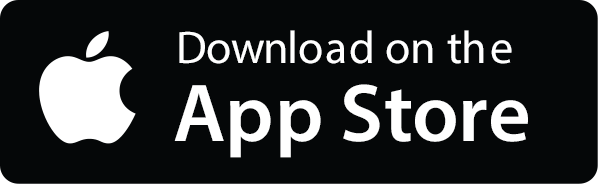
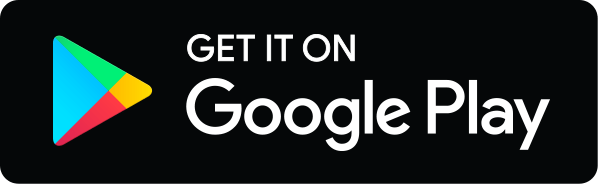