Hemostasis is the physiologic response to bleeding that occurs when a vessel wall is disrupted and is regulated through several processes. In the arterial hemostatic system, the first response is the activation, adhesion, and aggregation of platelets. The platelet plug that forms is stabilized by the deposition of fibrin, which is generated on the surface of the platelet membrane and on nearby disrupted vessel surfaces. The extent of thrombosis is limited by endogenous anticoagulant systems and by the fibrinolytic system, which preclude the development of thrombosis beyond the site of injury.1
Thrombosis is a pathologic process characterized by an inappropriate or exaggerated generation of clot. It may occur if there is vessel wall injury without disruption (i.e., no bleeding), or in the setting of excess activation of platelets or coagulation factors. Inadequate endogenous anticoagulant or fibrinolytic function may also contribute to the development of thrombosis.
In the arterial circulation, hemostasis and thrombosis are platelet dependent, where high shear stresses and potentially turbulent flow stimulate this mechanism and where the fibrinolytic system regulates secondary fibrin formation.2,3 By contrast, the venous system is characterized by low flow and stasis, permitting the development of hemostasis and thrombosis through activation of the coagulation cascade and regulation by endogenous anticoagulant proteins.1 This distinction is important when considering defined hypercoagulable states and the role they may play in peripheral arterial disease.
The initiation of hemostasis or thrombosis in the arterial system is dependent on the platelet, with stabilization of the platelet plug with fibrin. The basic pathway and contributing factors are depicted in Figure 10-1.
FIGURE 10-1.
Basic steps in arterial hemostasis. vWF, von Willebrand factor; PAF, platelet activation factor; TSP, thrombospondin; TBX A2, thromboxane A2; PAI-1, plasminogen activator inhibitor-1; TAFI, thrombin-activated fibrinolytic inhibitor; TFPI, tissue factor pathway inhibitor; tPA, tissue plaminogen activator; uPA, urokinase-like plaminogen activator.

Under normal conditions, platelets do not adhere to the normal endothelial surface of the arterial wall. If there is a disruption of the endothelial surface, platelets will spontaneously adhere to the exposed subendothelial matrix, medial wall, or adventitial layers. This adhesion is mediated by integrin receptors on the platelet surface, including glycoprotein (GP) Ia/IIa, which binds to collagen.2,3 Collagen may be the most important component of the subendothelial matrix for the initiation of platelet adhesion.4 von Willebrand factor (vWF) is also embedded in the subendothelial matrix,5 which is preferentially recognized over circulating plasma vWF by GP Ib/IX, another key integrin receptor on the platelet surface, creating platelet adhesion.2,5
Disruption of the smooth endothelial surface creates turbulent flow and, if the vessel becomes narrowed by vasospasm or irregularities on the endovascular surface, shear stress on the platelet may increase. Platelet binding to subendothelial matrix appears to be significantly augmented at higher sheer stress.6 Platelet receptor–ligand interactions result not only in platelet adhesion but also in platelet activation. When activated, intracellular signaling results in activation of GP IIb/IIIa receptors on the platelet surface.7 Multiple ligands bind to this receptor, including vWF, fibrinogen, fibronectin, vitronectin, and other proteins with the amino acid sequence arginine-glycine-aspartate (RGD) present in subendothelial matrix and augment platelet adhesion.8 Platelets taken from patients with peripheral arterial disease demonstrate increased adhesivity in vitro under flow conditions.9
Adhesion of platelets induces platelet activation, which results flattening and pseudopod extension of the platelet membrane.10 Activated platelets also release platelet aggregation agents from storage pool granules, including adenosine diphosphate (ADP), fibrinogen, collagen, fibronectin, thrombospondin, epinephrine, platelet-activation factor (PAF), serotonin, and vWF.2,3,10 Thromboxane A2 production and release are stimulated.1,10 Each of these factors promotes platelet aggregation. P-selectin is found on the membrane of alpha granules and is rapidly elaborated on the platelet surface when platelets are activated, which serves as a receptor for white cell adhesion.11 Increased platelet activation, indicated by P-selectin expression, platelet fibrinogen binding, and activated microparticle formation, has been demonstrated in peripheral vascular disease.12,13
With the release of potent mediators, platelet aggregation rapidly follows platelet adhesion and activation, covering the exposed subendothelial surface or area of vessel injury with a platelete-rich thrombus. Collagen, fibrinogen, and vWF mediate platelet aggregation by serving as bridging ligands between platelets via GP IIb/IIIa receptors.7,14 Thrombin is a potent platelet agonist, causing both platelet activation and aggregation.15 However, during arterial hemostasis and thrombosis, platelet adhesion, aggregation, and accumulation occur within milliseconds, leading some authors to argue that there is insufficient time for thrombin formation to play a significant role in the initial formation of the platelet-rich thrombus.2,16 In experimental models, however, platelet deposition in the thrombus formation has been attenuated by treatment with direct thrombin inhibitors.3,17 Initiation of arterial thrombosis may thus be dependent on small amounts of thrombin as a platelet agonist for hemostasis. Pathological platelet-dependent thrombosis may occur more readily in the setting of arterial vascular disease, where stenosis may increase shear stresses and atherosclerotic plaque rupture releases adhesive, activating, and aggregatory platelet ligands.18 Increased spontaneous platelet aggregation has been demonstrated in blood taken from patients with peripheral artery disease as compare to controls.9,19
Histopathological and experimental observations demonstrate that formation of a fibrin-rich thrombus is an important second phase of arterial hemostasis and thrombosis.4 Activated platelets more readily bind fibrin and fibrinogen through GPIIb/IIIa receptors.7,14 In arterial thrombosis, fibrin generation occurs on the phospholipid membrane of platelets, the site of activation and effective propagation of thrombin generation.1
When activated, the phospholipid component of the platelet membrane is altered, and phosphotidylserine flipped to the external surface, which promotes and supports coagulation.20 Initiation of the coagulation cascade on these procoagulant surfaces begins when tissue factor (TF) binds to factor VIIa.21 There are several potential sources for TF in arterial hemostasis and thrombosis, some of which are dependent on the presence of activated platelets. Damage to the endothelial surface of the vessel may expose flowing blood to the smooth muscle cells in the media. TF is elaborated by these cells when stimulated by agonists such as platelet-derived growth factor, which is stored in platelet granules and released with platelet activation.22 With deeper vessel wall injury, the adventitial layer is exposed, where TF is present in normal vessels.23 Increased elaboration of TF by the smooth muscle cells and monocytes/macrophages involved in the development of the atherosclerotic plaque, especially when stimulated by inflammatory markers, likely enhance the risk for thrombosis in peripheral arterial disease.3
TF/activated factor VII (factor VIIa) complexes form on procoagulant surfaces (platelet membrane, media, and adventitia), initiating coagulation by the extrinsic pathway21 (Figure 10-2). TF/VIIa complexes in turn activate factor X. Factor V, released from platelet granules, and factor VIII are activated by thrombin. Factor Xa/Va complexes form and convert prothrombin into thrombin. The generation of thrombin results in a multitude of effects, including recruitment and activation of additional platelets.24 Feedback activation of factor Va and VIIIa by thrombin contributes to the sustained and amplified activation of the coagulation cascade through the intrinsic pathway and to further thrombin generation.25 Thrombin cleaves fibrinogen into fibrin and activates factor XIII, which cross-links and stabilizes the fibrin mesh. In arterial hemostasis, the generation of thrombin is thought to occur predominately on the phospholipid surface of the accumulated platelets and is highly dependent on the presence of platelet deposition.1,24,26
FIGURE 10-2.
Simplified coagulation cascade. Hemostasis is initiated by tissue factor (TF)/factor VIIa complexes through the extrinsic pathway, which generates factor Xa. Factor Xa/Va complexes convert prothrombin (factor II) into thrombin (IIa). Thrombin has multiple effects, including platelet aggregation, fibrin formation, and inhibition of fibrinolysis. Thrombin also provides feedback amplification of the coagulation cascade through the intrinsic pathway, activating factors Va and VIIIa, which contribute to further generation of factor Xa.

Hemostasis is regulated by the fibrinolytic system, which is activated when plasminogen binds to the fibrin present in a thrombus. Endothelial cells secrete plasminogen activators when stimulated by the fibrin, thrombin, and by the altered flow or absence of flow created by the thrombus.27 Tissue plaminogen activator (tPA) binds to fibrin and to the plasminogen bound there, cleaving the plasminogen to plasmin.28 Plasmin lyses both the cross-linked and uncross-linked fibrin to which it is bound.27,28 This process opens additional binding sites for plamsinogen, extending thrombolysis. Plasmin also cleaves tPA into a two-chain molecule that has a greater affinity for fibrinogen, which further limits the thrombus propagation by reducing the amount of fibrinogen locally available for clot building.27,28 Adjacent to the area of injury and thrombus, intact endothelial cells with surface receptors for tPA, urokinase-like plasminogen activator (uPA), and plasminogen facilitate the generation of plasmin and limit the propagation of thrombus beyond the area of injury.29
Excess fibrinolysis at the site of injury is regulated by fibrinolytic inhibitors. Major vascular physiologic inhibitors of fibrinolysis include α2-antiplasmin and plasminogen activator inhibitor-1 (PAI-1).27,28,29 α2-Antiplasmin is a plasma protein that binds and inhibits plasminogen, reducing the amount of substrate available for plasmin generation. PAI-1 binds and inhibits tPA and uPA, which reduces the activation of plasminogen to plasmin. PAI-1 is made in endothelial cells, smooth muscle cells, adipose tissue, hepatocytes, and platelets.30 PAI-1 is released from platelet granules during platelet aggregation31 and is present in the subendothelial matrix,32 which limits fibrinolytic activity at the site of injury, permitting stabilization of the thrombus.
The overall net effect of the fibrinolytic system in response to vascular injury is to limit thrombolysis at the site of injury (subendothelial matrix) but facilitate thrombolysis to limit the extent of thrombosis at the surface of the clot (on fibrin) and on the surface of intact endothelial cells adjacent to the site of injury.
The inadequate release of fibrinolytic activators or excessive release of fibrinolytic inhibitors can result in reduced fibrinolytic activity and permit the pathologic propagation of thrombosis.27 Some reports note an increase in tPA antigen in plasma33 and vessel walls,34 which is thought to represent a compensatory physiological activation of the fibrinolytic system in response to the pathologic changes in atherosclerosis. However, significant reductions in circulating tPA activity and increased activity of PAI-1 have been reported in peripheral arterial disease, resulting in a loss of fibrinolytic potential activity33 and diminished capacity to limit thrombus propagation.
Regulation of thrombin generation is mediated by endogenous anticoagulant proteins, including tissue factor pathway inhibitor (TFPI), antithrombin (AT, formerly known as antithrombin III), protein C, and protein S. TFPI is produced by endothelial cells and binds to factor Xa.35 TFPI/Xa complexes then bind with TF/VIIa complexes, forming a quaternary structure that renders all these factors inactive, which inhibits the extrinsic pathway of coagulation necessary for initiation of thrombosis.35 Antithrombin binds and inactivates activated clotting factors, especially factors Xa and IIa (thrombin), in the presence of glycosaminoglycans on the surface of the endothelium.36 Protein C is activated by thrombin/thrombomodulin complexes on the surface of endothelial cells.37 Activated protein C (aPC) then binds to protein S on a phospholipid surface; this aPC/PS complex inactivates factors Va and VIIIa.37 Resistance to the activity of aPC, most commonly conferred by the presence of the factor V Leiden (FVL) mutation, results in a modest reduction of endogenous anticoagulant activity.38
Although abnormalities in these endogenous anticoagulant pathways may limit thrombin generation and fibrin deposition, there is little consistent evidence that deficiencies of or resistance to these anticoagulant factors increases the risk of arterial thrombosis.2
The balance of pro- and antithombotic factors in normal hemostasis is heavily influenced by the vascular endothelium. The vascular endothelium produces and releases antithrombotic factors, including prostacyclin, nitric oxide, tissue plasminogen activator (tPA), and TFPI.39 Elaboration of surface-bound factors, including thrombomodulin, heparin sulfate proteogycans, and ecto-ADPase, enhances endogenous anticoagulant activity and further limit unwanted thrombosis.39 Processes intrinsic to peripheral arterial disease significantly alter these regulatory factors and create and increase potential for thrombosis.
Blood flow through normal arteries is laminar, applying minimal shear stress to platelets as well as to the endothelial surface. In contrast, arteriovascular disease, especially atherosclerosis, creates vessel wall abnormalities and endovascular remodeling creating abnormal turbulent flow40 through stenotic and irregular areas that may increase platelet activation, adhesion, and aggregation. The correlation demonstrated between peripheral arterial disease severity and markers of platelet activation and aggregation12,13,19 supports the concept that disturbed flow may contribute to platelet abnormalities. Correlation between platelet microparticle formation with ankle brachial index41 and evidence of increased platelet activation in the common femoral vein in proportion to degree of stenosis by angiography42 suggest abnormal vasculature contributes to platelet abnormalities. Other reports note that while markers of platelet activation are elevated in peripheral artery disease, there is limited correlation with the severity of disease.43 The diminished capacity of platelets from patients with very severe peripheral arterial disease to be activated in vitro12,44 suggests that perhaps platelets may be affected and ultimately “exhausted” by in the atherosclerotic circulation.
In addition to effects on platelets, disturbed flow and shear forces may activate or damage endothelial cells.45,46 Initially, healthy endothelial cells respond to shear stress by increasing synthesis of nitric oxide and prostacyclin, which inhibit platelet aggregation39 and upregulate TFPI,39 functionally reducing available TF.47 Release of tPA enhances fibrinolysis.39 However, continued shear stress may lead to endothelial injury and dysfunction, resulting in loss of these antithrombotic properties, upregulation of TF,48 adhesion molecules,49 release of vWF,50 and PAI-1.39 Attenuation of vasodilation caused by decreased production of nitric oxide by endothelial cells may further compromise blood flow, though most of observations of this phenomenon have been made in coronary arteries.51
Atherosclerosis in peripheral arterial disease involves several processes, including inflammation and oxidative injury.51 In addition to the shear stress created by endovascular remodeling, the inflammatory response to atherosclerotic plaque development leads to endothelial dysfunction, abnormal subendothelial matrix, and smooth muscle proliferation and migration.51 Endothelial stimulation by cytokines leads to increased expression of adhesion molecules, including P-selectin52 and release of vWF.39 Altered or decreased expression of surface receptors for plasmin(ogen), tPA, and uPA53,54 related to these processes may limit fibrinolytic capacity. Functional impairment of the fibrinolytic system, with impaired release of tPA, has been demonstrated in various disease states, including hypertension, chronic kidney disease,55 and increased PAI capacity noted in patients with arterial vascular disease.56 High levels of plasma lipoproteins, especially very low density lipoprotein, modulate endothelial production and release of tPA and PAI-1,57 and increased fibrinogen deposition has been noted in arterial segments from patients undergoing arterial surgery.58 Lipoprotein(a) is composed of a low-density lipoprotein particle linked to apliliprotein B-100, which is highly homologous to plasminogen and inhibits fibrinolysis through plasminogen competition.59 Inflammatory mediators downregulate concentrations of heparin-like molecules and the expression of thrombomodulin and endothelial protein C receptor on the endothelial cell surface, diminishing endogenous regulation of thrombin.54 Decreased activity of the thrombomodulin/protein C system has been associated with increased fibrin deposition on cell surfaces.60 The net effect of these inflammatory, oxidative, and lipid-mediated changes is a loss of fibrinolytic capacity with increased fibrin deposition.
If the normal vascular endothelium is denuded, normal subendothelial matrix containing vonWillebrand factor, collagen, vitronectin, and PAI-1 is exposed to flowing blood, promoting platelet adhesion and thrombosis.61 The source of these factors is the medial smooth muscle cell, which is activated by injury and growth factors released in the process of atherogenesis.34 During atherogenesis, smooth muscle cells increase production and elaboration of extracellular matrix proteins,51 which promote platelet adhesion and aggregation. These cells also elaborate increased amounts of TF62 and PAI-1 in excess of tPA.30,34 In advanced atherosclerosis, all of these factors are concentrated in the atherosclerotic plaque, which can rapidly promote local thrombosis in the event of plaque rupture.18
The impact of the vascular changes induced by atherosclerosis would be predicted to produce measurable changes in hemostatic factors. Table 10-1 lists changes in hemostatic factors that have been demonstrated in patients with peripheral arterial disease (see also Refs. 9,12,13,19,33,34,35,37,42,43,44,56,58,63,64,65,66,67,68,69,70,71,72,73,74,75,76,77.) As noted in Table 10-1, several changes are independently characteristics of patients with peripheral arterial disease. However, there is often a significant interaction between these hemostatic changes, traditional vascular risk factors, and inflammation.65,68 For example, factor VII activity is correlated with lipid levels45 and markers of inflammation,68 but is not consistently independently associated with peripheral arterial disease.67,71 Claudicants with diabetes have significantly higher levels of vWF, fibrinogen, and thrombin–antithrombin complexes, as compared to nondiabetic cluadicants; coronary and cerebrovascular diseases have also been found to be more common in these patients.78 Thus, both the metabolic changes of diabetes and the presence of other affected vascular bed may impact hemostatic factors. The fibrinolytic system in particular may be differentially affected by diabetes.79 Factor VIII and vWF are released from damage endothelium, often in response to inflammatory mediators.39,49,53 Since most data are collected from patients with known peripheral arterial disease, which can induce the changes in the coagulation system, it is not clear whether the hemostatic changes are a cause or a consequence of the vascular injury underlying the disease process.
Hemostatic Factor | Change | Correlation with Disease Severity | References |
---|---|---|---|
Platelet function P-selectin | Increased expression on intact platelet | Yes/no | 12,43,63 |
Increased expression on platelet microparticles | ND | 13 | |
Decreased quantity in alpha granules | Yes | 12 | |
Platelet aggregation | Spontaneous aggregation in vitro | Yes | 9,19 |
Presence of microaggregates | No | 41,44 | |
CD40-L | Increased levels of soluble CD40L | Yes | 43 |
Procoagulant factors von Willebrand factor | Increased plasma levels of antigen and activity | Yes | 63,64,65,66,67,68 |
Factor VII | Increased, correlated with triglycerides | ND | 65 |
Increased, correlated with markers of inflammation | ND | 68 | |
Factor VIII | Increased antigen, activity if history of myocardial infarction | ND | 64,65 |
Increased, correlated with fibrinogen | ND | 65 | |
Fibrinogen | Increased plasma levels of antigen and activity | Yes | 9,58,63,66,67,69,70 |
Increased if history of myocardial infarction | ND | 64,65 | |
Increased, correlated with markers of inflammation | Yes | 68,69 | |
Does not predict for vascular complications | ND | 69,71 | |
Fibrinolytic factors Tissue plaminogen activator (tPA) | Increased antigen | Yes | 37 |
Decreased activity | Yes | 33 | |
Diminished expression in vessel walls | ND | 58 | |
Increased expression in vessel walls in advanced disease | Yes | 34 | |
Plasminogen activator inhibitor-1 (PAI-1) | Increased activity | No | 33,67,72 |
Increased antigen, predicts for vascular complications | ND | 69,71,73 | |
Increased PAI-1 capacity | No | 56 | |
Diminished expression in vessel walls | No | 58 | |
Increased expression in vessel walls | Yes | 34 | |
Decreased PAI-1/tPA ratio | Yes | 63 | |
A2-antiplasmin | Increased antigen if cerebral vascular disease | Yes | 64 |
Markers of coagulation activation D-dimer | Increased | Yes | 9,56,70,72,73,74 |
Increased if history of cardiac or cerebrovascular events | Yes | 69 | |
Increased in arterial but not venous samples | ND | 75 | |
Increased, may predict thrombotic events | ND | 71 | |
Prothrombin fragment 1 + 2 | Increased if history of cardiac or cerebrovascular events | Yes | 69 |
Increased, correlated with D-dimer | No | 74 | |
Thrombin—antithrombin | Increased | ND | 9,56,73 |
complexes (TAT) | Increased, correlated with D-dimer | ND | 74 |
Increased, predictive of vascular events | ND | 71 | |
Whole blood clotting | Hypercoagulable changes by thromboelastography (TEG) | ND | 42 |
Shortened endotoxin-induced recalcification time | ND | 77 | |
Endogenous anticoagulants Antithrombin (III) | Modest decreased activity | Yes | 63 |
Protein C | Decreased activity levels | ND | 73 |
Levels correlated with factor VII | ND | 65 | |
Thrombomodulin | Increased soluble TM plasma levels | ND | 72 |
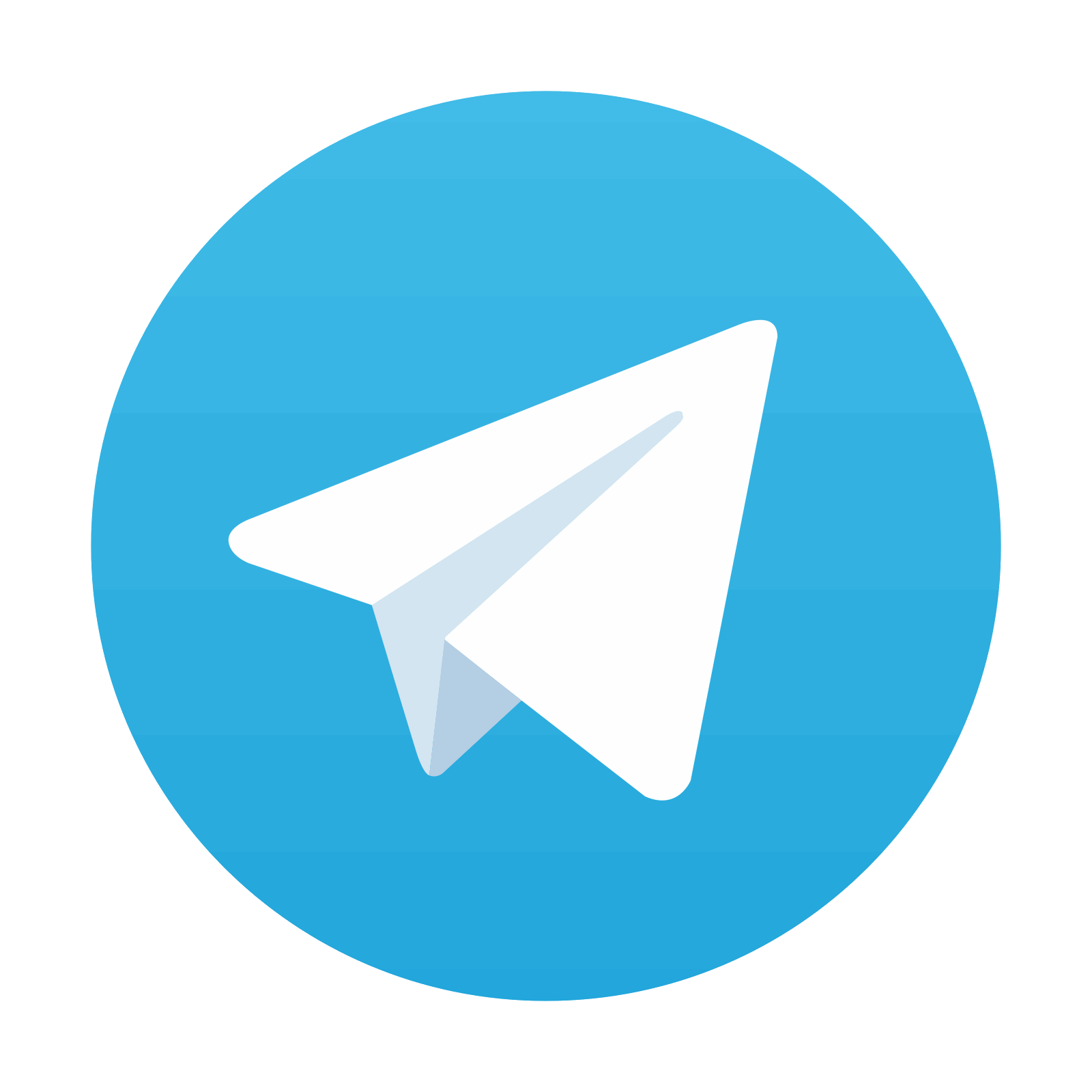
Stay updated, free articles. Join our Telegram channel

Full access? Get Clinical Tree
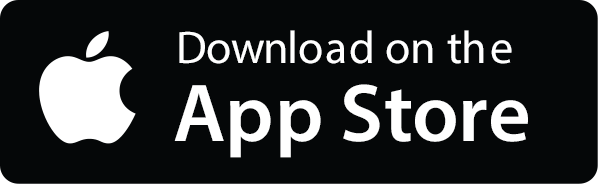
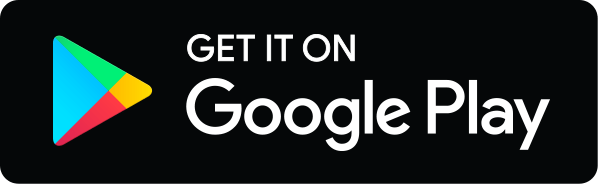
