Described in 1913 by Ravenhill as “puna of the nervous type” and reintroduced in the English language literature by Fitch (1964) half a century later, high altitude cerebral edema (HACE) is perhaps the most feared acute altitude illness as it can be associated with substantial morbidity and mortality if not recognized and treated promptly. This chapter is intended to provide a detailed review of this uncommon and poorly understood entity. After reviewing the epidemiology and important clinical aspects of the disease, including the timing and onset, symptoms and signs, and diagnostic approach, the chapter addresses the current understanding of disease pathophysiology and best practices for prevention and treatment. The chapter concludes by considering the expected time frame and extent of recovery and the safety of further ascent once symptoms resolve. Due to differences in case definitions and diagnostic criteria in the various reports in the literature and the challenges of capturing all cases and determining the total number of people traveling to high altitude, the true incidence of HACE remains unclear. Among the better studies on this question, Hackett et al. (1976) reported an incidence of 1% among 278 individuals trekking between 4240 m and 5500 m in Nepal, while. Wu et al. (2006) reported an incidence of 0.26% among over 74,735 construction workers on the Qinghai-Tibetan Railway, which spends over 75% of the 1118 km length at altitudes above 4000 m. Basnyat et al. (2000) have reported a remarkably high incidence of 31% among religious pilgrims trekking to 4300 m in Nepal, but this rate is a clear outlier compared to other reports in the literature and may reflect the particularly fast ascent rate used by those making the pilgrimage. There were also methodological issues with the study, such as the fact that it was based on symptom questionnaires, rather than a detailed physical examination, administered to a random sample of the very large number of pilgrims. The incidence of HACE is increased among those with other forms of acute altitude illness. For example, in the study noted earlier, Hackett et al. (1976) found the incidence increased to 3.4% among those diagnosed with acute mountain sickness (AMS) while other studies have shown that between 13% and 20% of those diagnosed with high altitude pulmonary edema (HAPE) also have HACE (Gabry et al. 2003; Hochstrasser et al. 1986; Hultgren et al. 1996). There are insufficient data to determine whether age, sex, or the presence of underlying medical conditions affect the risk of developing HACE. Richalet et al. (2012) have shown that ascent >400 m/day, history of migraine headaches, impaired ventilatory responses, and desaturation during hypoxic exercise predict the development of severe high altitude illness following ascent; however, HACE is just one of several entities included under that broad category of altitude illness. In contrast to AMS and HAPE, which may be seen with ascent above 2300–2500 m, HACE is typically only seen at altitudes above 3000–4000 m. The average altitude of onset, for example, was 4730 m in a review by Hultgren (1997) and 4695 m (range 3200–5490 m) in another series by Houston and Dickinson (1975). These figures could be slight overestimations, however, as affected individuals may have been manifesting early signs of HACE at lower elevations than noted in the reports yet continued ascending. Onset may also occur at lower elevations in those with concurrent HAPE (Hultgren 1997). The 3000 m threshold noted previously should not be viewed as absolute, however, as cases have been reported as low as 2100–2700 m (Dickinson 1979; Hackett et al. 1998). Symptoms and signs of HACE do not develop suddenly, as they might with an embolic stroke or subarachnoid hemorrhage and instead develop over a period of hours to days. Most cases begin as AMS and subsequently progress to HACE over a period of 24–72 hours, although progression to coma can occur in as little as 12 hours (Hackett and Roach 2004). Onset of symptoms in an otherwise well individual after more than four to five days at the same elevation should prompt consideration of other diagnoses, although individuals who continue to ascend to higher and higher elevations, as in extreme mountaineering, may develop HACE even with seemingly adequate ascent profiles. The frequency of symptoms and signs in two large series is provided in Table 21.1. In addition to severe headache, which is present in most, but not all affected individuals, the most common signs of HACE include ataxia and altered mental status. Evidence of the latter may be subtle at first and include problems such as apathy, irritability, lassitude, and inability to eat or care for oneself before the individual eventually progresses to more obvious signs including confusion, somnolence and, in the most severe cases, coma. The majority of cases are preceded by AMS, but in rare instances, HACE develops in the absence of antecedent AMS symptoms. Table 21.1 Symptoms and signs of high altitude cerebral edema Because HACE causes encephalopathy, focal neurologic deficits are not typically seen, although they have been documented along with other findings including abnormal reflexes, anisocoria, hallucinations, clonus, cranial nerve palsies, and seizures (Dickinson 1983; Houston and Dickinson 1975; Singh et al. 1969; Wu et al. 2006). Retinal hemorrhages have been reported in HACE (Wu et al. 2006) but can also be seen in otherwise healthy individuals (Bhandari et al. 2017) and are not a harbinger of the development of HACE or other forms of acute altitude illness (Barthelmes et al. 2011). Hypoxemia is seen in many patients with HACE and is either due to poor ventilatory acclimatization or occurs as a consequence of concurrent HAPE. In many cases, patients develop HACE in the absence of respiratory issues, whereas a subset of HACE patients present with concurrent HAPE. The latter pattern, for example, has been well described in several series of MRI findings in patients with HACE from Colorado (Hackett et al. 2019; Yarnell et al. 2000), where a high percentage of the patients had severe hypoxemia and radiographic opacities consistent with HAPE. A review of the cases in the literature suggests that the isolated cases of HACE tend to be more common at higher elevations (>4000 m) seen in the Alps and Himalaya while the cases of concurrent disease tend to occur at lower elevations (∼2500–3000 m) as well as at higher elevations. One hypothesis for this pattern, which has not been systematically examined, is that at higher elevations, ambient hypoxia is sufficient to trigger the pathophysiologic processes described below that lead to HACE, whereas at lower elevations, ambient hypoxia is insufficient to cause such changes. Instead, it is the onset of HAPE that lowers arterial oxygen tensions to the point that HACE pathophysiology ensues (Peter Hackett, personal communication). Because a large number of cases are identified and treated with resolution in resource-limited settings, laboratory and imaging studies are not performed in many patients. For those patients who do access care for evaluation and treatment, the following results of various investigations have been described. Basic laboratory studies are typically normal in HACE, aside from the possibility of a mild, nonspecific leukocytosis. Lactic acidosis is not expected due to the fact that seizures are not commonly seen in HACE. Arterial blood gases often reveal hypoxemia with an increased alveolar-arterial oxygen difference, particularly in those with concurrent HAPE. Patients with isolated HACE typically have normal plain chest radiographs, while those with concurrent HAPE manifest alveolar opacities consistent with pulmonary edema. When lumbar puncture is performed to rule out CNS infection, the opening pressure ranges from normal to elevated, while the protein, glucose, and cell counts are typically in the normal range. Houston and Dickinson (1975), for example, reported opening pressures as high as 240 mm H2O, while Singh et al. (1969) found that pressures were 60–210 mm H2O higher during illness than upon recovery. CT scans of the head show nonspecific changes of diffuse edema, including flattening of the gyri, absent sulci, low-density white matter, and decreased ventricular size (Hackett et al. 1998; Wu et al. 2006; Yarnell et al. 2000). MRI scans show two characteristic findings. First, there is increased signal on both T2- and diffusion-weighted imaging that is limited to white matter, rather than gray matter. For reasons that remain unclear, these characteristic signals are typically found in the genu and splenium of the corpus callosum (Hackett et al. 1998). Second, affected individuals have extensive microhemorrhages (Hackett et al. 1998; Kallenberg et al. 2008; Schommer et al. 2013), which are usually limited to the corpus callosum but have also been reported in the cerebral white matter and cerebellar peduncles (Pichler Hefti et al. 2017; Schommer et al. 2013) (Figure 21.1). Importantly, microhemorrhages in the corpus callosum are very specific for HACE, as they are not seen in individuals who remain healthy nor in those who develop AMS or isolated HAPE following ascent (Kallenberg et al. 2008; Schommer et al. 2013). Because hemosiderin deposits are not eliminated via an intact blood-brain barrier, these lesions remain detectable for more than 50 months following resolution of HACE (Figure 21.2), providing an opportunity to confirm the diagnosis even after HACE has resolved following descent (Schommer et al. 2013). Figure 21.1Brain MRI images from a patient with high altitude cerebral edema. (A) T2 flair image showing increased signal in the genu and splenium of the corpus callosum (white arrows) as well as in the internal capsule (black arrows). (B) Diffusion-weighted imaging showing increased signal in the same regions. (C) Apparent diffusion coefficient (ADC) mapping showing increased signal in the genus and splenium of the corpus callosum (white arrows). (Images provided by Peter Hackett, MD.) Figure 21.2Susceptibility weighted images (SWI) from brain MRI imaging of a single patient with HACE obtained one day (A), one week (B), and 20 months (C) after diagnosis. The arrows point to microhemorrhages in the genus and splenium of the corpus callosum. (Images provided by Peter Hackett, MD.) The degree of abnormalities seen on MRI may be related to the severity of clinical illness. Schommer et al. (2013), for example, examined MRI findings in individuals with a history of HACE as well as those with either HAPE, severe AMS without cerebral edema, or a history of travel above 6900 m without altitude illness. Microhemorrhage scores were low in the latter three groups but elevated in individuals with HACE and correlated with the severity of illness as assessed retrospectively using a clinical HACE score (Figure 21.3). Whether the severity of MRI findings correlates with the time to recovery and overall prognosis is not clear (Hackett and Roach 2004). Figure 21.3Microhemorrhage score and its relationship to the clinical high altitude cerebral edema (HACE) score. Each dot represents a single subject. The horizontal line between 0 and 1 on the y-axis denotes the difference between no microhemorrhages and questionable microhemorrhages. Points above the horizontal line between 1 and 2 denote clear microhemorrhages. Points to the right of the line between 0 and 1.0 on the x-axis denote a clinical diagnosis of HACE. (Adapted from Schommer et al. 2013.) As noted above, if not recognized and treated promptly, HACE can be fatal. Pathology reports from older case series indicate that deceased individuals have gross pathologic evidence of cerebral edema, ranging from mild to severe, as well as petechial hemorrhages, subarachnoid and intracerebral hemorrhage, and vascular thrombosis. Because these findings are taken from only a few, highly selected cases published in the literature, it is unclear how representative such changes are of the majority of cases seen in clinical practice. The differential diagnosis for severe AMS and HACE is provided in Table 21.2. In addition to the items in this list, a variety of other neurologic abnormalities have been described among travelers at high altitude who lack of other features of HACE, including isolated cranial nerve palsies (Murdoch 1994), transient global amnesia (Litch and Bishop 1999), and transient cortical blindness (Hackett et al. 1987). Sudden onset after four to five days at a given elevation, high fever, focal neurologic deficits, meningeal signs, and failure to respond to appropriate treatment for HACE should always prompt consideration of alternative etiologies. While more commonly associated with infection, fever is well documented (Hackett et al. 1998) and should not rule out the diagnosis of HACE if the clinical picture is otherwise compatible. Similarly, as noted above, focal neurologic deficits, such as cranial nerve palsies or hemiparesis, do occur in HACE. Failure of such findings to resolve with supplemental oxygen, treatment in a portable hyperbaric chamber, or descent would argue against a diagnosis of HACE and should prompt evaluation for a cerebrovascular accident. Acute psychosis Acute toxic encephalopathy due to substance ingestion Alcohol hangover Carbon monoxide intoxication Cerebrovascular accident Dehydration Encephalitis Hypoglycemia Hyponatremia Hypothermia Meningitis Migraine headache Physical exhaustion Ruptured intracranial aneurysm or arteriovenous malformation Many patients develop HACE in remote settings far away from sophisticated medical care, in which case the diagnosis must be made based solely on clinical findings rather than head imaging or other laboratory investigations. Because the clinical manifestations may be subtle in early stages of the disease, tests like heel-toe walking and the sharpened Romberg test (Bird et al. 2011) can be used to assess for ataxia, which should not be present in those who are well or simply have AMS. When patients are evacuated to medical facilities, further diagnostic testing, including basic laboratory studies, chest radiography, and head imaging, is warranted to rule out alternative etiologies. Not infrequently, people become ill at high altitude, descend to lower elevation and improve without need for further immediate medical attention but subsequently pursue medical consultation upon returning home to determine whether they had HACE. If the history provided during such delayed evaluations is insufficient to confirm the diagnosis, MRI of the brain can be performed to look for microhemorrhages in the corpus callosum, which, as noted previously, are specific for HACE and persist for many years following illness (Kallenberg et al. 2008; Schommer et al. 2013). From a general standpoint, cerebral edema can develop by way of one or two different mechanisms: (1) a vasogenic process, in which fluid moves from the vascular to the interstitial space due to a combination of increased hydrostatic pressure and/or increased vascular permeability, and (2) a cytotoxic process, typically seen in cerebral ischemia, in which water moves into cells due to changes in ion pump function and intracellular osmolality, thereby causing them to swell. While it has been difficult to identify the precise mechanisms accounting for the development of cerebral edema due to the low incidence of the problem and the difficulties developing a suitable experimental model where one can cause low levels of edema in humans, the general consensus is that HACE is largely a vasogenic process with cytotoxic mechanisms only playing a role in the late stages of very severe, often fatal disease, when extensive edema causes tissue hypoxia and cellular injury (Hackett and Roach 2004). The well-documented findings on brain MRI in patients with HACE are the primary evidence for a vasogenic origin for the edema. Gray matter is very sensitive to cellular energy demand-supply imbalances and, therefore, at higher risk of cytotoxic changes, while vasogenic edema spreads more easily through white matter than gray matter due to differences in their structural arrangement (Bradley 1987; Hackett et al. 1998; Klatzo 1987). The fact that MRI consistently shows reversible white-matter edema in the absence of gray-matter changes, therefore, points more toward a vasogenic process. Microhemorrhages and hemosiderin deposition, which have also been widely reported on MRI following HACE, are indicative of blood-brain barrier disruption and, as a result, also consistent with leakage of fluid and blood from the vascular to the interstitial space (Hackett et al. 1998; Kallenberg et al. 2008; Schommer et al. 2013). The fact that patients usually recover over a relatively short period and in the majority of cases have no sequelae would also fit more closely with a vasogenic process, as the injuries that lead to cytotoxic edema tend to either be permanent or take much longer to resolve (Hackett and Roach 2004). Vasogenic edema develops due to changes in the Starling forces and, in particular, is related to increases in hydrostatic pressure and/or changes in vascular permeability. Analysis of these variables in HACE has been limited by a lack of adequate animal models and the difficulties associated with measuring vascular pressures and permeability and other aspects of brain metabolism in humans. The inciting event for the increase in hydrostatic pressure is an increase in cerebral blood flow with hypoxia due to vasodilation in distal cerebral arterioles (Severinghaus et al. 1966; Wadsworth 1994). The magnitude of change will vary between individuals as a function of their ventilatory responses to hypoxemia, acid-base balance, and cerebrovascular sensitivity to the changes in PaO2 and PaCO2. Although increases in cerebral blood flow may cause brain swelling following ascent due to an increase in vascular volume (Morocz et al. 2001), this depends on the effectiveness of venous outflow (discussed below). As such, changes in cerebral blood flow do not raise capillary hydrostatic pressure high enough in most individuals to cause edema. The other factor that may help raise hydrostatic pressure above the required threshold is some impairment in cerebral venous outflow. This idea was raised by a case report in which an individual treated for HAPE with 10 cm H2O of positive end-expiratory pressure (PEEP) through a tight-fitting mask subsequently developed HACE (Oelz 1983). By raising intrathoracic pressure, PEEP decreased the pressure gradient for venous return from the intracranial space, thereby increasing capillary hydrostatic pressure. Drawing on evidence that abnormalities in cerebral venous drainage may contribute to development of idiopathic intracranial hypertension (Higgins et al. 2005) and other clinical syndromes, Wilson et al. (2011) have more recently argued that impaired outflow due to anatomic variants in larger cerebral veins may be the critical factor in increasing venous and capillary hydrostatic pressure enough to cause edema. Another consideration would be that as edema develops in the early stages of HACE, compression of small, deep cerebral veins further impedes venous outflow, worsening venous hypertension and setting in motion a problematic feedback loop (Sagoo et al. 2017). While studies have shown evidence of cerebral venous hypertension at high altitude, including retinal vein dilation (Bosch et al. 2009) or impaired regional brain oxygenation due to increases in the venous compartment of intracerebral blood volume (Heine et al. 2009), the link between venous hypertension and AMS or HACE has not yet been confirmed. Finally, recent work has conceptualized the idea of a (g)-lymphatic, or glymphatic system, in the brain, consisting of a series of periarterial and perivenous channels associated with glial cell aquaporin channels that play a role in removal of metabolic waste products from the central nervous system (Benveniste et al. 2017; Simka et al. 2018). From a theoretical standpoint, alterations in this system at high altitude could affect clearance of interstitial fluid and therefore predispose to edema formation, but this has yet to be investigated. Increased permeability, mediated by chemical factors such as arachidonic acid, bradykinin, catecholamines (Raichle et al. 1978), free radicals, nitric oxide (Clark et al. 1999), reactive oxygen species, and vascular endothelial growth factor (VEGF), may also contribute to edema formation (Hackett and Roach 2004). A role for VEGF, a potent inducer of angiogenesis upregulated in hypoxia by HIF-mediated pathways, was first suggested by Severinghaus (1995), who proposed that macrophage expression of VEGF and other cytokines dissolved the capillary basement membrane and degraded extracellular matrix, thereby increasing capillary permeability. This link has been examined in several animal studies. Xu and Severinghaus (1998), for example, demonstrated increased VEGF mRNA expression in rat brains after exposure to hypoxia (FIO2 of 6–12%), while Schoch et al. (2002) demonstrated increased VEGF expression and capillary permeability in mice, which correlated with the degree of hypoxia and was prevented by blocking VEGF activity. Providing further support for this concept, other studies have demonstrated that administration of antiangiogenesis agents (Tarshis et al. 2016) or prolyl hydroxylase inhibitors (Singh et al. 2016) to rats exposed to hypoxia can decrease cerebrovascular leakage, VEGF expression, and edema formation. Whether VEGF actually plays this hypothesized role in humans remains unclear. The rarity of HACE makes it difficult to study VEGF expression in such cases while those studies that have explored the relationship between VEGF and AMS symptoms have reported conflicting results (Schommer et al. 2011; Tissot van Patot et al. 2005). Given the predominance of neurologic symptoms in acute mountain sickness and high altitude cerebral edema and the fact that most HACE cases are preceded by AMS, there has long been a suspicion that AMS and HACE share a similar pathophysiology and that AMS simply represents a mild form of cerebral edema. This continuum has been brought into question recently (Bailey et al.
Introduction
Epidemiology
Altitude and Timing of Onset
Clinical Features
Symptoms and signs
Concurrent HACE and HAPE
Investigations
Basic Laboratory Studies and Chest Imaging
Lumbar Puncture
Head Imaging
Postmortem Findings
Diagnostic Approach
Differential diagnosis
Diagnostic strategies
Pathophysiology
Mechanism for vasogenic edema formation
Increased Hydrostatic Pressure
Increased Capillary Permeability
The link between AMS and HACE
Stay updated, free articles. Join our Telegram channel

Full access? Get Clinical Tree
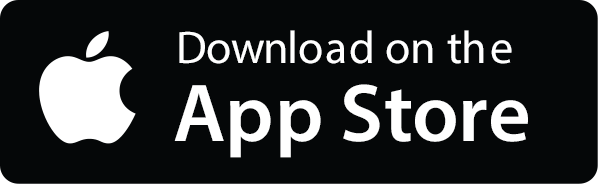
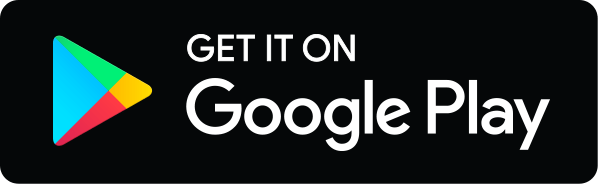