Abstract
This chapter examines a variety of immunologic-mediated lung diseases. Hypersensitivity pneumonitis is caused by exposure to a variety of antigens that elicit a Th1-mediated hypersensitivity response in the lungs. In contrast eosinophilic lung disease is typically a Th2-mediated response manifested by a prominent eosinophilic inflammatory response in the lungs. Eosinophilic lung diseases in which a causative antigen exposure has been identified include allergic bronchopulmonary aspergillosis, drug induced eosinophilia, and helminth associated eosinophilic lung disease. There are other eosinophilic lung diseases in which a causative exposure has not been identified, such as acute and chronic eosinophilic pneumonia, eosinophilic granuloma, and Churg-Strauss syndrome. Finally, hypereosinophilic syndrome, a systemic eosinophilic disorder in which the lung may be affected, is described.
Keywords
bird fancier’s lung, Aspergillus fumigatus , hypersensitivity pneumonitis, allergic bronchopulmonary aspergillosis, drug-induced eosinophilia, acute eosinophilic pneumonia, chronic eosinophilic pneumonia, eosinophilic granuloma, helminth associated eosinophilic lung disease, Churg-Strauss syndrome, hypereosinophilic syndrome
Hypersensitivity Pneumonitis
Epidemiology
Hypersensitivity pneumonitis (HP), also known as extrinsic allergic alveolitis, is an immune mediated lung disease occurring in response to repeated inhalation of an antigen. It appears to be an underdiagnosed condition, often masquerading as a recurrent pneumonia, idiopathic pulmonary fibrosis, Hamman-Rich disease, or interstitial pneumonia. Extrinsic allergic alveolitis seems particularly appropriate as terminology, because it describes the disease in graphic terms: “extrinsic,” meaning it comes from an outside source; “allergic,” denoting a hypersensitivity basis; and “alveolitis,” referring to that part of the lung most affected by the disease. Whatever term is used, HP is a hypersensitivity reaction of the lung in response to inhalation of an antigen—most often an organic dust. The prevalence and incidence of HP is difficult to estimate. Among individuals exposed to a known antigen(s), the prevalence of pigeon breeder’s disease HP is 6%–20% and the prevalence of farmer’s lung is 0.5%–19% among farmers. The incidence rate of HP at a Danish referral center was 2 cases per year over a 12-year period during a national cohort study on interstitial lung disease.
Etiology
There are more than 200 antigens known to cause HP ( Table 65.1 ). The etiology of HP differs between adults and children and is due to differences in exposure. The majority of adult cases of HP are due to various occupational exposures. In children, most cases are due to household exposures, with avian antigens being the most common etiology, followed by molds. There are multiple categories of antigenic sources, including fungal, bacterial and amoebal proteins, animal proteins, and chemicals and medications (see Table 65.1 ). In addition, there are a number of factors that determine how one will respond to inhalation of an organic dust. First, what is the basic immunologic reactivity of the host? An atopic individual will characteristically respond with production of IgE antibody. A nonatopic person will more likely produce IgG. Second, what is the nature and source of the antigen? Is it small enough to reach the distal part of the lung? Is it temperature-required such that it will grow in the respiratory tract, such as Aspergillus? Finally, consider the nature and circumstances of the exposure: Is it intense and intermittent or is it low-grade and chronic? An intermittent short-term intensive exposure such as that experienced by a pigeon breeder while cleaning out the coops will manifest acute reversible disease. A pet-store employee who has intermittent, lower grade but long-term exposure will develop a subacute form that is usually reversible. Finally, a long-term low-grade exposure experienced by a parakeet owner may result in chronic irreversible disease.
Disease | Source | Antigen |
---|---|---|
BACTERIA | ||
Farmer’s lung | Moldy hay, moldy plant materials | Faeni rectivirgula Saccharopolyspora rectivirgula, Thermoactinomyces vulgaris Thermoactinomyces actinomycetes Thermoactinomyces sacchari Aspergillus spp |
Bagassosis | Moldy sugar cane | Thermoactinomyces sacchari Thermoactinomyces vulgaris |
Ventilation pneumonitis | Contaminated air conditioners | Thermoactinomyces candidus Thermoactinomyces actinomycetes Thermoactinomyces sacchari Naegleria gruberi Acanthamoeba polyphagia Acanthamoeba castellano |
Humidifier lung | Ultrasonic cool mist humidifiers | Bacillus subtilis Klebsiella oxytoca |
Mushroom worker’s lung | Moldy compost and mushrooms | Thermoactinomyces sacchari |
Machine operator’s lung | Contaminated/aerosolized metal working fluid | Nontuberculous mycobacteria Aspergillus fumigatus Mycobacterium immunogenum |
Floor finisher’s lung | Moldy wood | Cephalosporium acremonium |
Hot tub lung | Hot tub mists, tub water, mold on ceiling | Mycobacterium avium complex |
Swimming pool lung | Mist from pool water, sprays, fountains | Mycobacterium avium complex |
FUNGI | ||
Enoki mushroom worker’s lung (Japan) | Moldy mushroom compost | Penicillium citrinum |
Composter’s lung | Compost | Aspergillus spp Thermoactinomyces vulgaris |
Peat moss HP | Contaminated peat moss | Monocillium spp Penicillium citreonigum |
Suberosis | Moldy cork | Thermoactinomyces viridis Aspergillus fumigatus Penicillium spp |
Malt worker’s lung disease | Moldy or contaminated barley | Aspergillus clavatus Aspergillus fumigatus |
Sequoiosis | Mold redwood dust | Aureobasidium pullulans Pullularia spp Graphium spp Trichoderma spp |
Maple bark stripper’s lung | Contaminated maple logs | Cryptostroma corticale |
Winemaker’s lung | Mold on grapes | Botrytis cinerea |
Tobacco grower’s lung | Mold on tobacco, tobacco plants | Aspergillus spp |
Woodman’s disease | Mold on oak and maple trees | Penicillium spp |
Potato riddler’s lung | Moldy hay around potatoes | Thermoactinomyces actinomycetes Faeni rectivirgula Thermoactinomyces vulgaris Aspergillus spp |
Cheese washer’s lung | Moldy cheese casing | Penicillium casei Aspergillus clavatus |
Thatched roof lung | Dead/dried grasses and leaves | Saccharomonospora viridis Thermoactinomyces vulgaris Aspergillus spp |
Wood worker’s lung | Contaminated wood pulp or dust | Alternaria spp Wood dust |
Hardwood worker’s lung | Kiln-dried wood | Paecilomyces |
Wood trimmer’s lung | Contaminated wood trimmings | Rhizous spp Mucor spp |
Dry rot lung | Rotten wood | Merulius lacrimans |
Familial HP | Contaminated wood dust in walls | Bacillus subtilis |
Paprika slicer’s lung | Moldy paprika | Mucor stolonifer |
Stipatosis | Esparto dust | Thermoactinomyces actinomycetes Aspergillus fumigatus Pseudomonas spp |
Basement shower HP | Mold or unventilated shower | Epicoccum nigrum |
Basement lung | Contaminated basement | Cephalosporium spp Penicillium spp |
Wind instrument lung | Contaminated saxophones, trombones | Mold Bacteria |
Steam iron HP | Contaminated water reservoir | Sphingobacterium spiritivorum |
Summer-type HP | Japanese house dust | Trichosporon cutaneum |
Lycoperdonosis | Lycoperdon puffballs | Puffball spores |
Chiropodist’s lung | Foot skin and nail dust | Fungi |
ANIMAL PROTEINS | ||
Pigeon-breeder’s disease Bird fancier’s lung | Pigeons, parakeets, parrots, doves, cockatiels | Avian proteins from bird excreta, feathers or bloom |
Feather duvet lung | Feather beds, pillows, duvets | Avian proteins |
Laboratory worker’s lung Animal handler’s lung | Rat or gerbil urine | Rodent urinary, serum, pelt protein |
Furrier’s lung | Animal pelts | Animal fur dust |
Miller’s lung Wheat weevil disease | Infested wheat flour | Sitophilus granaries |
Pearl oyster shellfish HP | Oyster/mollusk shell protein | Pearl oyster proteins. shell dust |
Mollusk shellfish HP | Sea snail shell dust | Sea snail shell proteins |
Silk producer HP | Dust from silkworm larvae and cocoons | Silkworm proteins |
Pituitary snuff taker’s lung | Bovine and porcine pituitary powder | Pituitary proteins, snuff |
Fish meal worker’s lung | Fish meal dust | Fish meal |
Bat lung | Bat droppings | Bat serum proteins |
CHEMICAL AND DRUGS | ||
Chemical worker’s lung | Polyurethane foams, paints, elastomers, glues | Diisocyanates Isocyanate Trimellitic anhydride |
Epoxy resin lung | Heated epoxy resin | Phthalic anhydride |
Dental technician’s lung | Polishing and grinding prostheses | Methyl methacrylate |
Paint refinisher’s disease, Bathtub refinisher’s lung | Varnishes, lacquer, foundry casting, polyurethane foam | Toluene diisocyanate |
Pauli’s reagent alveolitis | Laboratory reagent | Sodium diazobenzene sulfate |
Pyrethrum lung | Insecticide | Pyrethrum |
Bible printer’s lung | Moldy typesetting water | Unknown |
Coptic lung | Cloth wrapping of mummies | Unknown |
Grain measurer’s lung | Cereal grain | Unknown |
Coffee worker’s lung | Coffee bean dust | Unknown |
Drug Induced HP | Medications | Amiodarone Closporine Gold Minocycline Chlorambucil Sulfasalazine Nitrofurantoin Methotrexate Beta blockers Mesalamine |
The first example of HP described was farmer’s lung due to a thermophilic organism. These are unicellular branching organisms that resemble true bacteria. A previously common occupational form of HP was bagassosis or Louisiana sugarcane workers’ disease. Mold can also be a cause of HP, with two examples being maple bark strippers disease, developing in loggers who strip the bark and are exposed to Cryptostroma corticale underneath the bark and malt workers lung, developing in brewery workers exposed to Aspergillus clavatus present in the moldy barley on brewery floors. Probably the most common cause of HP today is avian antigen. Birds are becoming an increasingly popular pet and serve as a potent source of antigens responsible for HP. Finally, an example of a chemical source is toluene diisocyanate, which may cause disease in bathtub refinishers.
Bird Fancier’s Lung
Avian antigens are the most commonly reported cause of HP in children. Proteins derived from the bloom, serum, or excrement of several avian species have been demonstrated to cause HP. Bloom, a dust coating the feathers composed of keratin covered with IgA, is produced in large quantities by flying birds. While pigeons are the principal source of avian antigen in HP, several other birds have been implicated, such as parakeets, parrots, doves, and cockatiels. Although bird fancier’s lung is the commonest form of HP seen in children, it is still a rare disease. Most pediatric reports note that children were initially treated for pulmonary infections or had multiple health care encounters before a diagnosis was made. The diagnosis should be considered in any child with persistent, unexplained respiratory symptoms. The mainstay of therapy is bird avoidance, and therapy with corticosteroids is frequently required. Importantly, bird antigens have been found to persist at high levels as long as 18 months after bird elimination from the home. As is the case with other forms of HP, patients with acute forms of the disease have the best prognosis, while those with chronic disease have higher morbidity.
Other Environmental Exposures
HP may also result from exposure to various fungal or bacterial antigens. Thermophilic actinomycetes, Neurospora, and Candida albicans have been reported as causes of HP in patients that used covered swimming pools. Residential mold contamination with Aureobasidium pullulans has been reported to cause HP in an adult and in child siblings. This same species has been implicated as the etiologic agent causing HP in a child exposed to indoor hydroponics. Household exposure to a fungus, Trichosporon cutaneum, causes summer-type HP, a common form of HP in Japan. Contamination of the home with the fungus Bjerkandera adusta was found to cause HP in an elderly male. A report of two children with HP secondary to exposure from an unventilated basement shower identified Epicoccum nigrum as the causative mold. Fusarium napiforme was also found to be the etiologic agent in a 17-year-old with HP due to residential mold exposure. Exposure to mold or various bacteria through ultrasonic humidifiers and saunas has also been demonstrated to cause HP. Aspergillus fumigatus was found to be the causative agent in a child with HP who had repeated exposure to organic compost on a playground. There are also numerous reports of “hot tub lung,” caused by Mycobacterium avium complex, a nontuberculous mycobacteria. This disease typically occurs after exposure to hot water aerosols from hot tubs, showers, and swimming pools. Most reports note that poor hot tub maintenance or poor personal hygiene practices contribute to the development of the disease. Some controversy does exist on whether this disease is a true representation of HP or has an infectious etiology. Patients with hot tub lung have well-formed, sometimes necrotic, granulomas, obstructive lung disease, and usually lack serum precipitating antibodies to the offending antigen, all supporting an infectious nature of the illness. However, the clinical presentation, bronchoalveolar lavage (BAL) lymphocytosis, high-resolution computed tomography (HRCT) findings, and therapeutic response to cessation of exposure and treatment with corticosteroids are consistent with HP. Cladosporium has also been implicated as a cause of HP in an enclosed hot tub area. Finally, “lifeguard lung,” a granulomatous pneumonitis from indoor swimming pool exposure, was reported in several lifeguards exposed to water spray features at an indoor pool.
Pathology/Pathogenesis
The pathogenesis of HP is incompletely understood. The initial event involves sensitization to an inhaled antigen in the distal airway, but the level and duration of antigen exposure that is required for sensitization is unknown ( Fig. 65.1 ). After antigen exposure in a sensitized individual, an acute alveolitis develops with an increase in neutrophils. This typically peaks at 48 hours and is followed by an increase in the number of macrophages and lymphocytes. Typically CD8+ T cells predominate, but CD4+ T cells can also be found distinguishing HP from sarcoidosis. In 2009, Ye et al. identified interleukin (IL) 12, IL-18, and tumor necrosis factor alpha (TNF-α) synthesis by BAL macrophages from patients with acute and chronic HP. Spontaneous and lipopolysaccharide stimulated BAL cell culture revealed higher IL-12, IL-18, and TNF-α secretion compared with controls, indicating a TH1-macrophage axis pathogenesis. Another study by Bellanger et al. revealed significantly higher IL-8, IL-6, TNFα, IL-17, and IL-23 levels in BAL fluid samples from HP patients compared with patients with other forms of interstitial lung disease.

Type III and Type IV Hypersensitivity Responses
It appears that both type III antigen-antibody complex and type IV cell-mediated responses are involved (see Fig. 65.1 ). Antigen-specific precipitating antibodies are found in patients with HP in response to the offending antigen supporting a type III reaction ; however, precipitating antibodies can also be found in exposed subjects without evidence of clinical disease. In addition, there is no immune complex deposition in the lungs. Inhaled soluble antigens can bind to immunoglobulin and cause complement activation, leading to increased vascular permeability and an influx of neutrophils and macrophages. This leads to the production of IL-1, IL-8, TNF-α, monocyte chemoattractant protein (MCP)-1, macrophage inflammatory protein (MIP)-1α, RANTES (regulated on activation, normal T cell expressed and secreted), and CCL18, with resultant migration of leukocytes into the alveolar interstitium. Several studies illustrate the role of T cell mediated immunity in the pathogenesis of HP. A variety of factors, such as MIP-1α, MCP-1, CCL18, and IL-2, lead to an influx and proliferation of lymphocytes in the lung of patients with HP. Secretion of IL-12 and MIP-1α by alveolar macrophages promotes the polarization of CD4+ Th0 lymphocytes to Th1 cells (T helper type 1), which produce interferon (IFN)-γ and are essential for granulomatous inflammation and the development of HP.
Several other factors may play a role in the pathogenesis of HP. Natural killer (NK) cells are increased in the BAL and lung tissue of patients with HP and appear to provide a protective effect. Aberrant regulatory T cell and Th17 cell function may also play a pathologic role. As mentioned previously, increased IL-17 levels have been found in the BAL fluid of HP patients compared with patients with other forms of interstitial lung disease. In a murine model of HP, long-term exposure to antigen was associated with increased collagen deposition, IL-17, and IL-1α levels. Upregulation of costimulatory molecules on alveolar macrophages and increased expression of L-, E-, and P-selectins may lead to the influx of various leukocytes into the lung. MyD88, an adapter protein that interacts with several Toll-like receptors (TLRs), has been found to play an important role in the inflammatory response seen in HP. Surfactant protein A stimulates inflammatory cytokine release and is increased in BAL fluid from patients with HP. Increased formation of free radicals, through a variety of mechanisms, also contributes to lung inflammation. Excessive accumulation of extracellular matrix components through increased levels of fibrinolysis inhibitors, such as thrombin-activatable fibrinolysis inhibitor and protein C inhibitor, or decreased activity of matrix metalloproteinases, contribute to the fibrotic process seen in chronic HP.
Multiple susceptibility factors have been implicated in the pathogenesis of HP, such as cigarette smoking, viral infections, endotoxin, and genetic predisposition. Several studies have observed a negative relationship between cigarette smoking and the development of HP in similarly exposed individuals. Many patients with HP report a viral type illness or flulike symptoms during the initial stage of disease. An acute viral infection could enhance the antigenic response in HP by increasing the ability of alveolar macrophages to present antigen, decreasing the clearance of antigens and inducing the release of proinflammatory cytokines. Endotoxin coexposure with antigen has been shown to augment lung pathology in a murine model of HP, and may act through TLRs on macrophages, leading to a Th1 T cell response, or play an indirect role in the pathogenesis of HP as an adjuvant. Finally, various HLA haplotypes and genetic polymorphisms, such as those of the TNFA promoter and IL6 gene, encoding TNF-α and IL-6, respectively, have been associated with susceptibility to HP. Familial cases of HP have also been reported.
Clinical Features
Symptoms and Physical Findings
Schuyler and Cormier proposed criteria for the diagnosis of HP ( Box 65.1 ). Major criteria included symptoms compatible with HP, evidence of antigen exposure, radiographic abnormalities consistent with HP, lymphocytosis on bronchoalveolar lavage fluid (BALF), lung biopsy demonstrating histologic changes consistent with HP, and reproduction of symptoms of HP upon antigen exposure. Minor criteria included bibasilar rales, decreased diffusion capacity for carbon monoxide (DLCO), and hypoxemia. The clinical manifestations of HP depend on the nature and circumstances of exposure and are divided into three stages: acute, subacute, and chronic.
Major Criteria (Four Major Criteria Need to Be Present)
- ▪
Symptoms compatible with HP
- ▪
Acute: High level of exposure to antigen over short period of time. Symptoms develop within 4–8 h and include fever, chills, myalgia, fatigue, dyspnea, nonproductive cough. Recovery 2–5 days after removal of exposure.
- ▪
Subacute: Low-level, intermittent, repeated exposure. Low-grade fever, progressive nonproductive cough, dyspnea over weeks-months. Recovery after removal of exposure.
- ▪
Chronic: Low-level, prolonged, and continuous exposure of antigen. Chronic nonproductive cough, dyspnea, malaise, weakness, anorexia, weight loss.
- ▪
- ▪
Evidence of exposure to antigen
- ▪
History
- ▪
IgG antibody to antigen
- ▪
- ▪
Radiographic characteristics consistent with HP
- ▪
Chest radiograph: Reticulonodular infiltrates, linear opacities
- ▪
HRCT:
- ▪
Acute: Ground-glass infiltrates
- ▪
Subacute: Micronodules, air-trapping
- ▪
Chronic: Fibrosis ± honeycombing, emphysema
- ▪
- ▪
BALF lymphocytosis
- ▪
CD8 > CD4 T cells
- ▪
Increased NK cells
- ▪
- ▪
Lung biopsy demonstrates histology consistent with HP
- ▪
Alveolitis, noncaseating granulomas, giant cells, foamy alveolar macrophages or fibrosis
- ▪
- ▪
Positive natural challenge that produces symptoms and objective abnormalities after reexposure to the offending antigen
Minor Criteria (Two Minor Criteria Need to Be Present)
- ▪
Bibasilar rales
- ▪
Decreased DLCO
- ▪
Hypoxemia, either at rest or with exertion
Clinical Prediction
- ▪
Exposure to known offending antigen
- ▪
Positive IgG antibody to offending antigen
- ▪
Recurrent episodes of symptoms
- ▪
Respiratory crackles
- ▪
Symptoms occurring between 4 and 8 h after exposure
- ▪
Weight loss
DLCO, Diffusing capacity carbon monoxide; HP, hypersensitivity pneumonitis; HRCT, high-resolution computed tomography.
Acute Stage
In the acute form such as seen in pigeon breeders, chills, fever up to 40°C, cough, and shortness of breath are seen within 4–6 hours after exposure. Symptoms may persist for up to 18 hours. Patients usually recover within 2–5 days, with episodes occurring after each subsequent exposure. Physical examination reveals only crackling rales in the lower lung fields. HP is an example of an interstitial pneumonia in which there is a disparity between the symptoms of the patient and the physical findings. Wheezing is uncommon, as most patients exposed to organic dusts do not develop HP and asthma.
Subacute Stage
This form occurs as a result of an intermittent low grade but continuous long-term exposure, such as seen in a pet-store employee. The symptoms are milder and include malaise, low-grade fever, cough, chills, and progressive dyspnea, often associated with anorexia and weight loss. This form is usually reversible.
Chronic Stage
A long-term low-grade exposure experienced by a parakeet owner or a contaminated home humidifier may result in chronic irreversible disease. These patients present with dyspnea, chronic cough, fatigue, anorexia, and weight loss.
Immunologic Studies
Laboratory evaluation generally reveals a marked leukocytosis with prominent neutrophilia during the acute phase of HP. Elevation of markers of inflammation such as erythrocyte sedimentation rate and C-reactive protein may be seen. Elevated rheumatoid factor is found in 50% of patients, along with elevation in serum IgG, IgA, and IgM. Skin testing has limited value in the workup of HP. A high percentage of patients without HP have positive skin tests reactions, and there is a lack of commercially available standardized extracts. A diagnostic hallmark of HP is an IgG precipitating antibody seen on a double gel diffusion plate ( Fig. 65.2 ). However, the presence of a precipitating antibody is only a marker of exposure and sensitization and does not correlate with disease activity. Approximately 40%–50% of asymptomatic exposed individuals will have precipitating antibody to the offending antigen. Reports from commercial laboratories are sometimes inaccurate, and the selection of a laboratory experienced in assays for the diagnosis of HP is important. Methods more sensitive than gel diffusion are available, including counterimmunoelectrophoresis (CIE), enzyme-linked immunosorbent assays (ELISA), and radioimmunoassay (RIA). Lymphocyte proliferation assays to the offending antigen are usually positive in patients with HP. In one study, alveolar macrophages from patients with HP enhanced the lymphoproliferative response, while the response was inhibited in normal patients. This suggests that a defect in the ability of alveolar macrophages to suppress the lymphoproliferative response leads to the development of the observed lymphocytic alveolitis seen in patients with HP. It should be emphasized that these studies are available only in research laboratories.

Radiologic Findings
Radiologic findings in HP correlate with stage of disease. In acute HP, chest radiography usually demonstrates poorly defined, nodular infiltrates, but patchy ground-glass opacities or diffuse infiltrates may also occur. It is important to note that patients with acute HP may have a normal chest radiograph after cessation of exposure and resolution of the acute episode. HRCT of the chest typically demonstrates ground-glass opacities in acute HP, but the presence of ground-glass opacities is generally a nonspecific finding. Ground-glass opacification represents cellular interstitial infiltration, small granulomas within the alveolar septa, or both. These opacities may be found either centrally or peripherally, but are predominantly in the lower lung zones with sparing of the apices in acute HP. In subacute HP, a reticulonodular appearance with fine linear shadows and small nodules is typically present on chest radiograph, although the chest radiograph may also be normal as seen in acute HP. The infiltrates in subacute HP usually predominate in the mid to upper lung zones. HRCT of the chest in subacute HP typically demonstrates centrilobular nodules associated with larger areas of ground-glass opacity, as well as air trapping and mosaic perfusion ( Fig. 65.3 ). Centrilobular nodules correspond to the presence of poorly marginated granulomas and active alveolitis, while mosaic perfusion indicates the redistribution of blood flow, and air trapping denotes obstructive bronchiolitis. The presence of ground-glass opacities, air trapping, mosaic perfusion, and areas of normal lung attenuation on the same film yields an appearance on HRCT known as the headcheese sign. This is characteristic for subacute HP. Chronic HP is characterized by diffuse reticulonodular infiltrates, volume loss, and coarse linear opacities on chest radiography. The findings in chronic HP appear to be more severe in the mid to upper zones of the lung. HRCT often demonstrates fibrotic changes that include irregular linear opacities, honeycombing, and traction bronchiectasis. These changes can also be found in several other disorders, such as sarcoidosis, interstitial pulmonary fibrosis, and collagen vascular diseases. Centrilobular nodules are often found in chronic HP when ongoing antigen exposure is occurring. The radiographic findings in chronic HP, unlike acute HP, are unlikely to resolve when antigen exposure ceases. There are several findings that are not characteristically found and may be helpful in differentiating HP from other disorders. Pleural effusions or pleural thickening, as well as cavitation, calcification, or atelectasis, are usually absent in HP. Hilar adenopathy, commonly seen in sarcoidosis, is rarely seen in HP.

Pulmonary Function Testing and Bronchial Challenge
Pulmonary function testing typically reveals restriction with a reduction in lung volumes and a decrease in DLCO; however, obstructive, mixed obstructive and restrictive, or normal pulmonary function tests can be seen. A decrease in airway compliance is often seen with a shift in the pressure-volume curve down and to the right. A decrease in arterial oxygen tension (PaO 2 ) on arterial blood gas analysis is most commonly seen in chronic HP, but can be found in both acute and subacute forms of the disease. The hypoxemia and decreased diffusion capacity may be accounted for by filling of the alveolar space with fluid and inflammatory cells. In a cross-sectional longitudinal study of children diagnosed with HP, the forced expiratory volume 1 second (FEV1) was abnormal in only 9.1% of patients, but DLCO and total lung capacity (TLC) were abnormal in 40.9% and 13.6%, respectively. DLCO/alveolar volume (VA) was within the normal range, and peak oxygen uptake was normal in nearly 90% of subjects, suggesting a favorable long-term prognosis.
Oxygen desaturation with exercise or with sleep is not an uncommon finding. Twenty percent to 40% display nonspecific airway reactivity, and 5%–10% have asthma. In addition, in patients with farmer’s lung, Karjalainen et al. showed an increased risk in developing asthma after their diagnosis of HP. The concomitant or future development of asthma after HP has also been shown in HP caused by diisocyanates, after summer-type HP or after residential mold exposure. Bronchial challenge has been studied in adults but is infrequently used in children. These challenges can be performed either through reexposure to the suspected setting or through a controlled challenge in an experienced laboratory. In a review by Fan, 20 of 86 children with HP underwent bronchial challenge, and only 55% of these patients had positive challenges. In a large cohort of patients with bird fancier’s lung, Morell and colleagues reported a sensitivity of 92% and specificity of 100% using bronchial challenge to avian extracts. As the authors note in their study, bronchial challenge testing should be performed in a hospital setting with experienced staff and high-quality antigenic extracts. Some difficulties that may be encountered in bronchial provocation are poor standardization of the antigen dose used for the challenge, difficulties in objectively defining a positive test, as well as the difficulty that many young children have in performing routine pulmonary function testing.
Bronchoalveolar Lavage and Lung Biopsy
The BAL from normal individuals typically reveals a preponderance of alveolar macrophages, with approximately 10% lymphocytes that have a CD4+/CD8+ ratio of 1.8. Following acute antigen exposure, patients with HP reveal a predominance of neutrophils, which is then followed by a lymphocytic alveolitis and usually comprises 60% or greater of the white blood cell differential in BAL. Historically, a low CD4+/CD8+ ratio has been associated with HP, while a CD4+/CD8+ ratio of more than 3.5 : 1 has been associated with sarcoidosis. More recent studies, however, have disputed this finding with the demonstration of HP cases with higher CD4+/CD8+ ratios. A study by Ando and colleagues also suggested that the CD4+/CD8+ ratio may differ by the causative antigen and the smoking status of the patient. The BAL findings in HP may also differ based on the age of the patient. Ratjen et al. evaluated BAL findings in children with HP. Nine subjects, aged 6–15 years, with acute HP were included in this study. The BAL uniformly revealed an increase in the percentage of lymphocytes and foamy macrophages in children with HP. All patients were found to either have an increased expression of human leukocyte antigen (HLA)-DR (7 of 8 children) on BAL lymphocytes or an increase in NK cells (5 of 8 children) in BAL fluid. Importantly, there were no significant differences between normal controls and patients with HP with respect to the CD4+/CD8+ T cell ratio. Histologic findings in HP vary based on the stage of disease. There are few reports on the pathology associated with acute HP, but most note the presence of interstitial mononuclear cell infiltrates. Granulomas and macrophages with foamy cytoplasm have also been reported. The classic triad of subacute HP includes an interstitial lymphocytic-histiocytic cell infiltrate, bronchiolitis obliterans, and scattered, poorly formed, nonnecrotizing granulomas. In a large series of patients with bird fancier’s lung, this triad was only seen in 9% of cases that underwent transbronchial lung biopsy, but at least one of the findings was present in 69% of cases. An example of a lung biopsy of an 11-year-old boy with HP due to pigeon sensitivity is shown in Fig. 65.4 . There was interstitial pneumonia with inflammation composed of predominantly lymphocytes, macrophages, and plasma cells. Macrophages with foamy cytoplasm surrounded by large numbers of mononuclear cells are unique to HP. Lymphocytes stained for CD3+ T cells and CD56+ NK cells. Typically there is a predominance of CD8+ T cells. CD20+ B cells were also in the center of a lymphoid follicle. Other findings commonly seen in HP include interstitial giant cells, interstitial noncaseating granulomas, or Schaumann bodies. In a pathologic review of 25 cases with chronic HP, giant cells, granulomas, or Schaumann bodies were seen in 88% of cases, and 72% of cases exhibited a usual interstitial pneumonia (UIP)–like pattern. A nonspecific interstitial pneumonia (NSIP)–like pattern or a bronchiolitis obliterans organizing pneumonia-like disease can also be seen in chronic HP. Multiple studies have demonstrated decreased survival rates in patients with UIP-like or NSIP-like patterns of fibrosis. The usefulness of biopsy for the diagnosis of HP has been questioned. Although the aforementioned findings are commonly seen in various stages of HP, none of the findings are pathognomonic for the disease, as they can be found in other lung disorders. Most patients with HP can be diagnosed based on signs, symptoms, exposure history, radiographic, laboratory, and BAL findings, obviating the need for lung biopsy. When a biopsy is performed, a surgical lung biopsy is the preferred method, as it has greater diagnostic yield. A study in patients with farmer’s lung found limited usefulness of transbronchial biopsies as a diagnostic tool in HP. Since the utility of transbronchial biopsies in HP is questioned and the majority of cases can be diagnosed without biopsy, surgical lung biopsy is usually reserved for cases when other studies do not yield a definitive diagnosis.

Management and Treatment
In the acute form, simple removal from the offending environment generally suffices. If symptoms are severe, the patient should be started on a tapering dose of prednisone, beginning with 60 mg per day. Supportive measures would include oxygen (O 2 ), antitussives, and antipyretics. For repeated, acute, or subacute form, exposures should be decreased as much as possible, with administration of long-term corticosteroids emphasizing alternate day therapy. The chronic form can be treated with long-term corticosteroids, but only if radiographic findings and physiologic changes indicate a response. Clinical follow-up should include spirometry with lung volume and diffusion capacity and chest radiograph. It is vital that the antigen responsible for HP be determined so that appropriate environmental control measures can be carried out. Several initiatives can decrease the antigenic burden. Chemicals can be used to prevent the growth of organisms. A good example is propionic acid, which, when added to sugarcane, eliminates the growth of the thermophilic organism responsible for bagassosis. Water should be changed frequently in humidification or air conditioning units. Storage dryers will decrease the growth of mold and thermophilic organism in hay and straw. Finally, crops should be harvested when the moisture content is low. There are many ways to decrease exposure to organic dust. Dusty materials within closed spaces should be mechanically handled. Effective ventilation will remove dust from the ambient air. In some instances, personal respirators or masks may be used. Finally, when the previous measures have failed, the worker should be removed from the disease-producing environment.
Prevention
The incidence of HP may be reduced by decreasing exposure to responsible antigens. This may be accomplished by decreasing contact with potential agents, reducing microbial contamination, or using protective equipment.
Prognosis
The prognosis for patients with HP is generally good if the offending antigen is detected and avoidance measures are enforced during the acute and subacute stages. In a review of HP in children by Fan (9), 97% of reported pediatric cases of HP had a favorable outcome when exposure was eliminated. A study in patients with farmer’s lung demonstrated a recovery in DLCO up to 2 years from initial diagnosis. In chronic HP, the prognosis is not as good, especially in patients with continued antigen exposure. The presence of fibrosis seems to be the most important factor in prognosis, as antigen class, symptom duration, and pulmonary function did not appear to be significant predictors for survival in patient with HP. Fortunately, with early identification and treatment, progression to the chronic stage of HP can be avoided.
Eosinophilic Pulmonary Diseases
Etiology
The eosinophilic lung diseases include a group of disorders that are characterized by increased peripheral blood and/or pulmonary eosinophilia. As seen in Box 65.2 , there are diverse causes of eosinophilic pulmonary diseases, including allergic bronchopulmonary aspergillosis (ABPA) and mycosis, drug and toxin induced eosinophilic disease, helminth associated eosinophilic lung disease, acute eosinophilic pneumonia, chronic eosinophilic pneumonia (CEP), eosinophilic granuloma, Churg-Strauss syndrome (CSS; eosinophilic granulomatosis with polyangiitis), and hypereosinophilic syndrome (HES). The clinical presentation of pulmonary eosinophilia consists of pulmonary symptoms, abnormal chest radiographs, and blood and sputum eosinophilia.
- 1.
Allergy bronchopulmonary aspergillosis and mycosis
- 2.
Drug and toxin induced eosinophilic disease
- 3.
Acute eosinophilic pneumonia
- 4.
Chronic eosinophilic pneumonia
- 5.
Eosinophilic granuloma (formerly Histiocytosis X)
- 6.
Helminth associated eosinophilic lung disease
- 7.
Churg-Strauss syndrome (allergic angiitis and granulomatosis)
- 8.
Hypereosinophilic syndrome
Allergic Bronchopulmonary Aspergillosis
Etiology
Hinson et al. first described ABPA in adults in 1952 in England, and Slavin et al. described the first case in a child in 1970 in the United States. ABPA is a Th2 hypersensitivity lung disease caused by bronchial colonization with Aspergillus fumigatus that affects asthmatic and cystic fibrosis (CF) subjects. As seen in Box 65.3 , ABPA is characterized by exacerbations of asthma, recurrent transient chest radiographic infiltrates, peripheral and sputum eosinophilia, IgE and IgG sensitization to A. fumigatus, and elevated total serum IgE level. A. fumigatus hyphae are generally found in the sputum at the time of acute exacerbations of ABPA. ABPA may lead to corticosteroid-dependent asthma, bronchiectasis, and/or pulmonary fibrosis. ABPA is most commonly caused by A. fumigatus; however, other fungi species such as Candida, Penicillium, and Curvularia have been identified as causing symptoms similar to ABPA. Those patients who have symptoms of ABPA with identification of a fungus other than Aspergillus fumigatus are classified with allergic bronchopulmonary mycoses or ABPM. Chowdhary et al. reviewed 143 cases worldwide of ABPM and found that Candida albicans is the most common fungi causing ABPM seen in 60% of cases. Of note, 70% of the cases from the review of ABPM did not have asthma, which is a clear distinction from ABPA. ABPA is relatively uncommon in childhood, although it has been reported in children with CF from England and the United States. A. fumigatus is a ubiquitous mold that is commonly encountered around farm buildings, barns, stables, silos, and compost heaps. Human disease has been reported from most countries of the world. ABPA should be distinguished from other lung diseases caused by A. fumigatus, such as invasive aspergillosis, aspergilloma, IgE-mediated asthma from A. fumigatus sensitivity, and HP due to A. fumigatus or A. clavus (malt worker’s disease).
a Adapted from Agarwal R, Chakrabarti A, Shah A, Gupta D, Meis JF, Guleria R, Moss R, Denning DW. ABPA complicating asthma ISHAM working group. Allergic bronchopulmonary aspergillosis: review of literature and proposal of new diagnostic and classification criteria. Clin Exp Allergy . 2013;43(8):850-873.
Minimum Criteria
Patients with asthma or cystic fibrosis
Worsening lung function not attributable to another etiology
Positive skin prick test with Aspergillus species
Total serum IgE ≥1000 ng/mL (416 IU/mL)
b Stevens DA, Moss R, Kurup VP, Knutsen AP, Greenberger P, Judson MA, Denning DW, Crameri R, Body A, Light M, Skov M, Maish W, Mastella G, and the Cystic Fibrosis Foundation Consensus Conference. Allergic bronchopulmonary aspergillosis in cystic fibrosis: Cystic Fibrosis Foundation Consensus Conference. Clin Infect Dis . 2003;37(suppl 3):S225-S264.
In CF, total serum IgE levels:
- ▪
≥1000 IU/mL, classic
- ▪
≥500 IU/mL, minimum
- ▪
200–500 IU/mL. If ABPA is suspected, repeat in 1–3 months. If patient is taking steroids, repeat when steroid treatment is discontinued.
- ▪
Increased Aspergillus species-specific IgE and IgG antibodies
New or recent abnormalities on chest radiography or chest CT that have not cleared with antibiotics and standard physiotherapy
Additional Criteria
Increase in blood eosinophilia ≥400 eosinophils/µL when patient is not on corticosteroids
Aspergillus species-specific precipitating antibodies
Central bronchiectasis
Aspergillus species-specific containing mucus plugs
Epidemiology
The prevalence of ABPA is estimated to be 1%–2% in asthmatic patients and 7%–9% (up to 15%) of patients with CF. Denning et al. estimated that the global prevalence of ABPA may be 0.7%–3.5% of patients with asthma.
Pathology/Pathogenesis
The pathology of ABPA demonstrates cylindrical bronchiectasis of the central airways, particularly the upper lobes. These airways may be occluded by “mucoid impaction,” a condition in which large airways are occluded by impacted mucus and hyphae. Airway occlusion may lead to atelectasis of a segment or lobe, and if the atelectasis is long-standing, saccular bronchiectasis may result. Typically, ABPA is worse in the upper lobes than in the lower lobes. Microscopic examination of the airways shows infiltration of the airway wall with eosinophils, lymphocytes, and plasma cells. The airway lumen may be occluded by mucus containing hyphal elements and inflammatory cells, especially eosinophils. Squamous metaplasia of the bronchial mucosa develops commonly, and sometimes granulomas form. Rarely, bronchiolitis obliterans or bronchocentric granulomatosis develops.
Biology of Eosinophils
In ABPA and the other eosinophilic pulmonary diseases discussed later in this chapter, eosinophilic inflammation is prominent. In the pathogenesis of ABPA, there is extensive eosinophilic infiltration and inflammation in the bronchial airways and in the lung parenchyma ( Fig. 65.5 ). Furthermore, there is extensive degranulation of eosinophil mediators, such as major basic protein (MBP) in the lung interstitium. Eosinophils are inflammatory granulocytes important in host defense principally against helminth parasites. They also play an important inflammatory role in a variety of diseases, including asthma, allergic diseases, eosinophilic esophagitis, and HESs. Eosinophils are bone-marrow derived granulocytes. The key cytokines that are critical for bone production of eosinophils are IL-3, IL-5, and granulocyte-monocyte-colony stimulating factor (GM-CSF), produced by CD4+ T cells. The activation of eosinophils is principally by IL-5 stimulation, which also increases tissue eosinophil survival. Tissue recruitment of eosinophils from the vascular system is stimulated by the chemokines platelet activating factor (PAF), leukotriene (LT)-D4, C5a, CCL11/eotaxin, and CCL5/RANTES (regulated on activation normal T cells expressed and secreted). Stimulation by eotaxin is selective for only eosinophils.

Eosinophils possess a number of preformed granule-derived proteins (MBP, eosinophil peroxidase, eosinophil cationic protein, eosinophil-derived neurotoxin, and Charcot-Leyden crystal protein), de novo synthesized lipid mediators (LTC4, PGE1, PGE2, TXB2, 15-HETE, and PAF), and reactive oxygen species that are released upon stimulation and result in inflammation. Eosinophils synthesize a number of Th2 cytokines (IL-4 and IL-5), Th1 cytokines (IFN-γ), and chemokines (eotaxin, RANTES). Eosinophils also have Fc receptors for principally IgA, which may regulate antibody-dependent cellular cytotoxicity (ADCC) eosinophil degranulation. Thus eosinophils are principally Th2-driven granulocytes that have potent inflammatory and tissue destructive properties.
In the pathogenesis of ABPA, A. fumigatus spores 3–5 µm in size are inhaled and germinate into hyphae deep within the bronchi ( Fig. 65.6 ). In addition, fragments of the hyphae can be identified within the interstitium of the pulmonary parenchyma (see Fig. 65.6 ). The implication is that there is the potential for high concentrations of A. fumigatus allergens to be exposed to the respiratory epithelium and immune system. A. fumigatus releases a variety of proteins, including superoxide dismutases, catalases, proteases, ribotoxin, phospholipases, hemolysin, gliotoxin, phthioic acid, and other toxins. The first line of defense against Aspergillus colonization in the lungs is macrophage and neutrophil killing of the conidia and the hyphae. In the development of ABPA, Kauffman’s group proposed that Aspergillus proteins have a direct effect on the pulmonary epithelia and macrophage inflammation. They demonstrated that Aspergillus proteases induce epithelial cell detachment. In addition, protease-containing culture filtrates of Aspergillus induce human bronchial cell lines to produce proinflammatory chemokines and cytokines, such as IL-8, IL-6, and MCP-1. Thus various Aspergillus proteins have significant biologic activity that disrupts the epithelial integrity and induces a monokine inflammatory response. This protease activity allows for enhanced allergen exposure to the bronchoalveolar lymphoid tissue immune system. This is evident by the bronchoalveolar lymphoid tissue synthesis of Aspergillus -specific IgE and IgA antibodies.

Genetic susceptibility factors have been proposed in the development of ABPA ( Box 65.4 ). Chauhan and colleagues observed that asthmatic and CF patients who expressed HLA-DR2 and/or DR5 and lacked HLA-DQ2 were at increased risk to develop ABPA after exposure to A. fumigatus. Furthermore, within HLA-DR2 and HLA-DR5, there are restricted genotypes. In particular, HLA-DRB1*1501 and HLA-DRB1*1503 were reported to produce high relative risk. On the other hand, 40%–44% of non-ABPA atopic Aspergillus -sensitive individuals have the HLA-DR2 and/or HLA-DR5 genotype. Additional studies indicated that the presence of HLA-DQ2 (especially HLA-DQB1*0201 ) provided protection from the development of ABPA. Recently, increased sensitivity to in vitro IL-4 stimulation, as measured by enhanced expression of the low-affinity IgE receptor (CD23) on B cells, was observed in ABPA patients. This was associated with single-nucleotide polymorphisms of the IL-4 receptor alpha chain (IL4RA) in 92% of ABPA subjects, principally the IL-4-binding single-nucleotide polymorphism ile75val.
HLA-DR restriction and HLA-DQ protection
HLA-DR2: HLA-DRB1*1501 and *HLA-DRB1*1503
HLA-DR5: HLA-DRB1*1101 and HLA-DRGB1*1104
HLA-DQ2 protective, decreased in ABPA
DQB1*0201
IL-4RA polymorphisms
IL-4RA ile75val
IL-10 polymorphisms
Promoter – 1082 GG genotype
Surfactant protein A2 (SP-A2) polymorphisms
SP-A2 ala91pro
Cystic fibrosis transmembrane conductance regulator (CFTR) mutations
Heterozygous CFTR mutations in asthmatic patients with ABPA
Toll-like receptor (TLR) polymorphisms
TLR9 T-1237C
HLA, Human leukocyte antigen; IL, interleukin.
This increased sensitivity to IL-4 is demonstrated by increased expression of CD23 and CD86 on B cells of ABPA subjects and increased CD23 expression during flares of ABPA. CD23 is expressed on a variety of cells, including B cells, NK cells, subpopulations of T cells, and a subpopulation of dendritic cells. T-cell CD23 and B-cell CD21 form a costimulatory pathway. T-cell CD28 and B-cells CD80 and CD86 costimulatory pathways activate both T and B cells, and CD28:CD86 is important in IgE synthesis. CD86 is also found on dendritic cells that have the histamine receptor 2, which skews antigen-specific T cells to a Th2 response. In a murine model of ABPA, Kurup and colleagues have found that CD86 expression is upregulated in the lung tissue (V.P. Kurup, Medical College of Wisconsin, personal communication). Recently, we have also observed increased CD86 expression on monocyte-derived dendritic cells of ABPA subjects. Thus antigen-presenting cells such as monocytes and dendritic cells bearing HLA-DR2 and/or HLA-DR5 and increased sensitivity to IL-4 stimulation probably play a critical role in skewing A. fumigatus -specific Th2 responses in ABPA.
Brouard and coworkers recently reported a third genetic risk, the association of the – 1082GG genotype of the IL-10 promoter with colonization with A. fumigatus and the development of ABPA in CF. The -1082GG polymorphism has been associated with increased IL-10 synthesis, whereas the -1082A allele has lower IL-10 synthesis. Thus dendritic cells expressing HLA-DR2/DR5 that have an HR2 phenotype, increased IL-10 synthesis, and increased sensitivity to IL-4 stimulation due to IL-4RA polymorphisms may be responsible for skewing Aspergillus- specific Th2 responses in ABPA.
Studies in asthma have implicated the role of bronchial epithelia and mesenchymal cells forming the epithelial-mesenchymal trophic unit (EMTU) with a profibrotic response when stimulated with proteases such as Der p1 and with IL-4 and IL-13. In ABPA subjects, Aspergillus proteases and allergen stimulation of the EMTU in conjunction with increased sensitivity to IL-4 due IL-4RA SNPs may result in increased bronchial epithelial secretion of IL-8, GM-CSF, and transforming growth factor (TGF-α), the ligand for epidermal growth factor leading to bronchial destruction and fibrosis.
Clinical Features
Symptoms and Physical Findings
Clinical symptoms of ABPA include increased coughing, episodes of wheezing, anorexia, malaise, fever, and expectoration of brown plugs (see Box 65.3 ). ABPA can present acutely with acute symptoms and signs associated with transient pulmonary infiltrates and eosinophilia or with mucoid impaction; it may also present an exacerbation of a chronic disease characterized by proximal bronchiectasis. It is thought that the chronic form of the disease develops following the acute process and that it can be prevented by effective therapy. In chronic ABPA, the acute episodes are superimposed on a background of chronic cough and sputum production. In adults, ABPA usually affects the younger age group of adult asthmatics, and most cases occur before 40 years of age. In pediatrics, ABPA rarely affects children with asthma, and it is usually seen in children with CF, who may simply appear to have a worsening of their pulmonary status or an acute pulmonary exacerbation of CF. ABPA does sometimes affect children with asthma, and there is a report of three children who developed it before 2 years of age. Physical examination shows the signs of chronic lung disease from CF or asthma, such as hyperaeration of the lungs, expiratory wheezing, a chronic productive cough, and crackles or wheezes. The chronically ill patient with bronchiectasis may have coarse crackles, weight loss, and digital clubbing.
The criteria for the diagnosis of ABPA are shown in Box 65.5 . The minimal criteria are worsening of lung function not attributed to another cause in asthmatic or CF patients, new pulmonary infiltrates and/or high-attenuation mucus (HAM) impaction, IgE to A. fumigatus demonstrated by either allergy prick skin test or in vitro specific IgE to A. fumigatus, elevated IgG to A. fumigatus demonstrated either by Aspergillus specific precipitins or in vitro IgG to A. fumigatus, elevated serum IgE ≥1000 ng/ml (≥416 IU/ml), and blood eosinophilia ≥400/mL. When central bronchiectasis is also present, ABPA is classified as ABPA central bronchiectasis (ABPA-CB) and when absent classified as ABPA serologic (ABPA-S). In CF, the Cystic Fibrosis Foundation Consensus Conference recommended different criteria for total serum IgE levels. In the diagnosis of ABPA in CF, elevated serum IgE was defined as a level ≥1000 IU/ml. Minimum IgE level was defined as ≥500 IU/mL. If ABPA is suspected and the total level is 200–500 IU/mL, it is recommended to repeat testing in 1–3 months. A problem with applying the criteria in children is that usually ABPA occurs in children with CF in whom many of the criteria could be due to the underlying disease. Some children with CF appear to have a clinical variant of ABPA without having all the typical criteria, and they may respond clinically to corticosteroids. Patients being considered for this diagnosis should have skin testing with A. fumigatus antigen.
- 1.
Transpulmonary passage of helminth larvae (Loeffler’s syndrome)
- ▪
Ascaris lumbricoides, Ancylostoma duodenale, Necator americanus, Strongyloides stercoralis
- ▪
- 2.
Hematogenous seeding with helminth larvae
- ▪
Cutaneous larva migrans—nonhuman ascarids and hookworms, Trichinella spiralis, Schistosoma
- ▪
Visceral larva migrans—aberrant infection— Toxocara canis
- ▪
Disseminated Strongyloides
- ▪
- 3.
Pulmonary parenchymal invasion with helminthes
- ▪
Parogonimus westermani lung flukes
- ▪
- 4.
Tropical pulmonary eosinophilia—Filaria
- ▪
Wucheria bancrofti, Brugia malayi
- ▪
Aspergillosis in Cystic Fibrosis
In 2013, Denning’s group proposed a novel immunologic classification of aspergillosis in adult CF patients. Four classification of aspergillosis were identified by examinations of sputum Aspergillus colonization using RT-PCR and Aspergillus hyphae by galactomannan (GM), total IgE level, and Aspergillus specific IgE and IgG level. Class 1 (nondisease) represented ± RT-PCR, negative GM, negative IgE, and IgG Aspergillus serology; class 2 (serologic ABPA), positive RT-PCR, positive GM, elevated total IgE, and Aspergillus specific IgE/IgG; and class 3 ( Aspergillus sensitized), ± RT-PCR, negative GM, elevated Aspergillus IgE, but not Aspergillus IgG; and class 4 ( Aspergillus bronchitis), positive RT-PCR, positive GM, elevated Aspergillus IgG, but not IgE.
In 2016, Mirković et al. reported using Aspergillus stimulated basophil activation test (BAT) measuring surface marker CD203c expression to identify ABPA in CF patients. Three groups were identified. In Group 1 (nonsensitized), decreased BAT CD203c, decreased total IgE, and decreased Aspergillus specific IgE; Group 2 ( Aspergillus sensitized), decreased BAT CD203c, decreased total IgE, and decreased Aspergillus specific IgE; and Group 3 (ABPA), elevated BAT CD203c, elevated total IgE, and elevated Aspergillus specific IgE.
Clinical Staging
The spectrum of ABPA varies widely, from individuals with mild asthma and occasional episodes of pulmonary eosinophilia (with no long-term sequelae), to patients with fibrosis, honeycomb lung, and respiratory failure. Patterson and colleagues have suggested a clinical classification with five clinical stages, as shown in Table 65.2 . Stage I is the acute stage of ABPA, with many of the typical features of the disease. If this stage goes into remission, the infiltrates clear, symptoms reduce, and the serum IgE value will decline by up to 35% within 6 weeks. Stage II is remission. Stage III is an exacerbation associated with the recurrence of the initial symptoms and a twofold increase in serum IgE level. Stage IV is reached when patients need continuous corticosteroids either to control their asthma or to prevent a recurrence of ABPA. Stage V is the fibrotic stage, which is present when there is severe upper lobe fibrosis present on the chest radiograph, and it may be associated with honeycombing. The stage V lesions may not respond to corticosteroids, although steroids are often necessary to maintain a bronchodilator response, and severe wheezing may develop if steroids are discontinued. Pulmonary fibrosis is an advanced complication that can lead to pulmonary hypertension and cor pulmonale.
Stage | IgE Level | Precipitins | Eosinophilia | IgE-Af | IgG-Af | Pulmonary Infiltrates |
---|---|---|---|---|---|---|
(I) Acute | +++ | + | + | + | + | + |
(II) Remission | + | ± | — | ± | ± | — |
(III) Exacerbation | +++ | + | + | + | + | + |
(IV) Corticosteroid-dependent | ++ | ± | ± | ± | ± | — |
(V) Fibrotic | + | ± | — | ± | ± | — |
Radiographic Findings
There are several characteristic radiographic abnormalities associated with ABPA. The most common lesion is a large, homogeneous shadow in one of the upper lobes with no change in volume. The shadow may be triangular, lobar, or patchy, and it frequently moves to another site. “Tram line” shadows are fine parallel lines radiating from the hila that represent inflammation of airway walls. Mucoid impaction causes toothpaste shadows or gloved-finger shadows. Several adult patients have been reported with normal chest radiographs, so radiographic abnormalities are not invariably present. In these individuals, cylindrical bronchiectasis was demonstrated by high-resolution computerized tomography (HRCT) scan ( Fig. 65.7 ).

HAM has been proposed by Agarwal et al. to be evaluated with HRCT. Based on multiple studies, Agarwal et al. found that the presence of HAM is pathognomonic of ABPA. They have also investigated the immunologic severity of these patients with HAM. They, along with International Society for Human and Animal Mycology (ISHAM), have proposed a change in severity and classification based on radiographic studies as follows: mild ABPA-serologic (ABPA-S), moderate ABPA-central bronchiectasis (ABPA-CB), and severe ABPA-CB-HAM. In a study of 234 patients, Agarwal et al. found 25% normal HRCT, 76.5% with CB, 20.9% with HAM, and 11.5% with other radiologic findings. It was noted that patients with CB or HAM had frequent relapses; however, those with HAM had significantly elevated immunologic markers compared with the other groups, which was correlated with more severe cases of ABPA. Of note, failure to achieve remission was associated with CB, not with HAM. HAM has been shown to be 100% specific in the diagnosis of ABPA.
Laboratory Findings
Laboratory tests that support the diagnosis of ABPA are those that demonstrate allergy to the mold, such as a positive specific IgE test and positive Aspergillus precipitins. The precipitins are only weakly positive compared with the strong reactions seen in patients with mycetomas. Culture of A. fumigatus from the sputum is only a secondary criterion for the diagnosis of ABPA, because a large proportion of individuals with CF without ABPA have Aspergillus on sputum cultures. Some normal individuals and many individuals with lung diseases have small numbers of spores in their sputum; these are probably present because of passive inhalation. The presence of hyphae is more specific, and the presence of eosinophils in association with hyphal elements is suggestive of the diagnosis. The presence of eosinophilia in sputum or blood is suggestive of ABPA and is a primary diagnostic criterion. The peripheral blood eosinophil count is usually greater than 1000/mm 3 , and values greater than 3000/mm 3 are common.
An increased serum IgE value is very characteristic of ABPA, and values may reach as high as 30,000 IU/mL. Usually, the value is greater than 1000 IU/mL. Much of the IgE is not specific to Aspergillus but is the result of polyclonal B-cell activation. The IgE level is a very useful marker of disease activity, and it can be used to follow outpatients for “flares.” The simple skin-prick test is a useful screening test, as ABPA is very unlikely in patients with a negative reaction. A dual-reaction of intradermal Aspergillus skin test with an immediate (10–15 minutes) and a late (4–8 hours) reaction occurs in one-third of patients with ABPA. Alternatively, serum may be measured for the presence of specific IgE and IgG antibodies. Patients with ABPA and Aspergillus -sensitive asthma will have Aspergillus specific IgE antibody, but patients with ABPA will have quantitatively increased Aspergillus -specific IgE levels. Crameri’s group has reported that ABPA and Aspergillus -sensitive patients have elevated IgE antibodies to recombinant Aspergillus Asp f1, Asp f3, Asp f4, and Asp f6 allergens, and that IgE levels to Asp f4 and Asp f6 are highly specific for ABPA.
The usual pattern of serum precipitins is that immunoelectrophoresis shows one to three precipitin lines, often to only one extract. Patients with aspergilloma will have multiple precipitin lines to all antigen extracts. Extracts of A. fumigatus contain a complex mixture of proteins that are mainly derived from the hyphae. Antigenic composition varies between batches according to the culture conditions, even within the same laboratory. Thus there is a lack of standardization that makes it difficult to compare results between laboratories. However, there has been some success with purification of the major antigenic components that may lead eventually to improved diagnosis. We find it best to send all our testing to one central laboratory that has well-established methods for the characterization of the serologic responses to A. fumigatus.
Differential Diagnosis
Several diseases cause pulmonary infiltrates in children with asthma or CF. The differential diagnosis of ABPA should include the following: viral or bacterial pneumonia, poorly controlled asthma with mucoid impaction or atelectasis, inhaled foreign body, CF (with or without ABPA), immotile cilia syndrome, tuberculosis with eosinophilia, sarcoidosis, pulmonary infiltrates with eosinophilia, HP, and pulmonary neoplasm.
Management and Treatment
Treatment is designed first to control the acute episodes and then to limit the development of chronic lung disease. Most cases of ABPA require treatment with systemic corticosteroids, and the treatment of choice is prednisone. Steroid therapy rapidly clears the eosinophilic infiltrates and the associated symptoms, although it is less effective at treating mucus impaction. The usual starting dose is 0.5 mg/kg per day, taken each morning, and this dose is maintained for 2–4 weeks while following the patient clinically and checking the chest radiograph for resolution of the acute process. After this induction treatment, the dose of prednisone should be reduced to 0.5 mg/kg given on alternate days. If mucus impaction persists and is associated with atelectasis, bronchoscopy should be performed to confirm the diagnosis and to attempt to remove the mucus plugs.
Following resolution of the acute process, the dose of prednisone should be reduced over 1–3 months. Chronic treatment with corticosteroids is controversial, especially in adults, because a minority of patients with ABPA are at risk of chronic lung disease. The relationship between acute episodes and lung damage is unclear, and the precise dose of prednisone is not certain, since acute exacerbations may continue while the patients are on low doses of steroids. However, children with ABPA usually have CF and may need treatment with long-term corticosteroids to prevent progressive lung damage. Therefore we usually maintain therapy with a dose of 0.5 mg/kg on alternate days for 3 months, and then, after 3 months, the dose of prednisone is tapered over an additional 3 months while checking the chest radiograph and the serum IgE level for evidence of relapse. Initially the serum IgE level should be checked at every visit and, if the level increases by twofold or more, the steroid dose should be increased. We recommend that patients are followed with serum IgE levels and chest radiographs every 6 months for the first 1–2 years, and then, if the patient remains in remission, it should be possible to reduce the frequency of these studies.
The antifungal agent itraconazole has been used to reduce the doses of steroids that are required. Initially there were only open nonrandomized studies indicating that itraconazole is a useful adjunct to systemic corticosteroid therapy. Two recent randomized controlled trials have also favored itraconazole use. A double-blind, randomized, placebo-controlled trial of itraconazole 200 mg twice daily dose resulted in decreased IgE levels and an increase in pulmonary function and exercise tolerance. Another randomized, controlled trial showed that treatment of stable ABPA in adults with 400 mg/day itraconazole resulted in a significant reduction in sputum eosinophil count, sputum eosinophilic cationic protein levels, serum IgE concentrations, and Aspergillus -specific IgG. There was also a reduction in episodes of exacerbation, requiring treatment with systemic steroids. In the treatment of children with ABPA, we have used a dose of 10 mg/kg per day of itraconazole.
Omalizumab, a humanized monoclonal antibody that binds free IgE, has been used in patients with refractory ABPA. Current dosing of omalizumab is based on total serum IgE levels. However, patients with ABPA can have IgE levels that far exceed the upper limit of omalizumab dosing, as part of their disease process. Therefore they should be treated with the maximum recommended weight-based dose of omalizumab. Collins et al. performed a retrospective chart review of 21 patients who had received omalizumab for refractory ABPA. This group of patients overall had decrease in steroid use, decrease in levels of total serum IgE, and improved symptoms which were noted with an increase in Asthma Control Test (ACT) scores. In France, Tillie-Leblond et al. evaluated 16 patients with ABPA who received omalizumab for 1 year. This group of patients had decreased exacerbations and the need for oral steroids during this time. In 2016, Voskamp et al. reported a randomized, double-blind, placebo-controlled, cross-over trial with 2 treatment phases of 4-month duration. Thirteen patients with asthma and ABPA were identified and underwent the active treatment phase with omalizumab 375 mg every 2 weeks for 4 months with a washout period of 3 months, and crossover arm. ABPA exacerbations occurred less frequently during omalizumab treatment compared with the placebo period, 2 versus 12 events. Mean fractional exhaled nitric oxide (FeNO) decreased from 30.5 to 17.1 ppb during omalizumab treatment. In addition, omalizumab treatment resulted in decreased basophil reactivity to A. fumigatus and FcεR1 and surface-bound IgE levels. These recent studies and many case reports show the benefit this additional treatment option offers patients with ABPA.
Recent studies have shown utility in inhaled amphotericin B to provide localized treatment of ABPA and thereby decrease side effects. In addition, hypertonic saline has been used to help clear mucus from the airways in patients with bronchiectasis, though patients must be monitored carefully with their first dose, as it can induce bronchoconstriction. Macrolide antibiotics have been used for antiinflammatory effects in patients with bronchiectasis, though no studies have been done in patients with ABPA or to compare macrolide versus itraconazole therapy.
There is no place for immunotherapy in children with ABPA, since it is ineffective and potentially dangerous. Inhaled antiinflammatory agents, such as cromoglycate and beclomethasone, are not generally thought to be effective. The role of inhaled spores in the pathogenesis of ABPA is unclear, but there is a seasonal incidence of ABPA that is probably related to seasonal changes in mold spore counts. Therefore it is reasonable to advise patients with ABPA to avoid exposure to places with high spore counts, such as damp basements, barns, and compost heaps.
Prognosis
The prognosis for children with ABPA is good if the disease is detected early and treatment started promptly. It is important that the diagnosis is made and treatment commenced before there is permanent lung damage from bronchiectasis. In such patients, there should be no progression of the disease, although relapses can occur many years later, and long-term follow-up is recommended. In patients with CF, the relapses seem to be more frequent than in asthma, and careful surveillance is necessary to ensure resolution of the disease process. In some CF patients, it is difficult to wean the steroids without an increase in symptoms, such as dyspnea and wheezing; whether this is due to the underlying CF lung disease or due to patients going from stage II to stage III ABPA on withdrawal of steroids is unclear. Symptoms are not a reliable guide to therapy; therefore it is important to reevaluate the chest radiograph and the serum IgE at regular intervals until a long-term remission is established.
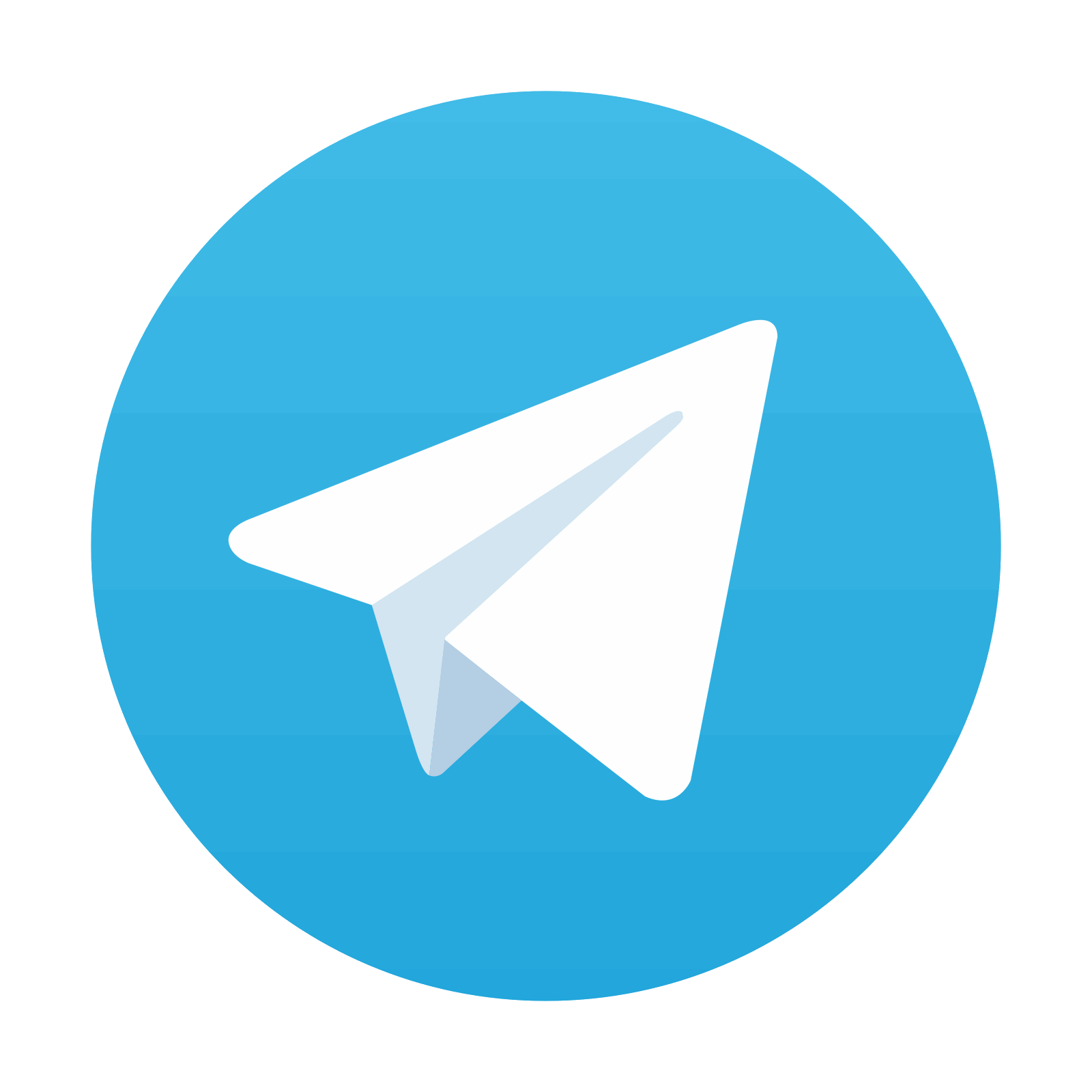
Stay updated, free articles. Join our Telegram channel

Full access? Get Clinical Tree
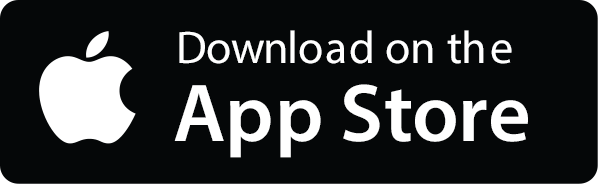
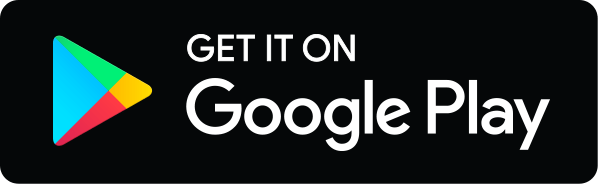