Hypertension accounts for 6% of deaths worldwide. In Canada, the overall prevalence of hypertension was 22% and was higher in men (26%) than in women (18%) in a survey done between 1986 and 1992.1 Hypertension prevalence increases progressively with age, being lower in women than men aged 18 to 34 years (2% vs.11%) and aged 35 to 64 years (21% vs. 31%).1,2 However, in the group aged 65 to 74, women had a higher prevalence than men (58% vs. 56%). The overall prevalence of hypertension in the United States in 2004 was 72 000 000 (33 000 000 males; 39 000 000 females). A higher percentage of men than women have hypertension until age 45 years. The percentage of hypertension is similar in men and women between the ages of 45 and 54. After the age of 54, a higher percentage of women have hypertension than men. It is estimated that middle-aged North Americans have a 90% life-time risk of developing hypertension. The prevalence of hypertension is higher in southeastern United States as compared to other regions.3 Hypertension in black men and women in the southern states is higher (35–34%) as compared to other parts of the United States (27–33%). The prevalence of hypertension in white men and women in the southern United States is slightly higher (21.5% to 26.5%) as compared to other parts of the United States (21% to 24.3%). When Canadian survey data were compared to similar data from the United States, hypertension prevalence was similar between the two countries (20.1% vs. 21.1%, Canadian vs. American), but awareness of the diagnosis (69% vs. 57%), and treatment of hypertension (52% vs. 34%) were substantially higher in the United States than in Canada.1 Recent data from the United States show a further improvement in treatment and control rates. There was a large increase in diagnosis (51%) and treatment (66%) of hypertension between 1994 and 2003.4 There is substantial evidence that the patient management guidelines are not followed frequently, and the current practice patterns present a major barrier to treatment and control of hypertension.5 It is not only the treatment practice but also the pathophysiology of the disease that affects the outcome. There has been advancement in understanding the mechanism of the disease and in diagnostic and treatment modalities. This chapter will deal with the definition of hypertension, measurement of blood pressure, epidemiology, pathophysiology, complications, and treatment.
The higher the pressure, the worse the prognosis. The prognosis is based not on whether hypertension is thought to be present, but on the actual recorded pressure. However the question is “At what pressure level should treatment be instituted?” The dividing line between normotension and hypertension is arbitrary. The decision to treat should be based on the evaluation of risk. The reason for a dividing line is to initiate treatment. The risk associated with blood pressure increases monotonocally with blood pressure, hence the benefit of treatment is progressively higher at higher levels of blood pressure. Blood pressure has been classified into four groups (Table 9-1) by the Joint National Committee on Prevention, Detection, Evaluation and Treatment of High Blood Pressure (JNC-7).6 Normal blood pressure is systolic <120 mm Hg and diastolic <80 mm Hg. Hypertension is classified into three groups: prehypertension, stage 1 and stage 2 hypertension. The European Society of Hypertension—European Society of Cardiology guidelines in 2003 classified blood pressure into seven categories (Table 9-1): optimal, normal, high normal, Grade 1 hypertension (mild), Grade 2 hypertension (moderate), Grade 3 hypertension (severe), and isolated systolic hypertension (ISH).7 According to the Framingham criteria, the terms low, moderate, high, and very high added risk indicate a 10 year risk of cardiovascular disease of <15%, 15% to 20%, 20% to 30%, and >30%, respectively.
JNC-7 Guidelines Class | Systolic (mm Hg) | Diastolic (mm Hg) |
---|---|---|
Normal | <120 | <80 |
Prehypertension | 120–139 | 80–89 |
Hypertension (stage 1) | 140–159 | 90–99 |
Hypertension (stage 2) | ≥160 | ≥100 |
European Societies of Hypertension and Cardiology Guidelines∗ | ||
Optimal | <120 | <80 |
Normal | 120–129 | 80–84 |
High normal | 130–139 | 85–89 |
Hypertension Grade 1 (mild) | 140–159 | 90–99 |
Hypertension Grade 2 (moderate) | 160–179 | 100–109 |
Hypertension Grade 3 (severe) | ≥180 | ≥110 |
Isolated systolic hypertension | ≥140 | ≥90 |
ISH is a separate class in itself. It can be graded as 1, 2, and 3 according to the systolic pressure ranges indicated in Table 9-1. Systolic pressure rises almost linearly between the ages of 30 and 84 years, while diastolic pressure rises until age 50 years, levels off, and then declines. More than 90% of untreated hypertensive subjects in the United States have ISH by the time they reach 70 to 75 years of age.8 ISH accounts for 57.4% of all hypertension in men and 65% in women aged 65 to 89 years. ISH is associated with increased risks of all cardiovascular disease complications and is an independent risk factor. For every 1 mm Hg elevation in systolic blood pressure there is a 1% increase in all causes of mortality. Pulse pressure indicates stiffening of the aorta and is an accurate predictor of cardiovascular risk in normotensive subjects, and a predictor of recurring events in patients with impaired left ventricular function after myocardial infarction.
Accurate measurement of blood pressure is of utmost importance for the diagnosis of hypertension. Presently, blood pressures are being measured by (a) physicians in office (office measurement or auscultatory method), (b) self (self-measurement), and (c) 24 hour measurement (ambulatory measurement).
The gold standard for clinical blood pressure measurement is by the sphygmomanometer and the Korotkoff sound technique. However, the auscultatory office blood pressure measurement has shortcomings such as (a) inaccuracy in methods, which includes mechanical defects and noncompliance of official guidelines for their use; (b) inherent variability of blood pressure; and (c) the white-coat effect (WCE).6,9
The inaccuracy in blood pressure measurement is related to cuff size. The ideal cuff should have a bladder length that is 80% and a width that is at least 40% of the arm circumference (a length-to-width ratio of 2:1). The cuff should be at the level of the right atrium. Diastolic pressure is approximately 5 mm Hg higher in the sitting position than in the supine position, while systolic pressure is 8 mm Hg higher in the supine than in the sitting position. Crossing of the legs raises systolic pressure by 2 to 8 mm Hg. The blood pressure is high when the arm is below the level of the right atrium, and low when the arm is above the level of right atrium. For every inch below or above the heart level, there is a change of 2 mm Hg. If the arm is held up by the individual the isometric exercise will raise the pressure. The mercury column should be deflated at 2 to 3 mm/s. Neither patient nor the physician should talk during the measurement. Blood pressure should be checked in both arms. The first reading is the highest. A minimum of two measurements should be made at intervals of 1 minute, and the average should be recorded. If the difference between the first and second readings is more than 5 mm Hg, an additional one or two readings should be taken to calculate the average.
WCE is defined as a transient rise in blood pressure and sometimes in heart rate, in the presence of the health professional, that disappears when the patient has left the office. This phenomenon occurs in both normotensive and hypertensive subjects irrespective of medications. In many cases it diminishes or disappears with repeated measurements. As a result of the WCE, an individual has elevated blood pressure in the physician’s office but a normal blood pressure outside the office, a phenomenon called white-coat hypertension (WCHT).10 Office blood pressure values equal to or higher than 140 mm Hg systolic and/or 90 mm Hg diastolic, with self-measured or daytime ambulatory blood pressure lower than 135 mm Hg systolic and 85 mm Hg diastolic, is defined as WCHT. The prevalence of WCHT varies from 15% to over 50% in patients with mildly elevated blood pressure, 25% to 35% in patients with diagnosed hypertension and around 10% in the population at large and in clinical practice. The study of Verberk el al10 showed that 33% of the patients having mild-to-moderate hypertension consistently had a substantial WCE and 14% had WCHT. WCHT has been associated with a significantly lower incidence of cardiovascular events than sustained hypertension. However, some studies suggest that the rate of cardiovascular events is higher in individuals with WCHT than in normotensives. Subjects with WCHT have a more marked morning blood pressure surge and greater daytime blood pressure variability compared to normotensives, although significantly lower than hypertensives. The cardiovascular risk of individuals with WCHT and normotensives is fairly similar and WCHT is benign compared to hypertension.
The aneroid sphygmomanometer measures blood pressure by a mechanical system of metal bellows that expand as the cuff pressure increases, and a series of levers that register the pressure on a circular scale. The stability of this system over time deteriorates and requires calibration. The inaccuracy of the aneroid devices varies between 1% and 44%.
Recently, automated oscillometric blood pressure devices are being used for office, home, and ambulatory pressure measurements. The advantages of automated methods include elimination of observer error, minimizing WCE, and increasing the number of readings. However, this method has a few disadvantages, that is, inherent error in the oscillometric method and epidemiologic data are based on the auscultatory method. The devices should be validated before the reading is accepted. The validation requires comparison of the device measurements (four measurements) alternating with five mercury sphygmomanometer measurements. Validation requires that both systolic and diastolic pressures are within 5 mm Hg of each other for at least 50% of the readings.
Several types of blood pressure monitoring devices are available for home use. Self-monitored blood pressure may be more representative of 24 hour blood pressure. The reproducibility of self-measurement is twice as good as the clinic blood pressure. The advantages of self-monitoring blood pressure are that it (i) distinguishes sustained hypertension from WCHT, (ii) improves patient adherence to treatment, and (iii) reduces management costs.
Ambulatory blood pressure measurement (ABPM), a non-invasive fully automated method, measures blood pressure during daily activities and sleep every 15 to 30 minutes. The devices measure blood pressure commonly by oscillometry or the auscultation method. The oscillometric measurement depends upon detection of pulsatile oscillations of the brachial artery in the cuff. The oscillometric technique is accurate for midrange blood pressure, but is less accurate in older patients or in patients with low or high blood pressure. Blood pressure has a reproducible circadian rhythm with higher values while awake, and mentally and physically active, and much lower values during sleep, and an early morning surge. The suggested daytime optimal, normal, and abnormal systolic and diastolic pressures are <130/80, <135/85, and >140/90 mm Hg, respectively, night time values are <115/65, <120/70, and >125/75 mm Hg, respectively and 24 hour averages are <125/75, <130/80 and >135/85 mm Hg, respectively. In most individuals the blood pressure drops by 10% to 20% during the night. Individuals with a nondipping pattern (<10%) are at increased risk of cardiovascular events. Individuals whose 24 hour blood pressure is greater than 135/85 mm Hg are nearly twice as likely to have a cardiovascular event as those with 24 hour mean blood pressure of <135/85 mm Hg, irrespective of office blood pressure level.6 ABPM is helpful in the following clinical situations; WCHT, hypertension with or without target organ involvement, antihypertensive drug resistance, episodic hypertension, automomic dysfunction, and hypotensive symptoms with antihypertensive medication.
Hypertension is of two types: (I) essential or primary hypertension where the etiology is unknown. This type of hypertension accounts for approximately 90% to 95% of all hypertension, and (II) secondary hypertension where the etiology is known. The prevalence of this type of hypertension is 5% to 10%.
Essential hypertension, also called primary or idiopathic hypertension, is hypertension of unknown etiology. The mechanism(s) responsible for essential hypertension is complex because of the numerous factors involved in the regulation of blood pressure and their complex interactions. Hypertension seems to be a reflection of separate disease processes. Essential hypertension may likely have a number of discrete etiologies.
Approximately 50% of hypertension is genetically controlled and the other 50% environmentally controlled. First degree relatives of patients with hypertension have a twofold greater risk of hypertension and this risk increases to fourfold when more family members are hypertensive. A family history of hypertension in blacks increases the risk of hypertension ninefold. A family history of hypertension at an early age increases the risk. Essential hypertension is a polygenic disorder. More than 60 genes have been studied and the genetic variants associated with hypertension in different ethnicities have been identified. These variants are genes from the renin—angiotensin (Ang)–aldosterone system, adrenergic receptor system, renal kallikrein–kinin system, α-adducin, and epithelial sodium channel. Some of the genes, such as Ang-converting enzyme, angiotensinogen, Ang II type 1 receptor, aldosterone synthase, glucocorticoid receptor, α-adducin, α-2 adrenergic receptor, β-2 adrenergic receptor, epithelial sodium channel, kallikrein, G-protein β-3 subunit, and WNK4, have either familial aggregation and linkage or an association to essential hypertension.11,12,13 Many of these genes encode proteins that are directly or indirectly involved with renal ion transport. The genes encode angiotensinogen, Ang-converting enzyme, α-adducin, G-protein β3 subunit, sodium epithelial channel and WNK kinases. Gene-by-environment interactions between genetic variations and environmental factors contribute to the development of essential hypertension.
Salt sensitive individuals respond to high salt intake with an increase in blood pressure, while salt resistant individuals do not. Fifty-one percent of hypertensives and 26% of normotensive are salt sensitive. The subjects whose blood pressure rises with high salt diet and falls with salt restriction are salt sensitive individuals. The hypertension in salt sensitive hypertensives may be because of changes in renin activity, increased activity of the sympathetic nervous system, and chloride ions. Genetic marker candidates for salt sensitivity may be angiotensinogen and α-adducin. α-adducin induces signal transduction in renal tubular cells and regulates sodium reabsorption. With unaltered sodium intake, the incidence of hypertension in the AA genotype of angiotensinogen is more than in the GG genotype. Increased insulin resistance and abnormalities in glucose disposal are observed in normotensive salt sensitive individuals and salt sensitive subjects with essential hypertension.14 Insulin resistance could also be involved in hypertension through accumulation of aldehydes.15
Insulin resistance and hyperinsulinemia are associated with hypertension. The mechanism of hypertension in individuals with insulin resistance could be multifactorial. Insulin increases the activity of the sympathetic nervous system leading to increases in cardiac output and peripheral vascular resistance.16 Insulin is known to increase sodium and water retention through its effect on renal tubules. Insulin-induced hypertension may be mediated through its effect at the cellular level. It has been shown that in insulin resistance, glucose metabolism through the glycolytic pathway is impaired, resulting in accumulation of glyceraldehyde and glyceraldehyde-3-phosphate. These metabolites are further metabolized to the highly reactive aldehyde, methylglyoxal. Methyloglyoxal binds with free sulfhydryl (SH) and amino groups of membrane proteins forming aldehyde conjugates, leading to altered cellular structure and function.17 Excess aldehyde binds SH groups of the calcium channel leading to increased cytosolic [Ca2+]i and hence increased vascular resistance.15,18 Aldehydes may also alter the function of enzymes, including antioxidant enzymes and nitric oxide synthase, and vascular tissue proteins that play a role in hypertension.15,19 Chronic dietary administration of methylglyoxal to rats increases tissue aldehyde conjugate, cytosolic [Ca2+]i and blood pressure, and decreases plasma levels of nitric oxide.15 Elevated levels of [Ca2+]i and decreased levels of [Mg2+]i have been reported in patients with essential hypertension, and insulin resistance.
It is generally accepted that sodium ions play a major role in hypertension. However suggestions have been made that chloride ions may also play a role in hypertension. In support of this, it has been shown that feeding of chloride ion-free sodium salts to salt sensitive rats does not produce hypertension. As mentioned in the preceding section, [Ca2+]i levels are high in patients with hypertension. Intracellular calcium is influenced by the extracellular environment. Low renin hypertensive subjects have lower serum ionized Ca2+ and calcitonin levels, and higher serum Mg2+, parathormone and 1,25-dihydroxycholecalciferol as compared to normotensive individuals. Intracellular calcium is elevated in all types of hypertension. However, there are variations (lower/higher) in the levels of extracellular Ca2+ and Mg2+.
Obesity and recent weight gain account for 70% of new onset hypertension. The mechanism of hypertension in obese subjects is not definitely known. Increased sodium intake does not explain the etiology of hypertension in obese individuals because continued salt intake with weight loss lowers blood pressure. Obesity is associated with insulin resistance, hyperinsulinemia, salt-sensitivity, and hyperleptinemia. Insulin and leptin increase sympathetic activity that would increase blood pressure. Increased renal sympathetic activity would cause salt retention via the reabsorption of sodium in the renal tubules.
Renin has been implicated in essential hypertension. There are three types of essential hypertension based on plasma renin levels: low renin, high renin, and nonmodulating.
The prevalence of low renin essential hypertension is 20%. These patients have increased extracellular fluid possibly because of sodium retention, and renin suppression because of increased levels of unidentified mineralocorticoids. The adrenal cortex may be more sensitive to Ang II. However, altered sensitivity to Ang II has been observed in patients with normal renin hypertension.
The prevalence of high renin essential hypertension is approximately 15%. The high renin levels may be secondary to increased sympathetic activity.
The prevalence of nonmodulating hypertension is 25% to 30%. In nonmodulating essential hypertension, the adrenal response to sodium reduction is reduced. Sodium intake does not modulate adrenal or renal vascular responses to Ang II, and this has been termed nonmodulating hypertension. These individuals have high or normal levels of plasma renin activity (PRA).
Recently, oxygen radicals (ORs) have been implicated in the pathophysiology of hypertension. Sources of ORs have been described in detail earlier.20 Exogenously generated ORs have been shown to increase systemic and pulmonary vascular resistance.21,22 Superoxide anion (O−2)23 and hydrogen peroxide (H2O2)24 induce increased vascular tone in rabbit aorta. ORs have been implicated in the pathophysiology of human essential hypertension.25,26,27 Antioxidant status is decreased in essential hypertension.28 Although there are various sources of ORs, NADPH oxidase seems to be the most important in the causation of hypertension. NADPH oxidase is present in the vascular endothelial cells, vascular smooth muscle cells, polymorphonuclear leukocytes, and adventitial fibroblasts.29 The role of vascular NADPH oxidase in the development and progression of hypertension in numerous animals has been established.30,31 Ang II induced hypertension is associated with oxidative stress.29 Ang II upregulates NADPH oxidase.29 Genetic factors, including polymorphism in the genes encoding the NADPH oxidase subunits, modulate O−2 production, influencing hypertension.32 ORs could contribute to development of hypertension in some other ways besides direct vasoconstriction. Superoxide anions combine with nitric oxide to form peroxynitrite thereby reducing the bioavailability of nitric oxide, a vasodilator. Peroxynitrite is a weak vasodilator compared to nitric oxide. Critical imbalances between superoxide anions and nitric oxide could lead to the development of hypertension. Superoxide anion is destroyed by superoxide dismutase. However, the reaction between superoxide anion and nitric oxide is three times faster than the reaction between superoxide anion and superoxide dismutase. ORs produce endothelial dysfunction, thus reducing the release of vasodilator substances such as prostanoids and nitric oxide. In such circumstances, there is unopposed action of circulating vasoconstrictors resulting in a rise in blood pressure.
C-reactive protein (CRP) is an inflammatory marker synthesized and secreted mainly by hepatocytes in response to the cytokines interleukin-6 and tumor necrosis factor-alpha. It is markedly increased in inflammatory diseases, acute or chronic.33 Hypertension is associated with increased CRP.34 Higher levels of high sensitive CRP in patients with hypertension as compared to normotensive individuals have been reported.35 A cross-sectional study has correlated CRP with blood pressure. CRP directly stimulates polymorphonuclear leukocytes to produce ORs.36 ORs could produce hypertension through direct vasoconstriction and endothelial cell dysfunction. There are, however, no longitudinal data to support the role of CRP in the development of hypertension.
Advanced glycation end products (AGEs) are a heterogeneous group of adducts resulting from nonenzymatic glycation and oxidation of proteins, lipids, and nucleic acids. Glucose and other reducing sugars produce AGEs. AGE formation proceeds slowly under normal glycemic conditions, but is accelerated in the presence of hyperglycemia and oxidative stress. There are mainly two receptors for AGE (RAGE): full length (RAGE) and soluble receptor for AGEs (sRAGE). Binding of AGE with full length receptor results in activation of nuclear factor-kappaB (NF-kB), increased expression of cytokines, adhesion molecules and tissue factor, and induction of oxidative stress. sRAGE acts as a decoy for RAGE ligands. This occurs by sequestering circulating AGEs or competing with full length RAGE. The interaction of AGE and RAGE has been implicated in the pathogenesis of hypertension, atherosclerosis, coronary artery disease, diabetic complications, and restenosis, as reviewed earlier.37 Several studies suggest a role of AGE in the progression and maintenance of hypertension. AGE and RAGE interaction may produce hypertension through oxygen radical generation and expression of vascular cell growth factor. ORs are known to increase vascular tone.21,22,23,24 sRAGE levels in the plasma are lower in patients with hypertension compared to normotensive individuals.38,39 sRAGE levels are inversely correlated with systolic blood pressure and pulse pressure. It appears that sRAGE is a marker for essential hypertension.
Homocysteine (Hcy) is a sulfur-containing amino acid derived from methionine and is essential for a number of biochemical processes including the metabolism of nucleic acids, fats, and high energy bonds. The synthesis and metabolism of Hcy involve three processes: demethylation, transmethylation, and transsulfuration, and are reviewed in detail elsewhere.40 There are various ways by which Hcy is elevated in plasma and tissue: (i) methionine-rich protein diets, (ii) enzyme deficiency, (iii) vitamin deficiency, (iv) drugs, and (v) certain diseases.40 Hyperhomocysteinemia is commonly associated with vitamin B12 and folic acid deficiency, the heterozyous/homozygous trait for β synthase, and polymorphism of methylenetetrahydrofolate reductase C677T.
Recently hyperhomocysteinemia has been implicated in the pathophysiology of hypertension.41,42,43,44 Hcy, through various mechanisms could lead to endothelial cell dysfunction. Endothelial cell injury could be caused by generation of ORs by Hcy.40 Autooxidation of Hcy generates superoxide anions and H2O2. Hcy also reduces antioxidant status. Endothelial dysfunction would reduce the formation of vasodilatory prostanoids and nitric oxide, and circulating vasoconstrictors in the blood would then have unopposed action on the vessels, resulting in hypertension.40
Hcy combines with nitric oxide to form s-nitroso-homocysteine, which is a weak vasodilator. ORs generated by Hcy would also destroy nitric oxide. These effects would reduce the bioavailability of nitric oxide resulting in the development of hypertension.40 Nitric oxide combines with superoxide anion to form peroxynitrite in the mitrochondria. Peroxynitrite in the presence of thiol causes nitration of tyrosine, forming nitrotyrosine. Hcy thus could reduce the bioavailability of nitric oxide.45
Hcy breaks downs elastin through activation of matrix metalloproteinases (MMPs), which could lead to vascular stiffness.45 Hcy proliferates vascular smooth muscle cells46 and elicits calcium-dependent vascular contraction. These structural changes with Hcy lead to an increase in vascular resistance resulting in hypertension.
In summary, Hcy causes hypertension by (i) increasing ORs, (ii) decreasing bioavailability of nitric oxide and other vasodilator metabolites, (iii) increasing matrix metalloproteinase activity causing collagenolysis and increasing resistance, and (iv) altering the vascular matrix resulting in a decrease in the vascular lumen.
There are numerous causes of secondary hypertension. Some of the important causes are shown in Table 9-2. The mechanism of hypertension and the diagnosis criteria are described briefly in the following section.
|
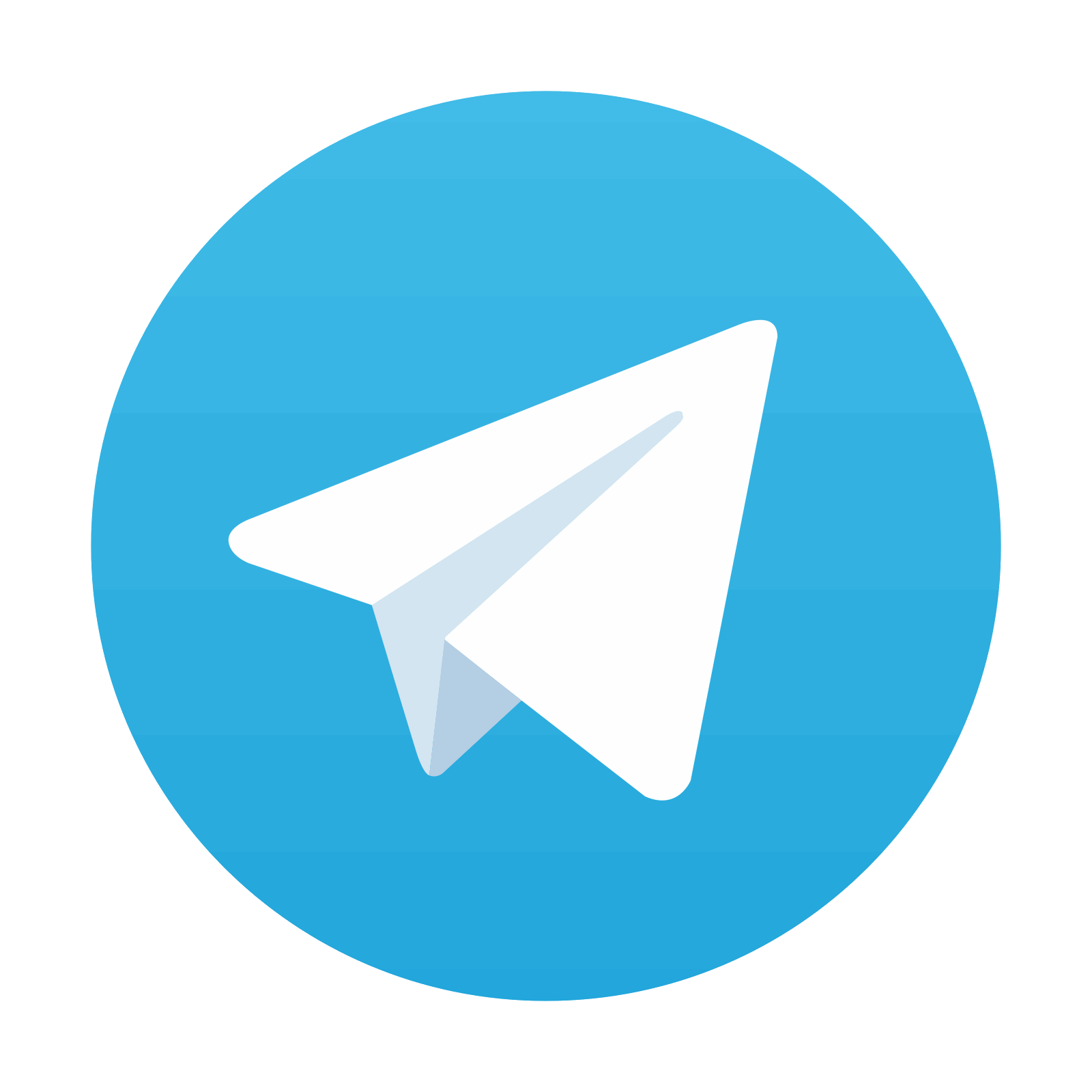
Stay updated, free articles. Join our Telegram channel

Full access? Get Clinical Tree
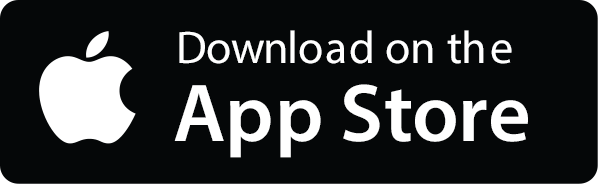
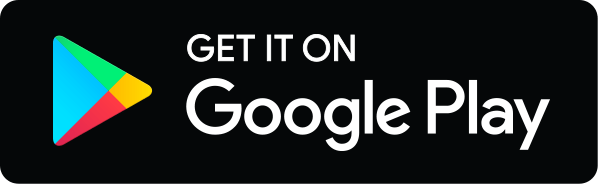
