Despite the established clinical and survival benefits of cardiac resynchronization therapy (CRT) in advanced heart failure, 20% to 30% of patients in randomized trials have been “nonresponders.” The definition of this term is still under debate; experts disagree as to whether clinical parameters (symptoms and exercise capacity) and/or echocardiographic parameters (ejection fraction, mitral regurgitation [MR], ventricular dimensions and volumes) adequately define a “CRT responder.” Further complicating the characterization of CRT response is the repeated lack of concordance between echocardiographic and clinical parameters after implantation.
Resynchronization of myocardial contraction occurs at three levels: (1) from the atria to the ventricles, or atrioventricular (AV) resynchronization; (2) interventricular (VV), between the right and left ventricles; and (3) intraventricular, among the different left ventricular (LV) segments. Although the physiology is unclear, emerging evidence regarding dyssynchrony indicates the importance of individually modifying pacing intervals as opposed to “out of the box” settings and suggests that resynchronization occurs at these three levels. Numerous techniques have been studied, predominantly focusing on echocardiographic parameters, to determine optimal AV and VV duration to enhance the effectiveness of CRT. Many trials have shown acute benefits after identifying optimal pacing intervals, but whether this translates into improved clinical outcomes in the long term has yet to be firmly established, primarily regarding VV optimization.
In this issue of JASE , Taha et al. describe a comprehensive echocardiographically guided optimization technique that is all inclusive in terms of assessing multiple systolic and diastolic echocardiographic parameters, as opposed to the more widespread practice of following one or two of these parameters during the optimization procedure. They conclude that such a comprehensive Doppler echocardiographic approach performed for every tested AV and VV interval improves functional status and that acoustic cardiography, with reduction in S 3 intensity, serves as an additive component for this protocol. Before commenting on their approach, it is imperative to review the different methods of echocardiographic optimization, including advantages and disadvantages.
Methods for CRT Optimization
The foundation of individually adjusting AV delays in biventricular pacing lies in the suboptimal LV filling noted with shortened or prolonged AV intervals. A prolonged AV interval results in delayed onset of early or passive LV filling (represented by the E wave on mitral inflow Doppler tracings), which therefore coincides with the atrial contribution to filling later in diastole (represented by the A wave on mitral inflow Doppler tracing); subsequent delay in LV contraction occurs in conjunction with incomplete mitral valve closure and diastolic (or presystolic) MR.
Conversely, with a shortened AV interval, premature mitral valve closure results in reduced LV filling because of the truncation of the atrial contribution to filling. Therefore, “optimal” AV delay improves LV filling without compromising the atrial contribution and has been found to correlate with immediate improvement in hemodynamics, defined as a rise in aortic pulse pressure and the maximum rate of LV dP/dt. The premise behind sequential biventricular pacing is to compensate for suboptimal LV lead position or correct for a mixture of different ventricular activation patterns commonly found in patients with LV dysfunction or scarred myocardium, thereby optimizing VV synchronization.
A wide variety of echocardiographic techniques for optimization have been described, with a plausible rationale supporting implementation; the majority for evaluating the AV delay focus on diastolic filling patterns. The Ritter technique, mentioned in Taha et al. ’s protocol, synchronizes the end of the atrial contraction with the onset of ventricular systole by evaluating the delay from the QRS onset to the end of the a wave using both a short and long AV delay. The short delay must force premature closure of the mitral valve, while the long AV delay does not allow intrinsic conduction. This difference is used to calculate the optimal AV delay. Although a simple, quick method for calculating this delay, the Ritter method was initially designed for use in dual-chamber pacing and AV block; application to dilated cardiomyopathy with elevated filling pressures and biventricular pacing is uncertain. The simplified mitral inflow method is even more straightforward than the Ritter method, using a single prolonged AV delay; the optimal delay is based on subtracting the time delay from the end of the A wave to the onset of MR from the tested prolonged delay (defined as the maximal AV delay with preserved biventricular pacing shortened by 5–10 msec). Limitations include the dependence on some degree of MR and the uncertain assumption that varying AV delays does not affect the timing of MR onset.
Diastolic filling time has also been used for optimization and is represented by the longest EA duration that does not compromise atrial contribution. The mitral inflow velocity-time integral (VTI) is another possibility (with stable mitral valve area) using pulsed-wave Doppler to measure maximal EA VTI. With the latter two techniques, concerns about reproducibility and averaging measurements for multiple beats at each delay have been raised. Finally, the iterative method was used in the Cardiac Resynchronization Therapy in Heart Failure (CARE-HF) study ; it is based on separating the E and A waves while termination of the A wave occurs with mitral valve closure, thereby avoiding A-wave truncation and premature mitral valve closure.
Difficulty discerning the end of the A wave is a shortcoming for many of these diastolic parameters for optimization. A different approach therefore focuses on echocardiographic changes in systolic performance measures, using VTI as a surrogate for stroke volume and cardiac output and defining the optimal pacing delay by the maximal aortic VTI for a given interval obtained by continuous-wave Doppler in the apical five-chamber view. Maximal aortic VTI has been used to identify the most favorable AV delay or VV delay, supporting sequential over simultaneous biventricular pacing. Multiple drawbacks exist in echocardiographic optimization, including (1) operator dependency, (2) a time-consuming process, (3) maintaining stable positioning of the transducer in the mitral inflow or aortic outflow tracts for each tested pacing interval, (4) beat-to-beat variation in the VTI, (5) the effect of loading conditions on measured LV filling patterns, (6) reproducibility and intrinsic variability in measured data, (7) uncertain stability over time, and (8) the effect of body position and activity or exercise, as the procedure is performed in a resting, supine state.
Because of such limitations, the focus for CRT optimization has moved beyond echocardiography. Device-based algorithms, such as Expert Ease for Heart Failure (Boston Scientific Corporation, Natick, MA) and QuickOpt (St. Jude Medical, St. Paul, MN), center on intrinsic PR/QRS duration and intracardiac electrograms, respectively. Noninvasive options include fast ventricular depolarization on surface electrocardiography, finger plethysmography (using conventional pulse oximetry probes to find the maximal pulse pressure), and indirect measurement of cardiac output (i.e., impedance cardiography, which estimates stroke volume through changes in thoracic impedance).
Acoustic cardiography (Audicor; Inovise, Portland, OR) combines phonocardiography with surface electrocardiography to measure diastolic heart sounds with the S 3 and S 4 strength and electromechanical activation time (the time delay from QRS onset to the mitral valve component of the first heart sound); invasive data have shown correlations with LV function and LV end-diastolic pressure. For CRT optimization, acoustic cardiographic results have been similar to those of echocardiography and produced significant clinical and hemodynamic improvement versus default settings in a pilot study.
A Comprehensive Echocardiographic Optimization Approach
The technique described by Taha et al. addresses a major criticism of optimization, particularly in echocardiography, in which the actual significance of measured data and the precision of the estimated optimal AV and VV delays, perhaps the most overlooked aspect of the procedure, are unknown. They describe a comprehensive echocardiographic optimization protocol that encompasses a broad range of diastolic and systolic parameters, as opposed to the traditional approach of evaluating one or two of these variables. The authors assessed maximal LV outflow and mitral inflow VTI, LV ejection time, LV filling time, MR (both systolic and diastolic), systolic pulmonary artery pressure, maximal E-wave VTI, myocardial performance index, and deceleration time, averaging 5 to 10 beats for each data acquisition on the basis of the patient’s rhythm. Taha et al. must be commended on an approach that, in theory, should improve the precision of echocardiographic optimization through the assessment of such a wide range of hemodynamic parameters, especially when rhythm issues or previous mitral valve surgery limit the interpretation of certain parameters.
Although their protocol guides a more detailed optimization, a major criticism involves determining which of the multiple collected variables has more impact on final selection. Taha et al. state, “In some patients, mitral filling time had to be compromised to minimize pulmonary vein flow reversal, while in others, the mitral inflow A wave had to be truncated to avoid diastolic MR,” but the final interpretation of which variable is most essential to “optimize” and which should be “compromised” is operator dependent. Perhaps a different interpreter would select another delay without a clearly defined algorithm, making the decision more subjective. For example, with acoustic cardiography, an algorithm exists with the goal of reducing S 3 strength to a value < 5.0 for optimal programming delays; if S 3 strength is <5 across tested intervals, the most favorable AV and VV delays are defined by a reduction in electromechanical activation time and/or increase in LV systolic time.
Likewise, the authors’ use of the Ritter method for AV optimization contributes to a vulnerability of their protocol. Their description of the AV optimization procedure raises an important point about the multiple modalities available for adjusting AV intervals. Taha et al. mention using the Ritter method to determine optimal AV delay and, if not feasible, the iterative method. They also describe acquiring the previously mentioned multiple systolic and diastolic parameters to select optimal delays, but it is unclear exactly what range of AV delays were evaluated for these parameters. Were these data points acquired for only a few intervals surrounding the optimal delay noted by the Ritter or iterative method (i.e., from 160 to 200 ms for an optimal AV delay by the Ritter method of 180 ms) or for a wider range of delays that would be assessed in traditional optimization procedures until loss of biventricular capture (i.e., from 80 to 300 ms in 20-ms increments)? If the former scenario applies, limitations then exist with this protocol, as Jansen et al. showed that the Ritter method fared the worst of four echocardiographic parameters in terms of correlation with the optimal AV delay as documented by LV dP/dt max . In fact, optimal AV delay by the Ritter method did not correlate with invasive measures in any patient compared with aortic VTI, mitral EA duration, and maximal mitral EA VTI. As previously mentioned, a major criticism of the Ritter method is its questionable application in the clinical scenario of cardiomyopathy with large ventricular volumes and elevated filling pressures.
A major vulnerability of CRT optimization lies in the assumption that data acquired across a wide range of AV and VV delays is precise and significantly different when selecting final programming. Currently, a broad range of reasonable optimization methods have been described in the literature, but we cannot be certain of the accuracy of measured data during CRT optimization: if repeating the procedure with the same parameter (i.e., maximal aortic VTI) will yield similar results and if temporal change in the optimal delays signifies CRT response or statistical variability. These questions remain important when selecting final AV and VV delays: intrinsic variability in the acquired data may lead to spurious findings, and programming to such delays could adversely affect cardiac function. To reduce error, data are usually recorded after a brief time delay between programming changes to allow an equilibrium period, over one to two respiratory cycles, averaging data for a few beats, and excluding premature and postpremature beats. In a small study of 20 successive patients referred for optimization, Turcott et al. found that commonly used echocardiographic methods (specifically aortic VTI, mitral VTI, A-wave truncation, and septal-posterior wall motion delay) did not generate significant differences in data across tested AV and VV delays in the majority of patients (62%–82%) versus impedance cardiography. These authors suggested that testing current optimization techniques for statistical significance could help narrow the wide range of available options until randomized clinical studies demonstrate definitive benefit in terms of clinical outcomes.
Taha et al. found an acute significant difference among most systolic and diastolic Doppler echocardiographic parameters after optimization compared with baseline and expanded clinically on this finding through correlation with 1-month outcomes, including improved New York Heart Association (NYHA) class and a trend toward improved quality of life ( p = .08). It is important to note that in their study, actual clinical improvement occurred in a small number of patients, taking into account that 50% had mild symptoms (22 of 44 patients in NYHA class I or II at baseline vs 27 at follow-up). Of the 22 with more advanced symptoms (NYHA class III or IV) prior to optimization, eight remained advanced and two either died or underwent transplantation, but follow-up data were not available in seven patients overall. Medical therapy was suboptimal in terms of angiotensin-converting enzyme inhibitor use (only 55% at baseline), but follow-up changes in drug therapy were not available in the study to determine any effect on functional status.
Through the inclusion of follow-up data, Taha et al. ’s study design addresses one of the most important factors limiting optimization today: few studies have evaluated the clinical impact of this procedure. Reverse remodeling from simultaneous CRT is well established through landmark clinical trials, and small studies have found further acute improvement in LV ejection fraction or dyssynchrony with sequential biventricular pacing. However, acute improvement does not necessarily translate into long-term clinical benefit. Three studies addressing clinical outcomes after VV optimization found no difference in NYHA class, 6-minute walking distance, or quality of life when comparing standard (simultaneous) with sequential biventricular pacing ; benefit was noted in both groups without an additional improvement from VV optimization. The InSync III Marquis study was a larger trial of 359 patients, in which significant incremental improvements in exercise capacity were found in patients with optimized sequential biventricular pacing after 6 months. Shorter follow-up of 3 to 6 months is a plausible explanation for the lack of additional benefit in the aforementioned studies; in another small study ( n = 37) evaluating long-term variation in pacing delays, reverse remodeling was evident after 12 months of optimized pacing but not at 6 months. From CARE-HF, reverse remodeling appears to be a time-dependent process, on the basis of greater neurohormonal response at 18 months than at 3 months. Regardless, questions remain as to whether biventricular pacing at optimal delays translates into clinical outcomes, although it does not appear to have an adverse effect.
Taha et al. enrolled patients in all functional classes of heart failure, including 50% of patients with mild symptoms and minimal exercise intolerance (NYHA classes I and II; refer to Table 2 in their report). Overall, the study population was not as advanced as typical patients referred for optimization procedures; in most studies, the majority have been nonresponders. The authors conclude that pacemaker optimization should be offered to most CRT patients on the basis of the immediate significant improvement in hemodynamic parameters in both NYHA class I or II and class III or IV groups (refer to Table 3 in their report). As stated, intrinsic variability and reproducibility of Doppler echocardiographic parameters limit the interpretation of this finding without correlation to clinical outcomes. Both NYHA class and quality of life are subjective indicators and prone to the placebo effect. Furthermore, half the enrolled patients had mild symptoms at enrollment, and recent clinical evidence has shown the potential for reverse remodeling with “preventive” CRT devices implanted earlier in the course of heart failure, indicating that such an extensive optimization procedure in milder stages of heart failure is likely premature and unnecessary. Further support for optimizing nonresponders only as opposed to all implanted patients was seen in the recently presented Frequent Optimization Study Using the QuickOpt Method (FREEDOM) clinical trial; routine and/or frequent device-based optimization of AV and VV intervals did not contribute significantly to further improving responsiveness to CRT when initiated within weeks of implantation in patients with standard CRT indications. For this reason, some experts suggest that a practical approach is to ensure adequate transmitral inflow on baseline echocardiography at the time of implantation (perform AV delay modification in the presence of A wave truncation) while reserving a comprehensive AV and VV optimization for nonresponders in follow-up. The Response of Cardiac Resynchronization Therapy Optimization With V-V Timing in Heart Failure Patients (RESPONSE-HF) trial used this approach, with AV optimization after implantation or enrollment and VV optimization performed 3 months later in nonresponders. Sequential biventricular pacing significantly increased clinical parameters in CRT responders (NYHA class) but not objective measures (LV ejection fraction, 6-minute walking distance) compared with simultaneous pacing. Optimal pacing involved stimulating the left ventricle first in 75% of patients, and no adverse clinical effects were noted with optimization. Of note, the nonresponse rate was lower than expected at 3 months, 15% compared with estimates of 30% to 40% in the literature, suggesting an important role for AV optimization in CRT response.
Temporal variation in pacing delays and the appropriate sequence of optimization are other unknowns when performing CRT optimization. As the left ventricle remodels, one would expect some degree of change in optimal AV and VV delays, which has been suggested with a trend toward longer AV delays and reduction in the LV predominance of the optimal VV delay during 9-month follow-up. Should the AV or the VV delay be adjusted first? In most centers, the sequence is AV first during simultaneous pacing, followed by VV delay assessment at the optimal AV delay. However, the appropriate sequence of AV and VV optimization has not been well studied. VV optimization has been shown to increase diastolic filling times and reduce intraventricular dyssynchrony beyond the benefit seen after AV optimization, thus illustrating the importance of ventricular resynchronization in AV mechanics and diastolic filling. Arguments to perform VV optimization before AV support an advantageous effect on both systolic and diastolic function. Further support for this sequence lies in the complexity of final programming, with two important considerations when performing AV optimization first: (1) maintain the appropriate AV delay for left-sided filling on the basis of right ventricular or LV preactivation after VV optimization, and (2) the differences in AV timing among devices (i.e., for Boston Scientific devices, AV delay is always based on right ventricular timing, whereas it is based on whichever ventricle is paced first for other devices ). When comparing the sequence of optimization to testing a full grid of AV and VV delays, Zuber et al. advised testing a full grid of different AV and VV combinations rather than optimizing one delay first; a larger study to validate results is needed to address the sequence issue in CRT optimization.
At this point, AV and VV optimization have an emerging role in enhancing CRT response. The issue is whether individually tailoring these delays makes a difference in objective measures and outcomes (ejection fraction, LV volumes, exercise capacity, heart failure morbidity), and convincing answers are lacking. Emerging studies, including that of Taha et al. , have correlated CRT optimization with clinical improvement in NYHA class. Given the time-consuming nature of the authors’ comprehensive echocardiographic approach to CRT optimization and the lack of an algorithm to determine which parameters are more significant (i.e., diastolic MR or pulmonary vein filling pattern), such a detailed protocol may be difficult to implement, even in an academic setting. The abundance of data could easily hinder final interpretation of the best pacing delays. Their approach, however, is novel and should more accurately identify optimal pacing delays in terms of hemodynamics, therefore providing a more accurate comparison for acoustic cardiography. The correlation of S 3 strength optimized delays with the comprehensive echocardiographic evaluation emphasizes the benefit of the simpler method. In this sense, their results are practical and could easily be applied to the daily practice setting. A rapid, simple method with a defined algorithm that is easily interpreted presents the most favorable solution to maximize CRT response.
Many issues remain when performing optimization, as no standard exists; the frequency, sequence, and most favorable method of optimization are still unanswered questions. Before considering a broad application of optimization in all who receive CRT devices, we need a reproducible, technically feasible approach that can be performed and interpreted in the real-world setting. The lack of supporting data precludes an extensive optimization in all CRT patients, and many centers will not have the resources for this approach. Although lasting clinical benefits are still being evaluated, optimization does not appear to impair CRT effectiveness and has an emerging role in nonresponders. Recent data suggest that a reasonable option involves the early adjustment of AV delays to ensure adequate transmitral pattern (without A-wave truncation), reserving VV optimization for nonresponders.
Dr. Hasan has received research grants from St. Jude Medical (St. Paul, MN), Biotronik GmbH (Berlin, Germany) , and CardioMEMS, Inc. (Atlanta, GA) and consultant fees from CardioMEMS, Inc.
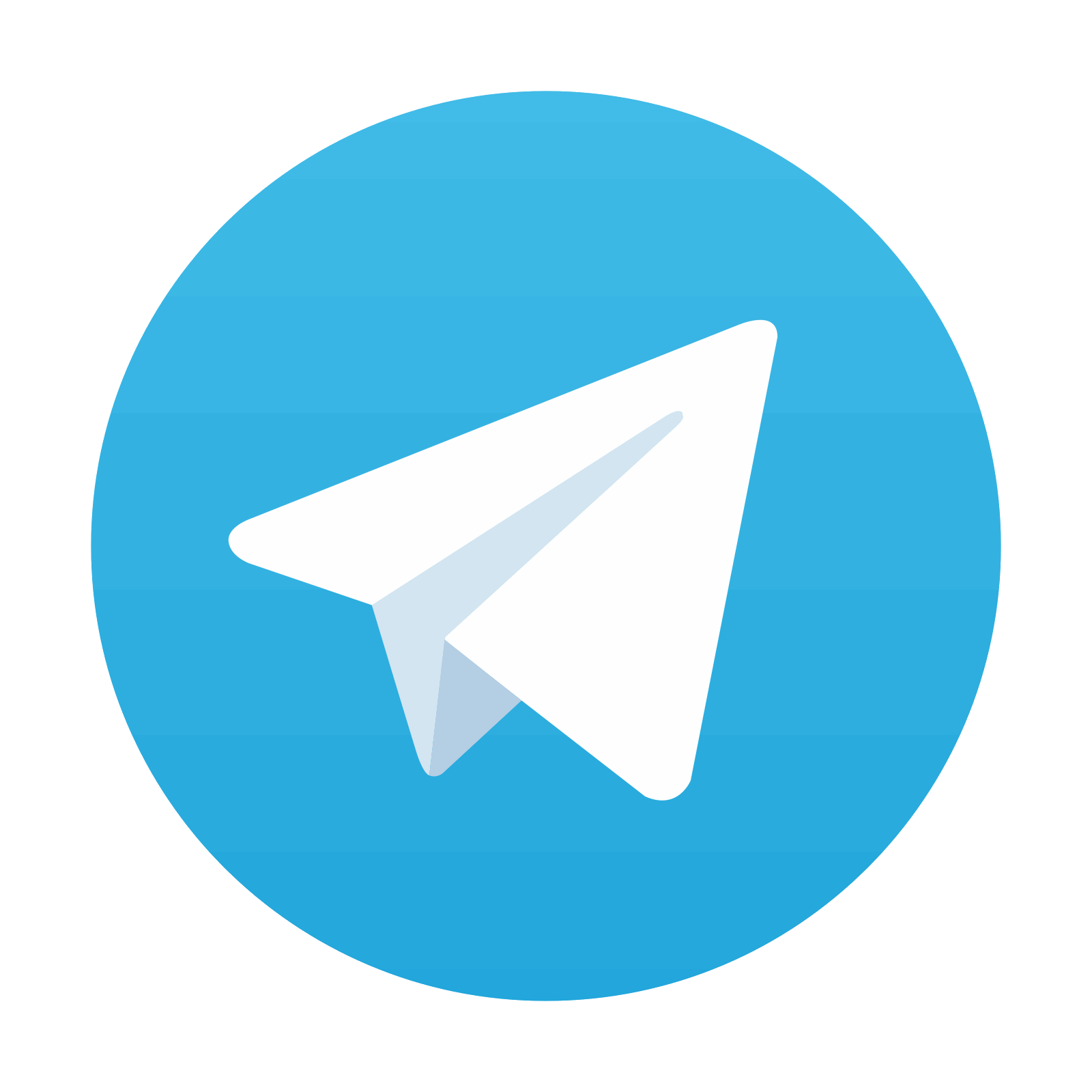
Stay updated, free articles. Join our Telegram channel

Full access? Get Clinical Tree
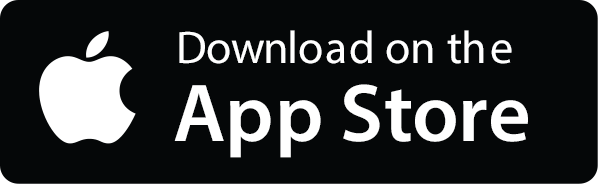
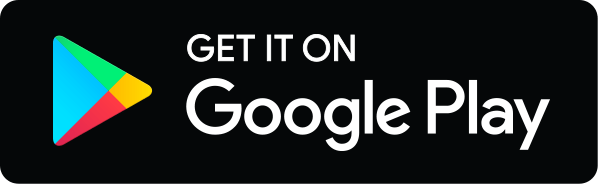