Brief history of research on long-term residents of high altitude Duration of exposure in high altitude residents Differences in phenotype between highland populations Other health issues in high altitude residents Increasing numbers of people are living permanently at high altitude. Whereas, as of 2004, about 89.3 million people were living at altitudes above 2500 m, including 25.9 million and 5.4 million living more than 3500 m and 4500 m above sea level, respectively (Beall 2014), it is now estimated that over 200 million people live above 2500 m (Moore et al. 1998; WHO 2019). High-altitude residents of the South American Andes, the Tibetan plateau, and Ethiopia highlands who have existed at altitude for hundreds of generations have developed distinct adaptive and maladaptive strategies while living in their respective hypoxic environments. This chapter does not deal directly with habitation at altitude (Chapter 3) nor genetic differences among highland populations (Chapter 6) but a related fascinating topic. This is the fact that successful groups of humans who have adapted to high altitude, Himalayan highlanders (Tibetans and Sherpa), Andeans (Aymara and Quechua), and Ethiopians (Amhara and Oromo), exhibit very different phenotypes (Table 4.1), although substantial variation is noted for some traits within each continental population. After describing the concept of adaptation in greater detail, a brief historical perspective of research in these high altitude populations is provided. Differences and similarities in phenotypes between these groups are reviewed, including pregnancy and childhood development. The chapter concludes with a review of another important issue for residents of high altitude regions, the burden of non-communicable and communicable diseases and how this burden is changing over time. Table 4.1 Summary of the key phenotypical differences between Tibetan, Andean, and Ethiopian high altitude populations when compared to acclimatized lowlanders When referring to phenotypic traits that have been acted upon by natural selection, the term “adaptation” has a specific meaning; namely, any features of structure, function, or behavior that increase the ability to survive and/or reproduce in a given environment (Moore 2017). Adaptation is distinct from “acclimatization” or the time-dependent rise in ventilation, hemoglobin concentration, heart rate, and redistribution of blood flow that serve to restore arterial O2 content and preserve O2 delivery to vital organs. Acclimatization processes are summarized in Chapter 7 and will be referred to within this chapter for purposes of making comparisons between acclimatized newcomers and long-term resident groups. Just as many people regard Paul Bert as the father of modern high altitude physiology, Carlos Monge Medrano (1884–1970) merits the title of father of the study of permanent high altitude residents. He started the influential Peruvian school in Lima, which was subsequently continued by Alberto Hurtado Abadilla (1901–83) and Monge’s son, Carlos Monge Cassinelli (1921–2006). Mention was made earlier of Barcroft’s unguarded statement that “All dwellers at high altitude are persons of impaired physical and mental powers” (Barcroft 1925). Monge took great exception to this and in his influential book Acclimatization in the Andes (Monge 1948) he referred to “the incredible statement of Professor Barcroft, the Cambridge physiologist, who after staying three months at Cerro de Pasco.” Monge made the point that because of the “climatic aggression” of high altitudes as he referred to it, Andean man should not be assessed using the same criteria as people who live near sea level. In fact, at one stage, Monge attributed Barcroft’s statement to the fact that the latter had mountain sickness at the time! Monge made extensive studies of the ability of permanent residents of the Andes to withstand the hypoxia and cold of the environment. Nevertheless, he is best known for his work on chronic mountain sickness, also known as Monge’s disease (discussed in Chapter 24), which he set out in his book La Enfermedad de los Andes (Monge 1928). In this book, he describes the condition associated with severe polycythemia, cyanosis, and a variety of neuropsychological complaints including headache, dizziness, somnolence and fatigue. Initially, the condition was thought to be polycythemia vera, but was later shown to be a distinct malady. Alberto Hurtado (1901–83) was a physiologist who trained under Monge and who made extensive studies of the high-altitude residents of Morococha at an altitude of 4550 m. Typically, the arterial PaO2 was only 45 mmHg with a corresponding arterial oxygen saturation of 81%. However, interestingly, because of the polycythemia, which raised the hemoglobin concentration to nearly 20 g dL−1, the arterial oxygen concentration was actually above the normal sea level value. The son of Carlos Monge Medrano, Carlos Monge Cassinelli (1921–2006) was a biologist with broad interests in high altitude including comparative physiology. However, he was very interested in the relationships between high altitude, polycythemia, and chronic mountain sickness and many of his studies were reported in a classical book (Winslow and Monge 1987; reviewed more recently by Heggie 2019). The Peruvian school remains very active today with high altitude scientists such as Fabiola León-Velarde, Francisco Villafuerte, and others. Environmental physiology was a major line of human biology research as part of the Human Adaptability Project of the International Biological Programme, a worldwide study of human adaptability (Weiner 1964). A high altitude program was suggested, with plans to conduct coordinated, parallel, multidisciplinary studies on the indigenous populations of the Andes, Himalayas, and Ethiopian highlands. A team of researchers from Pennsylvania State University, the Instituto de Biolgía Andina de Peru, and dozens of other institutions conducted comprehensive studies on the Quechua population of Nuñoa, Peru (∼4000 m) in the 1960s detailed in Man in the Andes: Multidisciplinary Study of High Altitude Quechua (Baker and Little 1977). Some of the general physiology-related questions of the expedition included: To which unique environmental stresses has the population adapted; how has the population adapted culturally and biologically to high altitude stresses; and how did these adaptations become established? The landmark textbook published in 1978 (Baker 1978) compiles and integrates the work on this topic. Although to a lesser extent than work in the Andeans, early studies in the Sherpa were performed as far back as 1925 (Pugh 1962; Pugh et al. 1964; Somervell 1925). The Kunde Hospital, funded by Sir Edmund Hillary, was founded in 1966 and has been a site of research on Sherpa physiology. Since 1990, the Ev-K2-CNR Pyramid Research Laboratory, located outside the village of Lobuje and near Everest base camp, has served as a hub for human physiology research, including many studies on the resident Sherpa population. Notably, the UBC-Nepal Expedition and Xtreme Everest teams have recently conducted comprehensive integrative physiology and metabolic investigations on Sherpa and lowlanders both here and in other areas of Sagamartha National Park. Fewer studies have examined the residents of the Ethiopian highlands when compared to those of the Andes and Himalayas. In the 1960s, a group from England and Ethiopia conducted a research expedition to the Ethiopian highlands and collected demographic and clinical information on residents (primarily Amhara) of the Simien Mountains (Harrison et al. 1969). Later work was conducted in the 1990s by Cynthia Beall with the Ethiopian physician Amha Gebremedhin, and subsequently a group led by Roger Hainsworth. As with the earlier studies, these investigations were also conducted in the Simien Mountains. More recently, high altitude populations in the Simien Mountains (largely Amhara) in Northern Ethiopia have been compared to high altitude populations of the Bale Mountains (largely Oromo) in Southern Ethiopia (Alkorta-Aranburu et al. 2012). As the Amhara have resided at high altitude for much longer than the Oromo (>5000 years versus ∼500 years), this has given scientists a natural model for studying high altitude adaptation. Other groups from Argentina, Bolivia, Chile, China, and Tibet continue to do extensive work on high altitude residents. The gold standard for investigating human adaptation to high altitude remains the multiple population–single stressor model; in other words, comparing how separate populations have adapted to life at high altitude. Despite the growing body of work in this area, few groups have accomplished what was proposed by the Human Adaptability Project of the International Biological Programme. As mentioned earlier, this program included some of the first large-scale insights on the physiology, hematology, growth and development, reproduction, and nutrition of peoples living above 2500 m in the Andean, Ethiopian highlands, and Himalayan regions (Baker 1978; Weiner 1964). Studies by Robert Winslow and others have provided detailed support for phenotypic differences between Quechua and Sherpa high altitude groups (Bigham et al. 2013; Claydon et al. 2008; Ghosh et al. 2019; Stembridge et al. 2019). Other groups have compared two populations, but aside from a study by Beall (2006), simultaneous comparisons among three populations are generally lacking and more comprehensive investigations are required to expand our understanding of human adaptation to generational high altitude stress. The timing of this research is essential, as many of the high altitude populations are undergoing rapid acculturation, urbanization, and migration, which is changing diet, physical activity habits, and other traditional aspects of the lives of the indigenous communities (Alae-Carew et al. 2019; McCloskey et al. 2017; Peng et al. 2019; Smith 1999), with subsequent effects on the burden of noncommunicable disease (discussed later) and other issues. Although there is considerable debate regarding specific durations at altitude, the general consensus is that the Old World plateaux (Ethiopian and Tibetan) have been settled for longer than the Altiplano in the New World (Andes) (Aldenderfer 2019; Alkorta-Aranburu et al. 2012; Beall 2006 2007; Ossendorf et al. 2019; Zhang et al. 2018b) as outlined in Figure 4.1. These three populations have likely lived under the stress of high altitude for a sufficient duration for natural selection to have changed the frequency of adaptive alleles. The genetic differences between these populations are presented in Chapter 6. As noted earlier, highland populations in the Himalayas and South American Andes have been the most thoroughly studied, while less research has been focused on Ethiopian populations. Nevertheless, the fact that these groups of highlanders have such different physiological characteristics, including hematological, respiratory, circulatory, and metabolic traits and developmental aspects, is truly remarkable. It is these physiological characteristics that are the subject of the next section of this chapter. More detail about the physiological responses to hypoxia seen in unacclimatized lowlanders traveling to high altitude is provided in subsequent chapters. Figure 4.1Summary of the proposed duration of Ethiopian, Tibetan, and Andean highlander settlements. Historical records indicate that the Oromo tribe of Ethiopia has only been settled in the highlands for ∼500 years (Lewis 1966); however, the Amhara tribe has been living at altitude for potentially up to 70,000 years (Aldenderfer 2003; Lewis 1966; Pleurdeau 2005). Humans are thought to have occupied the Tibetan plateau ∼30,000–40,000 years ago (Zhang et al. 2018b) and eventually crossed a land bridge that once connected present day Russia and Alaska, which facilitated North and South American settlements such as the Andean highlanders ∼7000–11,000 years ago (Haas et al. 2017). Hemoglobin concentration is a defining feature in the differential manifestations of adaptation between high altitude Andeans and Tibetans (Beall 2007) (Table 4.1). Tibetans demonstrate a hemoglobin concentration that is lower than the Andeans and that would be comparable to sea level residents (Beall 2007); the lower concentration is associated with greater reproductive success (Jeong et al. 2018) and exercise capacity (Simonson et al. 2015). Although some Ethiopian populations, especially the Amharas, have also been reported to have low hemoglobin concentration (Beall 2006; Beall et al. 2002; Cheong et al. 2017), the relationships to greater reproductive success or exercise capacity has not been established. Figure 4.2 shows hemoglobin concentrations plotted as a function of altitude and nicely demonstrates the higher concentrations seen in the Andeans and relatively low concentrations in the Tibetans and Ethiopians. Figure 4.2Hemoglobin concentration versus altitude for Tibetans (filled circles), Andeans (open circles), and Europeans (filled triangles). (Source: Beall 2000.) It has been widely assumed that lower hemoglobin concentration in Tibetans is achieved via the absence of a significant erythropoietic response to hypoxemia. However, this oversimplification disregards the equally important contribution of plasma volume in the regulation of hematocrit (Donnelly 2003). As discussed in Chapter 13, a larger plasma volume would decrease hemoglobin concentration and could decrease heart rate by mediating a larger stroke volume, but the importance of these volumetric measures has only recently been explored. It has been found, for example, that hemoglobin mass is highest in Andeans, but also elevated in Sherpa compared to lowlanders (Stembridge et al. 2019; Figure 4.3). Sherpa, however, demonstrated a larger plasma volume than Andeans, resulting in a comparable total blood volume at a lower hemoglobin concentration (Figure 4.3). The implications of this phenomenon provide a mechanism that enables Tibetans to maximize total oxygen carrying capacity of the blood without the detrimental effect of a high viscosity on microcirculatory blood flow. Further discussion on this topic can be found in Chapter 13. Figure 4.3The relationship between hemoglobin mass and exercise capacity in lowlanders, Sherpa, and Andeans at sea level and high altitude. Note: there were no relationships between hemoglobin concentration and exercise capacity. (Modified from Stembridge et al. 2019.) Increased hemoglobin-oxygen binding affinity has typically been regarded as beneficial at altitude by enhancing the rate of equilibration across the alveolar-capillary barrier. Reports of hemoglobin-oxygen binding affinity differ widely across studies, although unrelated to exercise capacity, recent data indicate greater blood-oxygen binding affinity (i.e., a decreased P50) in both Tibetans and Han Chinese volunteers at 4200 m when compared to lowlanders at sea level (Simonson et al. 2014). Andean residents also exhibit decreased P50 at high altitude when compared to lowlanders at sea level (Balaban et al. 2013). Any benefits of reductions in P50 at high altitude are unclear and likely require some adaptation at the tissue level to account for the higher affinity. Beall (2000) reported a lower resting ventilation in Aymara compared with Tibetans as shown in Figure 4.4. Here, the resting ventilation in L min−1 is plotted against the age of the subjects over a large range from less than 20 to 90 years of age. Males are shown by the filled circles and females by the open circles. Note that the resting ventilations of the Aymara are uniformly low with females’ ventilation typically less than that of males. This is somewhat surprising because many measurements of resting ventilation at high altitude indicate that females have higher values. For example, in her early studies of alveolar PCO2 in the Colorado mountains, FitzGerald (1914) found that women had a lower alveolar PCO2 than men at the same altitude, indicating that they had higher ventilation. This finding is consistent with observations that Andean females at 4300 m have a higher ventilation during their luteal phase that is associated with higher concentrations of progesterone, a known respiratory stimulate (León-Velarde et al. 2001). Figure 4.4Comparison of resting ventilation in Tibetans and Aymara plotted against age. Males, filled circles; females, open circles. (Source: Beall 2000.) A striking feature of Figure 4.4 is the large range of resting ventilations in Tibetans, and the corresponding fact that many Tibetans have higher resting ventilations than Aymara. Additional analysis of these data, where Tibetans and Andeans are compared at the same altitude, indicate that there is a difference between the two populations for resting ventilation (Beall 2000). The reason for these differences in ventilation is clarified when we look at the ventilatory responses to hypoxia (HVR) for the two populations, as shown in Figure 4.5. This looks similar to Figure 4.4 in that the Aymara have a fairly uniformly low HVR over the large age range, whereas there is much more variability for Tibetans. Furthermore, the relatively high response of the Tibetans may well be due to the genetic factors described earlier. Tibetans’ genomes exhibit an adaptive signal at EPAS1, a gene that codes for HIF-2α, and there is evidence that this transcription factor plays an important role in many potential mechanisms for adaptation, including the induction of tyrosine hydroxylase gene that increases the chemosensitivity of the carotid body. Early work by Severinghaus et al. (1966) in both healthy and polycythemic Andean highlanders found the HVR to be severely blunted in a limited number of highlanders, with a large range of hematocrit associated with a very small change in hypoxic chemosensitivity. The implication of alterations in these ventilatory patterns in these different populations on arterial blood gases and acid-base balance has yet to be reported. Figure 4.5Hypoxic ventilatory response (HVR) in Tibetans and Aymara plotted against age. Males, filled circles; females, open circles. (Source: Beall 2000.) Figure 4.6 shows arterial oxygen saturation estimated by pulse oximetry plotted against altitude for Tibetans and Andeans in comparison to Europeans. Based on the higher ventilations and hypoxic ventilatory response of the Tibetans, we would expect a higher arterial oxygen saturation, and this seems to be true above 4000 m—but two open circles between 3000 m and 4000 m are not in agreement. The data show samples of 10 or more highlanders with a mean age from 10 to 50 years. Most studies indicate arterial oxygen saturation is lowest among Tibetans, is relatively higher in Andeans, and is highest in Amhara Ethiopians (reviewed in Simonson [2015]). Figure 4.6Arterial oxygen saturation (SaO2) plotted against altitude for Tibetans (filled circles), Andeans (open circles), and Europeans (filled triangles). (Source: Beall 2000.) As outlined later in this chapter, it has been proposed that a genetic factor underlies oxygen saturation differences observed among Tibetan mothers and is further associated with decreased infant mortality in this population (Beall et al. 2004). Additionally, oxygen saturation is higher in Tibetan relative to Han Chinese or Andean infants at comparable altitudes, and such differences are hypothesized to underlie susceptibility to high altitude pulmonary hypertension and chronic mountain sickness later in life in the latter populations (Niermeyer et al. 2015). The long-term effects of hypoxia and hypertension are further noted in a recent study that showed individuals with excessive erythrocytosis, when compared to controls, were more likely to have been born to mothers who exhibited hypertension and perinatal hypoxia (Julian et al. 2015). The data on the four traits shown in Figures 4.2–4.6, resting ventilation, hypoxic ventilatory response, oxygen saturation, and hemoglobin concentration, show considerable scatter. Beall (2000) carried out additional statistical analysis of the data and found that the first two traits were more than 0.5 standard deviation higher in Tibetans than the Aymara. In addition, the Tibetan means were more than one standard deviation lower than the Aymara means for the last two traits. Also, Beall calculated the “effect size” for the four traits, that is the difference in the mean values of 20- to 29-year-old males divided by their pooled standard deviation. The result showed that the effect sizes of resting ventilation and hypoxic ventilatory response are large and positive, that is, the Tibetan means are larger than Aymara means, and the effect sizes of arterial oxygen saturation and hemoglobin concentrations are large and negative; that is, Tibetan means are smaller than Aymara means. As detailed in Chapter 17, periodic breathing during sleep is almost universal in newcomers to high altitude and tends to intensify over time. For example, in lowlanders, periodic breathing was not altered or improved over 13 months at the Antarctic base Concordia, located at an equivalent altitude of 3800 m (Tellez et al. 2014). Likewise, Andean natives, especially those with chronic mountain sickness, present with marked periodic breathing (Bernardi et al. 2003; Guan et al. 2015; Julian et al. 2013; Moraga et al. 2014; Rexhaj et al. 2016; Sun et al. 1996). In contrast to both lowlanders and the Andeans, the Himalayan Sherpa tend to have little to no periodic breathing during sleep (Hackett et al. 1980; Lahiri et al. 1983). Lahiri et al. (1983) have argued that this represents an important feature of the true adaptation of the Sherpas to high altitude. It is unknown if sleep disordered breathing occurs in Ethiopian high altitude populations. A limited number of studies provide insight into the basis of cardiac adaptations in highland populations. Compared to lowlanders, Tibetans and Sherpas have a higher maximal heart rate at altitude (Pugh 1962; Pugh et al. 1964; Sun et al. 1990; Wu 1990), have no difference in resting cardiac output, but exhibit mechanical reserve similar to lowlanders at sea level (i.e., smaller relative left ventricle size and reduced systolic deformation and slower diastolic untwisting). Interestingly, it has recently been reported that directly measured sympathetic nervous activity is significantly lower in Sherpa when compared to lowlanders at the same high altitude, which may be advantageous for blood pressure homeostasis (Simpson et al. 2019). Although not a universal finding (Tremblay et al. 2018), lower sympathetic nervous activity in the Sherpa may explain the “high-flow” phenotype that has been reported in their peripheral circulation (Erzurum et al. 2007). However, it should be noted that this is not a universal finding, as more recent studies have reported comparable blood flow patterns in the peripheral circulation in Sherpa, Andeans, and lowlanders at ∼4300 m (Tremblay et al. 2018; Tremblay et al. 2019). As outlined in Chapter 12, given the drop in arterial oxygen content at high altitude, increases in cerebral blood flow are necessary to maintain oxygen delivery to the brain. When lowland natives travel to high altitude, cerebral blood flow increases in proportion to the reduction in arterial oxygen content, leading to the maintenance of cerebral oxygen delivery (Ainslie and Subudhi 2014). This physiological response is intact at low, moderate, and severe high altitude (Hoiland et al. 2018). This pattern of cerebral blood flow regulation in relation to changes in arterial oxygen content is driven primarily by changes in hemoglobin concentration during acclimatization to high altitude with the remaining influences including ventilatory acclimatization and, to a lesser extent, alterations in cerebral vascular reactivity to alterations in arterial blood gases (Hoiland et al. 2018). In recent years it has become apparent that there are unique physiological adaptations in cerebral blood flow regulation among the three high altitude native populations (Table 4.1). Although not consistently observed in the high-altitude native Tibetans and Sherpa (Huang et al. 1992), cerebral blood flow and oxygen delivery are lower than that of lowland natives in both adults (Hoiland et al. 2019) and children (Flück et al. 2017). Such adaptations may underscore the unique resistance to neurological damage exhibited by Tibetans and Sherpa following extreme high altitude climbing (>8000 m) (Garrido et al. 1993; 1996). Studies utilizing transcranial Doppler, which measures cerebral blood velocity as a surrogate of blood flow, have indicated that cerebral blood flow in Andeans is ∼20% lower (Appenzeller et al. 2006) than that which is commonly observed in lowland natives at a similar altitude (Lucas et al. 2011). This is in agreement with the first study suggesting cerebral blood flow was lower in eight Peruvian natives living at 4300 m altitude in Cerro de Pasco (Milledge and Sorensen 1972). The authors found the mean arteriovenous oxygen content difference across the brain was 7.9 ± 1 vol%, which is about 20% higher than the published sea level mean of 6.5 vol%. Assuming that brain metabolic rate was normal, they suggested cerebral blood flow was proportionately ∼20% below sea-level normal values and postulated that the mechanism might be high blood viscosity given the high hematocrit (58 ± 6%) in these subjects. However, it is important to consider the impact of methodology on these outcomes, as this synthesis of data are from multiple reports. A direct comparison of lowlander to Andean cerebral blood flow and oxygen delivery has never been made, with the current comparisons impacted by unmatched arterial blood gas stimuli, among other methodological issues. Whether or not the Ethiopians express a unique phenotype for cerebral blood flow regulation at high altitude remains unknown. The currently limited data that are available indicate that the cerebral circulation of Ethiopians is less sensitive to hypoxia but more sensitive to alterations in carbon dioxide than other high altitude native populations and lowlanders (Claydon et al. 2008). How these functional differences coalesce to determine overall cerebral blood flow and oxygen delivery remains an unresolved area of research. Groves et al. (1993) showed the relationship between mean pulmonary artery pressure and arterial PaO2 in Tibetans, Andeans, and North Americans. In general, the fall in alveolar PaO2 triggers hypoxic pulmonary vasoconstriction (HPV), which contributes to a rise in pulmonary artery pressure. There is a striking difference between Andeans and Tibetans, with the Andean response not differing much from that in North Americans, while the Tibetans have a strikingly reduced or “blunted” HPV (Groves et al. 1993). Although Tibetan residents at sea level further exhibit a minimal pulmonary vasoconstrictor response to hypoxia, which was attributed to hyporesponsiveness of the hypoxia- inducible factor pathway (Petousi et al. 2014), many studies report that pulmonary artery systolic pressure values in Sherpa are similar to lowlanders at high altitude (Foster et al. 2014; Hoiland et al. 2015; Stembridge et al. 2015). The Amhara residents of Ethiopia at 3700 m have also been reported to have elevated pulmonary artery pressure, but without the elevated pulmonary vascular resistance characteristic of the classic model of the response to long-term hypoxia by the pulmonary vasculature (Hoit et al. 2011). Compared to lowlanders, Sherpas have a higher capillary-density-to-muscle-fiber ratio and decreased mitochondrial density (Kayser 1991) and exhibit a metabolic shift toward glucose metabolism under conditions of acute hypoxia (Hochachka et al. 1996). Recent analysis of skeletal muscle biopsies provides evidence for improved oxygen utilization efficiency, decreased fatty acid oxidation, and increased antioxidant levels in Sherpa (Horscroft et al. 2017), although such data are lacking in other highland populations. Andeans and Tibetans do not exhibit the same significant decline in exercise capacity typically observed in acclimatized individuals at high altitude (Chapter 18; Fulco et al. 1998). A within-population examination of exercise capacity and oxygen transport showed that Tibetans with lower hemoglobin concentration exhibit higher peak V. In-depth reviews regarding distinct traits exhibited by high altitude residents are available (Beall 2006; Gilbert-Kawai et al. 2014; Moore 2017; Simonson 2015). Efforts to identify relationships among key phenotypes, fully examine developmental changes in a longitudinal context, and directly compare within and across Tibetan, Andean, and Ethiopians populations will be necessary to unravel the complexities of highlander adaptations and maladaptations to high altitude. Beyond the phenotypic differences discussed in detail above, another area of attention in the literature has been the question of whether differences exist between high and low altitude populations with regard to other health issues, such as pregnancy and development and the burden of noncommunicable and communicable disease. These topics are discussed in this section. When considering such issues, one important challenge is identifying the underlying cause of any observed differences. While such differences may, in some cases, relate to the effect of long-term exposure to hypoxia, in other situations socioeconomic factors, such as access to medical care, differences in diet and activity levels, and other issues associated with residence in remote mountain communities may be the primary explanatory variable. As will become apparent in the discussion that follows, this can be difficult to sort out from the available literature. In humans, the effect of altitude on pregnancy has been extensively studied in high altitude residents (>2500 m), with fewer studies of unacclimatized lowlanders traveling to altitude during pregnancy (Keyes et al. 2016) or lowlanders residing at high altitude for longer periods of time. A major focus of many of these studies is the effect of chronic hypoxemia on birth weight. In general, birth weights are lower at altitudes >2500 m (Giussani and Davidge 2013; Giussani et al. 2001; Jensen and Moore 1997; Krampl et al. 2000; Levine et al. 2015), although direct comparisons between Tibetans and Andeans have not been conducted. It has been determined that there is a decrease in birth weight of approximately 102 g/1000 m elevation, which correlates with the decrease in atmospheric PaO2 of approximately 11% per 1000 m (Moore et al. 2011). Thus, the effect of hypoxia on the developing fetus is graded and proportional to the severity of the hypoxia (Jang et al. 2015). The cause of birth weight reduction is multifactorial. Vessels within an at-term placenta obtained at high altitude are more dilated and less frequently associated with smooth muscle, compared with sea level (Espinoza et al. 2001; Zhang 2002). This together with higher uterine artery blood flow in normotensive women at high altitude buffers fetal demands (Jensen and Moore 1997; Keyes et al. 2003) such that the high altitude fetus of a normotensive mother is only modestly hypoxic with lower heart rate and birth weight (Browne et al. 2015). However, the combination of lower uterine artery blood flow and early-onset preeclampsia may exaggerate fetal hypoxia leading to further fetal hypoxia, slower heart rate, and intrauterine growth restriction (Tissot van Patot et al. 2003; Tissot van Patot et al. 2010; Yung et al. 2012). As noted previously, unlike many other phenotypes (Table 4.1), there do not seem to be any major differences in adaptation strategies during pregnancy between the high altitude populations of the Andes and Himalayas. The adverse effect of altitude on birth weight is independent of maternal age, parity, and prenatal care, but is heavily influenced by population ancestry. In particular, the magnitude of effect is lessened among multigenerational high altitude communities, suggesting a genetic component in the adaptation (reviewed in Moore et al. 2011). Genetic factors that vary between Andeans and Europeans include genes related to the HIF-1 pathway in placentae (Gundling et al. 2013), soluble Epo receptor (Wolfson et al. 2017), and increased antioxidant status (erythrocyte catalase and superoxide dismutase) activity (Julian et al. 2012). An increased risk for certain forms of birth defects has been noted at high altitude. In a collaborative study, based on information from 1,668,722 consecutive births from three hospitals situated between 2600 m and 3600 m in Bogota (Colombia), La Paz (Bolivia), and Quito (Ecuador), Castilla et al. (1999) found increased risk of craniofacial defects, cleft lip, microtia, preauricular tag, brachial arch complex, constriction band complex, and anal atresia but a decreased risk of neural tube defects, anencephaly, and spina bifida. Later work by the same group provided further evidence that high altitude residence is a risk factor for cleft lip +/− cleft palate (Poletta et al. 2007). The high altitude infant starts life smaller than the average low altitude baby does, and its early growth is slower. Milestones such as sitting and walking seem to be delayed, but the differences between high and low altitude residents of the same ethnic group are less than those between different ethnic groups or between urban and rural populations (Clegg 1978). In Quechua natives in Peru, throughout childhood the high altitude child lags behind his low altitude counterpart in height by about two years. The adolescent growth spurt is less pronounced in high altitude youths but their growth continues for about two years longer and their adult stature is not reached until 22 years of age (Frisancho 1978). A large cross-sectional survey (N = 1458) that examined the effect of altitude on early childhood growth in Tibet reported that children born and living above 3500 m had two to six times the risk of stunted growth compared with those at 3000 m when socioeconomic and other factors were controlled (Dang et al. 2008). This finding, however, contrasts with another report that showed no association between stunting and altitude (Harris et al. 2001). It was, therefore, concluded that stunting was more related to malnutrition and illness between 12 and 24 months of age than altitude per se. Broadly consistent with this observation, a study from Ecuador found very little difference in rates of body weight increase in children at high altitude (>3000 m) compared with children from low altitude (Leonard et al. 1995). There were some minor differences in rates of height increase but the authors concluded that hypoxia plays a relatively small role in shaping growth in the first five years after birth (Leonard et al. 1995). Differences in population’s studies, experiential designs and challenges untangling socioeconomic and genetic factors as well as access to health care resources, are likely important explanations for the discrepancies between studies. For example, additional gene-by-environment interactions have been noted in terms of stature, as Native American ancestry is associated with short stature and limbs at high but not low altitude in Andeans (Pomeroy et al. 2015). Interestingly, however, early studies in Ethiopia reported that high altitude children were taller and heavier for their age than lowlanders (Frisancho 1978). Menarche occurs one to two years later in girls living in the Andes, Himalayas and Tien Shan than in low altitude residents (Frisancho 1978), while adrenarche, the increase in serum androgens, also occurs one to two years later in children at altitude compared with sea level in Peru (Goñez et al. 1993; Gonzales and Ortiz 1994). Based on an earlier report, the Ethiopian highlanders at 3700 m appear to be the exception, as no difference was found when compared to neighboring populations at 1500 m (Harrison et al. 1969). The impact of high altitude on key determinants of reproductive health, including menarche and menopause, has been reviewed in detail elsewhere (Shaw et al. 2018). As is the case with lowland populations, highland residents are at a growing risk for a variety of noncommunicable diseases including various forms of cardiovascular diseases, cancer, chronic respiratory diseases, and diabetes mellitus. Historically, high altitude regions have been associated with decreased access to medical resources, which can affect the burden of and outcomes from these and other noncommunicable diseases, although many of these access issues may be changing as a result of increasing levels of development, migration, and greater ease of travel to and from these regions. In this section, mortality from noncommunicable diseases specific to indigenous high altitude populations is reviewed followed by a discussion of several specific noncommunicable diseases. Chronic mountain sickness, a major form of noncommunicable disease in many high altitude regions, is discussed in Chapter 24. Frequently cited work on lowlanders suggests that living at “high altitude” is associated with a lower mortality rate, in particular morality due to cardiovascular causes; however, these studies have generally examined populations living at relatively low altitudes (< 2500 m) (Baibas et al. 2005; Fabsitz and Feinleib 1980; Voors and Johnson 1979), well below that of many of the populations considered in this chapter. In regions located >3000 m and primarily populated by high altitude indigenous residents, an inverse relationship between mortality rate and altitude has not been observed. Although life expectancy at birth has increased by 14.2 years from 1990 to 2013, Tibet still has the lowest life expectancy at birth compared to all provinces in China (Zhou et al. 2016), including higher rates of death from cerebrovascular and hypertensive heart disease (but not ischemic heart disease). A similar trend is apparent in the Andes. From 1996–2000, noncommunicable disease-related deaths increased in the Peruvian Andes with the mortality rate of 892.7/100,000 population in the Andes exceeding the national average of 670.7/100,000 (Huicho et al. 2009). In Bolivia, a shorter lifespan was identified in older adults living >1500 m compared to those living below 1500 m. Importantly, income level, housing standards, and medical care access were similar, suggesting differences in environmental and physiological stressors associated with high altitude, as opposed to socioeconomic status, may contribute to the observed differences in survival (Virues-Ortega et al. 2009). While this evidence suggests an increased mortality rate in high altitude indigenous populations compared to sea-level regions in the same country, it remains unclear whether this can be attributed to high altitude or the socioeconomic challenges faced in these regions. For instance, only 44.3% and 48.9% of households in the mountain and hill regions of Nepal, respectively, had access to health facilities within 30 minutes, with the highest density of poor individuals found in the midwestern hill and mountain regions (The Nepal NCDI Poverty Commission 2018: http://www.ncdipoverty.org/nepal). While an inverse association between altitude and the prevalence of diabetes has been documented in the United States (Woolcott et al. 2014) and a lower rate of diabetes has been noted in Indian soldiers stationed between 3692 m and 5538 m (Singh et al. 1977), there is considerable heterogeneity among indigenous high altitude populations. Andeans living at 3250 m have been shown to have a lower 12-hour continuous glucose levels compared to sea-level residents (Castillo et al. 2007), but there is no consistent evidence to suggest that those living at high altitude have a lower prevalence of diabetes. In the Andes the prevalence of metabolic syndrome was 24.2% in those living at 4100 m (primarily Quechuan) compared to 22.1% at 101 m (Baracco et al. 2007) with the highest prevalence seen in those with excessive erythrocytosis (De Ferrari et al. 2014) and lower oxyhemoglobin saturation (Miele et al. 2016). Hispanic-Mestizas women have an increased prevalence of obesity and diabetes compared to Quechua women in Cusco region (2577–3570 m) (Ojeda et al. 2014). Likewise, the prevalence of diabetes was higher in the coastal region (8.2%) compared to the highlands (Huarez 3000 m; 4.5%) in Peru (Seclen et al. 2015). An important contribution to this field was made by the CRONICAS Cohort study, which was conducted in four Peruvian sites with varying degrees of altitude and urbanization: Pampas de San Juan de Miraflores, in Lima, Latin America’s fourth largest city and a highly urbanized area located at sea level; Puno, located at 3825 m above sea level, which contributed one urban and one rural site; and Tumbes, a semiurban setting in the coastal North of Peru, also at sea level (Miranda et al. 2012). Here, it was found that those living in rural Puno (3825 m) had a lower prevalence of diabetes and median Homeostatic Model Assessment of Insulin Resistance (HOMA) compared to urban and sea level populations (Carrillo-Larco et al. 2018); however, Andeans in Puno had a higher incidence of diabetes compared to those living in Lima or Tumbes (Bernabé-Ortiz et al. 2016). In the same cohort, a more traditional diet was associated with a lower prevalence of diabetes (Alae-Carew et al. 2019), suggesting the rapid nutrition transition, which refers to changes in diet and physical activity patterns that are changing body composition at a population level, may pose a particular threat to rural high altitude populations (Chaparro and Estrada 2012). The findings reported in Tibetan and Sherpa populations are equally equivocal. Several studies demonstrate increased problems among these high altitude residents. For example, the prevalence of metabolic syndrome in Tibetan farmers and herdsmen ages 30–80 living at 3700 m was 8.2% (Sherpa et al. 2013) compared to a prevalence of just 3.6% was reported in Derong County (2060–3820 m (Huang et al. 2020). Similarly, the prevalence of fasting hyperglycemia is higher in Tibetans living >3500 m compared to those living 2500–3500 m, with prevalence varying as a function of the degree of hypoxemia and polycythemia (Okumiya et al. 2016). Polycythemia was also shown to be associated with increased hemoglobin A1c (HbA1c) in Kham Tibetans living at >3600 m when compared to those with a lower HbA1c (Zhang et al. 2018a). Other studies, however, have shown different results. For example, it has been shown that Tibetans in the Changdu region living at <3500 m had an increased prevalence of diabetes mellitus and obesity when compared to Han Chinese (Xu et al. 2015). Sherpa living at high altitude had a lower prevalence of impaired glucose regulation and plasma fasting glucose compared to Sherpa living in Kathmandu (Lhamo et al. 2008). The results of this study may reflect the effects of urbanization rather than altitude. This concept is supported by evidence demonstrating that the prevalence of diabetes in Nepal is higher among those living in urban settings at 2800 m and 3620 m compared to those living in rural settings at similar elevations of 2890 m and 3270 m (Aryal et al. 2017). Of note, the prevalence of prediabetes in this study was approximately threefold greater than the Nepalese national estimate (10.3%) (Aryal et al. 2017), suggesting a possible major forthcoming public health problem. The considerable heterogeneity in the prevalence of metabolic syndrome and diabetes mellitus among high altitude indigenous populations and the variability in prevalence between groups with similar genetic ancestry living at different elevations suggest it is rapid nutritional and physical activity transitions that are placing these populations at risk of developing metabolic diseases, particularly in areas where diabetes awareness is low (Sherpa et al. 2013). This may become an increasingly common risk factor for metabolic disease with increasing urbanization and departures from traditional ways of life (Peng et al. 2019). Several forms of cardiovascular disease warrant consideration. Studies spread over many years have shown that serum cholesterol levels were low in Andean and Bhutanese high altitude natives (Jackson 1968), lower in Tibetan high altitude residents compared to a Japanese sea level population (Fujimoto et al. 1989), and lower among Nepalese living in mountain regions compared to those from a lowland region of Nepal (Aryal et al. 2015). The CRONICAS cohort study has also shown that those in rural Puno had the highest prevalence of ideal cardiovascular health, defined as the presence of ideal health factors (untreated blood pressure <120/<80 mmHg, untreated total cholesterol <200 mg/dL, and untreated fasting plasma glucose <100 mg/dL) and ideal health behaviors (never smoker, body mass index [BMI] <25 kg/m2, moderate physical activity ≥150 min/week, and high adherence to components of the Dietary Approach to Stop Hypertension diet) (Benziger et al. 2018). The key question is whether such differences are associated with a decreased risk of atherosclerotic vascular disease. Evidence on this issue is limited. Early studies of populations in the Andes, for example, suggested that both coronary artery disease (CAD) and myocardial infarction were uncommon among high altitude residents. For example, no cases were found in one series of 300 necropsies carried out at 4300 m, and epidemiological studies in South America have shown that both angina of effort and electrocardiographic (ECG) evidence of myocardial ischemia are less at high altitude than at sea level (Ramos et al. 1967). As was the case with diabetes mellitus, there may also be a urban-rural divide in terms of stroke in the Andes, as the prevalence was higher in central area of Cusco, where residents are more sedentary and have a higher caloric intake compared to the peripheral area where the standard of living is lower (Serradj et al. 1995). In the Tibetan ethnic population of northern Bhutan, no autopsy studies were available but angina seemed uncommon, and, as judged by ECG recordings, evidence of coronary artery disease was minimal. In Qinghai, CAD was common and autopsies on Tibetans showed the same incidence as in lowlanders, although the incidence of coronary infarction was low (Sun 1986). One important factor which may affect the incidence of atherosclerotic disease is exposure to biomass for cooking and heating. Data from the CRONICAS study, for example, have shown that the incidence of carotid plaque and mean and maximum carotid intima/media thickness were increased in those chronically exposed to biomass fuels despite a higher cardiovascular disease burden in those using clean fuel (Painschab et al. 2013). Similarly, among Sherpa living in Chaurikharka (2562 m), indoor air pollution correlated with total peripheral resistance and arterial stiffness (pulse-wave velocity) (Pratali et al. 2019). The prevalence of hypertension varies between the high altitude regions of the world. Among Andeans, the prevalence of hypertension is low among otherwise healthy individuals (Baker 1978; Siqués et al. 2009; Toselli et al. 2001), but elevated among those with chronic mountain sickness (Corante et al. 2018). Although there is no longer a complete absence of hypertension in the Andes, blood pressure in Andeans is often lower than sea-level residents (Corante et al. 2018; Tremblay et al. 2019). In the CRONICAS cohort study, for example, prevalence of hypertension in rural and urban populations in Puno (13.1% in Bernabe study, 11.2% and 10.5% in Miranda) is much lower than Lima and Tumbes (23.5% in Bernade, 19.8% and 26.1% in Miranda) (Bernabé-Ortiz et al. 2017; Miranda et al. 2019). Further evidence from this study suggested that the lower prevalence of hypertension was associated with more traditional diet (Alae-Carew et al. 2019). In contrast to the Andes, a high prevalence of hypertension has been noted in diverse Tibetan populations (Huang et al. 2020; Zhang et al. 2018a), which may correlate with the altitude of residence (Mingji et al. 2015). For example, both older and more recent reports note a higher prevalence of hypertension among Tibetan highlanders than among Chinese lowlanders who had migrated to the region (Chen et al. 2010; Sherpa et al. 2013; Sun 1986). Similarly, in a study of 701 adults ages 40–89 living at 4300 m (Zhao et al. 2012), high rates of hypertension were found in Tibetan men and women; 56% of subjects had blood pressure over 140/90 mmHg, of which 61% had blood pressure of over 160/100 mmHg. This high prevalence of hypertension is noteworthy since ∼95% of the population studied had an active lifestyle working as herdsmen/women. Other studies, however, have found contradictory findings. A 15-year survey of 7797 male and 8029 female Tibetan native highlanders living on the Tibetan plateau demonstrated only a 2% prevalence of hypertension compared to over 4% among Chinese immigrants to Tibet (Wu 1994). The available data from Ethiopia suggest that the prevalence of hypertension is relatively low among the highlanders in comparison to the overall population (Beall et al. 1997; Cheong et al. 2017; Gebreyes et al. 2018) Ethiopian highlanders tend to demonstrate low blood pressures and prevalence of hypertension. In 1965–66, as part of the International Biological Programme, Harrison et al (1969) compared blood pressures in Ethiopians (Simien mountains, mostly Amhara) living at 1500 m, 3000 m, and 3700 m. Ethiopian males living in Debark (3000 m; 123/77 mmHg) had higher systolic blood pressure (SBP) than those living at 1500 m (119/76 mmHg), but no difference in diastolic blood pressure (DBP) or in those living at 3700 m (121/80 mmHg). Females were only examined at 1500 m (115/76 mmHg) and 3000 m (119/74 mmHg) and did not present any differences in blood pressure between sites. A reanalysis of the same data indicated that socioeconomic status may impact blood pressure as residents of 3700 m had higher DBP than the lower socioeconomic groups living at 1500 m and 3000 m (Clegg et al. 1976). No cross-sectional age-related increase in blood pressure was apparent in this population. Some cases of cardiovascular diseases were encountered during clinical work as part of the expedition; however, this was not elaborated upon. The majority of noncommunicable diseases were of the alimentary system (Harrison et al. 1969). Beall et al. (1997) observed lower blood pressures (male: 109/75 mmHg, female: 106/74 mmHg) than those reported by Harrison and Clegg in Amhara at 3530 m with no age-related increase in blood pressure (from ages 14–86 years); nine of 231 (4%) participants were classified as hypertensive. More recently, no difference in blood pressure was detected between low and high altitude in Ethiopia, but Amhara who live at high altitude (3700 m) had lower DBP compared to Oromo at high altitude (4000 m) (Cheong et al. 2017). Based on the individual data presented, no Amhara or Oromo appeared to have SBP >140 mmHg and very few had DBP >90 mmHg (∼2% of Amhara at 3700 m and Oromo at 4000 m). Thus, despite limited available data, Ethiopian highlanders appear to present very low levels of hypertension compared to overall prevalence of 15.8% across all of Ethiopia (Gebreyes et al. 2018). Without direct, well-controlled comparisons between high altitude populations, it is difficult to sort out why variations exist in the prevalence of hypertension across groups, but several factors warrant consideration. One potential explanation for the differences in the prevalence of hypertension between high altitude populations may be differences in diet. A key difference between Tibetans and Andeans, for example, is the consumption of Tibetan tea, which is made with salt and yak butter and, as a result, is associated with a high sodium intake. Older reports, for example, have noted that some Tibetans and Sherpa drank 20–30 cups of this tea per day (Sehgal et al. 1968) and consume 4–7 g of sodium on a daily basis (Goldstein and Beall 1990; Smith 1999), far higher than the recommended intake in published guidelines (Government of Canada). Sherpa with SBP ≥140 mmHg consumed a greater amount of Tibetan tea compared to those with SBP <140 mmHg. In a patient-blinded randomized controlled trial, a low-sodium, high-potassium, salt-substitute intervention was effective in lowering SBP and DBP by 8.2 mmHg and 3.4 mmHg, respectively, in Tibetans living at 4300 m (Zhao et al. 2014). Another factor that may account for differences is relative exposure to pollution from biomass fuels. Data from the CRONICAS cohort study, for example, showed that daily users of biomass fuel were more likely to be prehypertensive and had higher blood pressure on average (Burroughs Peña et al. 2015). In addition, interventions to reduce household air pollution, including improved cookstoves, reduced systolic blood pressure from 115 ± 13 mmHg to 109 ± 10 mmHg in Quechuan women living in rural Bolivia (3000–3350 m) (Alexander et al. 2015). Congenital cardiovascular malformations are common at altitude, with patent ductus arteriosus being 15 times more common at Cerro de Pasco (4200 m) than at sea level in Lima (Peñaloza et al. 1964). Marticorena et al. (1959) reported an incidence of 0.72% of patent ductus arteriosus in children born around 4300 m, compared with an incidence of 0.8% for all congenital heart disease at sea level. A recent large-scale (N = 84,303) cross-sectional study, including clinical screening and echocardiography, has revealed a strong correlation between congenital heart disease and increased altitude among different schools in Nagqu (Chun et al. 2018). The most common congenital heart disease type was patent ductus arteriosus (66.3%) followed by atrial septal defect (20.3%) and ventricular septal defect (9.1%). Studies have shown that genetic phenotypes likely interact with factors related to the intrauterine hypoxic environment and result in an increased risk of congenital heart disease and high altitude (Pan et al. 2018; Sergi 2019; Yang et al. 2019). Decreased arterial PaO2 after birth may play a role in maintaining patency of the ductus arteriosus. One of the challenges associated with congenital heart disease and high altitude residence is the appropriate means of screening newborns for these problems. While pulse oximetry is now a standard approach for screening for critical congenital heart disease at low elevation (Kemper et al. 2011), concerns have been raised about the utility of this approach at high altitude due to an increased risk of false positives related to ambient hypoxia, as well as variation in the physiological responses to hypoxia across high altitude populations (Hoffman 2016; Tekleab and Sewnet 2019). Different cut points for identifying newborns who require further evaluation with echocardiography may be required based on the altitude of residence and ethnic background (González-Andrade et al. 2018). Despite the permanent stress of hypoxic exposure, humans populating high altitude areas have reduced cancer mortality over a broad spectrum of cancer types (reviewed in: Merrill and Frutos 2019; Thiersch and Swenson 2018; Thiersch et al. 2017). A number of reasons have been proposed for these observations, including the physiological changes associated with adaptation to higher altitudes, increased physical activity, better dietary habits, lower risk factors for CAD, and environmental factors such as ultraviolet light exposure, vitamin D, and exposure to air pollution. While these data applies to all individuals living at high altitude, cancer data specific to indigenous high altitude populations at high altitude is scarce. In the Andes, there is an association between altitude and gastric cancer incidence and mortality (Chirinos et al. 2012; Torres et al. 2013); however, this may not be specific to high altitude, but rather factors that cluster in mountainous regions including genetic determinants, Helicobacter pylori exposure, dietary factors, socioeconomic status, and environmental exposures. Lung cancer did not differ between study sites for the CRONICAS cohort (Miranda et al. 2019). Gastric cancer is also one of the most common malignancies in Tibet and this may be associated with HIF-1α gene polymorphisms (Li et al. 2009). Despite this, cancer is not one of the 10 leading causes of death in Tibet (Zhou et al. 2016). While data are available documenting the prevalence of COPD in countries with resident high altitude populations (Adhikari et al. 2018; Zhou et al. 2016), more specific information about prevalence in high altitude regions themselves is very limited. Data from the CRONICAS cohort study documented a prevalence of 6.2% and 9.9% in urban and rural Puno, Peru (3820 m), respectively, while another report noted a prevalence of 36% among Krygyz highlanders residing at 2050 m (Brakema et al. 2019). Data from other high altitude regions are not available. Some studies have shown evidence of a weak correlation between altitude of residence and the prevalence of COPD (Laniado-Laborin et al. 2012) and that accelerated rates of lung function decline are associated with high altitude residence (Miele et al. 2018), but a pooled analysis of data from multiple studies did not find evidence of a relationship between altitude and prevalence of COPD (Horner et al. 2017). This study did, however, demonstrate that increasing altitude was associated with an increased risk of undiagnosed COPD, which could be related to difference in access to medical care. One important fact that may account for development of COPD in high altitude regions despite relatively low tobacco consumption is exposure to household air pollution from burning of biomass, a common problem across many rural high altitude regions. This has been shown in multiple studies to be associated with increased risk for COPD in low and middle income countries (Brakema et al. 2019; Kurmi et al. 2010; Siddharthan et al. 2018), although some more recent data have called this relationship into question (Amaral et al. 2018). Concerns have been raised about some of the methodological limitations of the studies on this question, including the utility of cross-section versus longitudinal designs, as well as the fact that a key variable in these studies, biomass exposure, is often measured based on self-report of solid fuel use and, therefore, subject to the effects of recall bias (Balmes and Eisen 2018). To the extent that it does affect the risk of COPD, public health interventions designed to improve access to safer heating and cooking systems can reduce the burden of lung disease among high altitude residents. Whereas these were formerly major problems in high altitude regions throughout the world, initiation of universal salt iodization in 1993 has significantly decreased the incidence of iodine deficiency (Tamang et al. 2019a; 2019b) and the incidence of goiter and other disorders of iodine deficiency (Ministry of Health and Population et al. 2007). Despite this, goiter remains a major public health problem, especially in developing regions of Ethiopia with a reported prevalence of 62% (Tigabu et al. 2017). Further details on iodine deficiency and goiter are outlined in Chapter 16. High altitude residents are at risk for many of the same communicable diseases seen at lower elevations such as rabies, viral hepatitis, measles, chronic eye infections, and diarrheal illness. Compared to the extensive body of research on physiologic responses to hypoxia in unacclimatized lowlanders and high altitude residents, there is a relative paucity of evidence regarding the effect of high altitude exposure on innate defense against microbial infection as well as a paucity of data comparing the risk of communicable disease between high and low altitude regions. Even in cases where risk may vary, these differences might be related less to the hypobaric hypoxia at high altitude and more to other factors such as differences in access to public health resources and medical care, as well as local living conditions. Data regarding several forms of communicable disease for which evidence is available are presented in the next few sections. Mycobacterium tuberculosis is endemic to many countries with long-term high altitude populations including Ethiopia, Nepal, Peru, and Tibet, among others. A consistent observation across studies from multiple regions of the world is that there is a negative relationship between altitude and the prevalence and incidence of pulmonary tuberculosis and positive tuberculin skin tests (TST), such that these variables, along with mortality related to tuberculosis, decrease with increasing elevation (Mansoer et al. 1999; Olender et al. 2003; Perez-Padilla and Franco-Marina 2004; Saito et al. 2006; Vargas et al. 2004). For example, Olender et al. (2003) compared the prevalence of positive TST in two high altitude villages located at 3340 m and 3500 m with three sea-level sites in Peru and found lower TST-positive prevalence in the high altitude villages (5.7% and 6.8%) than in the sea-level areas (25–33%). The mechanism for these observed effects of altitude on transmission of and mortality from tuberculosis, which appears to be present at even relatively modest elevations of 750–1000 m (Mansoer et al. 1999; Vargas et al. 2004), is not entirely clear. One potential explanation may be the fact that many high altitude regions lack the population density and crowding of low elevation cities, which contributes to the spread of this disease. In fact, when Saito et al. (2006) compared urban highland (≥3000 m) and urban nonhighland (<3000 m) communities, there were no significant differences in the prevalence of positive TST, whereas significant differences were present between the rural highland and rural lowland communities, thereby suggesting that crowding can overcome any beneficial effect of high altitude. A variety of other factors have also been proposed. For example, it was established long ago that reductions in arterial P
Introduction
Adaptation
Brief History of Research on Long-Term Residents of High Altitude
Duration of Exposure in High Altitude Residents
Differences in Phenotype Between Highland Populations
Hematological
Hemoglobin Concentration
Oxygen Affinity
Respiratory
Ventilation
Hypoxic Ventilatory Response
Oxygen Saturation
Nocturnal Periodic Breathing
Circulatory
Systemic Circulation
Cerebral Circulation
Pulmonary Circulation
Skeletal muscle and metabolism
Exercise
Other Health Issues in High Altitude Residents
Pregnancy and development
Birth Weight
Placental Development
Birth Defects
Childhood Growth and Development
Menarche
Noncommunicable diseases
Mortality Related to Noncommunicable Disease
Diabetes Mellitus
Cardiovascular Disease
Atherosclerosis, coronary artery disease, and stroke
Hypertension
Congenital heart disease
Cancer
Chronic Obstructive Pulmonary Disease (COPD)
Iodine Deficiency and Goiter
Communicable diseases
Tuberculosis
Stay updated, free articles. Join our Telegram channel

Full access? Get Clinical Tree
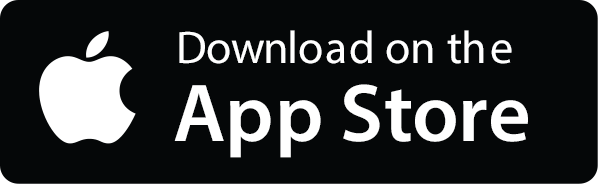
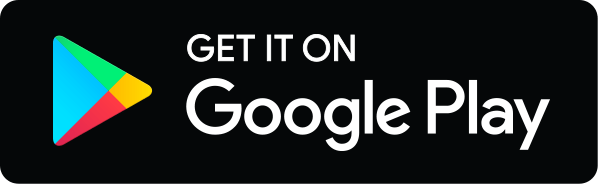