Background
Supine bicycle exercise (SBE) echocardiography and treadmill exercise (TME) echocardiography have been used for evaluation of coronary artery disease (CAD). Although peak imaging acquisition has been considered unfeasible with TME, higher sensitivity for the detection of CAD has been recently found with this method compared with post-TME echocardiography. However, peak TME echocardiography has not been previously compared with the more standardized peak SBE echocardiography. The aim of this study was to compare peak TME echocardiography, peak SBE echocardiography, and post-TME echocardiography for the detection of CAD.
Methods
A series of 116 patients (mean age, 61 ± 10 years) referred for evaluation of CAD underwent SBE (starting at 25 W, with 25-W increments every 2–3 min) and TME with peak and postexercise imaging acquisition, in a random sequence. Digitized images at baseline, at peak TME, after TME, and at peak SBE were interpreted in a random and blinded fashion. All patients underwent coronary angiography.
Results
Maximal heart rate was higher during TME, whereas systolic blood pressure was higher during SBE, resulting in similar rate-pressure products. On quantitative angiography, 75 patients had coronary stenosis (≥50%). In these patients, wall motion score indexes at maximal exercise were higher at peak TME (median, 1.45; interquartile range [IQR], 1.13–1.75) than at peak SBE (median, 1.25; IQR, 1.0–1.56) or after TME (median, 1.13; IQR, 1.0–1.38) ( P = .002 between peak TME and peak SBE imaging, P < .001 between post-TME imaging and the other modalities). The extent of myocardial ischemia (number of ischemic segments) was also higher during peak TME (median, 5; IQR, 2–12) compared with peak SBE (median, 3; IQR, 0–8) or after TME (median, 2; IQR, 0–4) ( P < .001 between peak TME and peak SBE imaging, P < .001 between post-TME imaging and the other modalities). ST-segment changes in patients with CAD and normal baseline ST segments were higher during TME (median, 1 mm [IQR, 0–1.9 mm] vs 0 mm [IQR, 0–1.5 mm]; P = .006). The sensitivity of peak TME, peak SBE, and post-TME echocardiography for CAD was 84%, 75%, and 60% ( P = .001 between post-TME and peak TME echocardiography, P = .055 between post-TME and peak SBE echocardiography), with specificity of 63%, 80%, and 78%, respectively ( P = NS) and accuracy of 77%, 77%, and 66%, respectively ( P = NS). Peak TME echocardiography diagnosed multivessel disease in 27 of the 40 patients with stenoses in more than one coronary artery, in contrast to 17 patients with peak SBE imaging and 12 with post-TME imaging ( P < .05 between peak TME imaging and the other modalities). Image quality was similar with the three techniques. The duration of the test was longer with SBE echocardiography (9.5 ± 3.8 vs 7.6 ± 2.5 min, P < .001).
Conclusions
During TME and SBE, patients achieve similar double products. Ischemia is more extensive and frequent with peak TME, which makes peak TME a more valuable exercise echocardiographic modality to increase sensitivity. However, peak SBE should be preferred to TME if the latter is performed with postexercise imaging acquisition.
Exercise is the recommended modality for patients referred to stress echocardiography who are able to exercise. Among the exercise echocardiographic techniques, treadmill exercise (TME) echocardiography and supine bicycle exercise (SBE) echocardiography are used worldwide. Peak imaging acqui-sition with SBE is feasible and has higher sensitivity than postexercise echocardiography with either SBE or TME. However, SBE achieves less oxygen consumption, and the ischemic threshold has been found to be lower with this technique compared with TME. Although peak TME echocardiography has been considered unfeasible in the past, several reports have shown that feasible images can be obtained at peak TME. Furthermore, peak TME echocardiography has been found to be more sensitive for the detection of coronary artery disease (CAD) than the traditional post-TME approach and also more sensitive for the prediction of multivessel CAD. However, a direct comparison between peak TME echocardiography and the more standardized peak SBE echocardiography for patients with known or suspected CAD has not been performed thus far. Therefore, this study was undertaken prospectively to compare the sensitivity and specificity of peak TME, peak SBE, and post-TME echocardiography for detecting CAD in the same patients.
Methods
Patient Population
The study group consisted of patients with known or suspected CAD who underwent or were likely to undergo coronary angiography within 30 days of the exercise studies and were considered to be able to exercise using both treadmill and bicycle. Patients were included from January 2009 to March 2011. Patients referred to the cardiology unit for angiography were invited to participate. Most of the exercise echocardiographic studies were performed before coronary angiography ( n = 65), although some were performed after the angiographic procedures ( n = 31). Also, some patients with typical angina or probable angina and several coronary risk factors who were referred for exercise echocardiography were recruited ( n = 27), although seven were finally excluded because angiography was not performed. Coronary angiography was exclusively ordered by the clinicians responsible for the patients. Patients were also excluded if they had recent myocardial infarctions (4 weeks), were in unstable clinical conditions or had moderate or severe valvular lesions, or were in New York Heart Association class II or higher. The institutional review board approved the study protocol. All patients gave informed consent before testing. Clinical data were obtained from review of medical records and patient interviews before the tests.
Exercise Testing with SBE and TME
All patients underwent both SBE and TME testing. The two tests were performed ≥24 hours apart (within 1 week), and both were symptom limited. The order of the stress modalities was randomized. Heart rate and blood pressure were measured and electrocardiograms were obtained at baseline and at each stage of the exercise protocol with both methods. Patients were encouraged to perform maximal exercise testing. Exercise end points included physical exhaustion, significant arrhythmia, severe hypertension (systolic blood pressure > 240 mm Hg or diastolic blood pressure > 110 mm Hg), or severe hypotensive response (decrease > 20 mm Hg). Ischemic electrocardiography (ECG) findings were defined as the development of ST-segment deviation ≥ 1 mm that was horizontal or sloping away from the isoelectric line 80 msec after the J point, in patients with normal baseline ST segments. ECG results were considered nondiagnostic in the presence of left bundle branch block, preexcitation, paced rhythm, repolarization abnormalities, or treatment with digoxin. Positive exercise test results were defined as chest pain during the test and/or ischemic ECG abnormalities in patients with diagnostic ECG findings. The same team performed both SBE and TME studies.
SBE and TME Echocardiography
Echocardiography was performed using a Vivid 5 ultrasound system with a 2.5-MHz transducer (GE Vingmed Ultrasound AS, Horten, Norway). For SBE, a cycle ergometer bed was used (Ergoline; GE Healthcare, Milwaukee, WI), with a capability of tilt to the left lateral position, as needed, to a maximum of 30° and head elevation to a maximum of 20° to optimize images. Patients pedaled at a constant speed beginning at a workload of 25 W and increasing by 25 W every 2 to 3 minutes. Echocardiography was performed in three apical views (long axis, four chamber, and two chamber) and two parasternal views (long and short axis) at baseline and at peak exercise with both SBE and TME, as well as after TME (<60 sec after the end of the exercise). For TME, the protocols used were Bruce in 106 patients and modified Bruce in 10 patients. Peak imaging with TME and SBE was performed with the patient still exercising, when signs of exhaustion were present or an end point was achieved, as previously described. Patients were asked to notify us about 1 min before exhaustion to allow for peak imaging acquisition with either TME or SBE. Once the left ventricular apex was localized in one apical view, an attempt was made to maintain the transducer in the same position to obtain all three apical views by rotating the transducer with the fingers of the examiner, without changing the position of the transducer. The same approach was used for parasternal imaging acquisition. In the case of TME, the patient was maintained between the transducer and the left hand of the examiner, applying some pressure on the patient’s back to minimize body and respiratory movements. Patients running at peak exercise were asked to walk rapidly during the time of peak imaging acquisition. Post-TME echocardiography was performed with the patient on the table bed in the left lateral decubitus position. For the three methods, a continuous harmonic imaging acquisition system was used. Resting and peak images were chosen for comparison in a quad-screen format.
Echocardiographic Analysis
Regional wall motion abnormalities (WMAs) were evaluated using a 16-segment model of the left ventricle. Each segment was graded using a four-point scale (1 = normal, 2 = hypokinetic, 3 = akinetic, and 4 = dyskinetic). However, isolated hypokinesia of the inferobasal or septal basal segment were not considered abnormal. A normal response was characterized by normal resting function, with no deterioration induced by exercise. Necrosis was defined as a resting WMA without change with exercise. Ischemia was defined as the development of new or worsening WMAs with exercise. Positive test results were defined as ischemia or necrosis. Wall motion score index (WMSI) and visually estimated left ventricular ejection fraction were calculated at rest and at exercise. WMSI was calculated as the sum of scores divided by the number of visualized segments. The change in WMSI from rest to exercise (ΔWMSI) was calculated. Myocardial segments were combined into vascular territories to express the extent of WMAs as one-vessel, two-vessel, or three-vessel CAD. The apex and anteroseptal, septal, and anterior walls were ascribed to the left anterior descending coronary artery, the lateral and inferolateral walls to the left circumflex artery, and the inferior and inferoseptal walls to the right coronary artery. Multivessel CAD was defined as exercise WMAs in more than one territory. All studies were analyzed by one expert observer unaware of the patients’ clinical characteristics, clinical and ECG responses to exercise, test modalities, and coronary angiographic results. In case of doubt, a second observer was required. Interobserver and intraobserver variability was assessed by reading 17 and 75 randomized recordings, respectively, using each method (peak TME, peak SBE, and post-TME echocardiography). For intraobserver variability, the second reading was performed ≥2 months after the first reading. Image quality was quantitatively and qualitatively analyzed. For quantitative analysis, we assessed the number of segments visualized in each view and the total number of nonvisualized segments per test. For qualitative analysis, studies were considered to be of excellent, good, poor, or bad quality. Excellent imaging quality was defined as complete visualization of all segments in the apical and parasternal views with clean assessment of endocardial border excursion and systolic thickening. Good quality was defined as complete visualization of all segments in the apical views with good assessment of endocardial border excursion and systolic thickening but incomplete assessment of the endocardial border excursion or thickening in at least one segment in the parasternal views. Poor quality referred to a lack of assessment of the endocardial border excursion or thickening in one segment in the different views. Bad quality was defined as a lack of assessment of endocardial border excursion or thickening in two or more segments in the different views.
Quantitative Coronary Angiography
Coronary angiography was performed in multiple projections using the standard Judkins technique. Angiographic studies were performed within 4 weeks of study enrollment. There were no myocardial infarctions or revascularization procedures between the exercise testing studies and coronary angiography. An independent experienced observer quantified the coronary angiograms (Excelera Pack; Philips Medical Systems, Best, The Netherlands). A significant coronary stenosis was defined as ≥50% narrowing of the reference luminal diameter.
Statistical Analysis
Continuous variables are reported as mean ± SD, and differences were assessed using paired Student’s t tests. Noncontinuous variables are reported as medians and interquartile ranges (IQRs), and differences were assessed using Wilcoxon’s tests. Categorical variables are reported as percentages, and comparisons between groups were based on χ 2 tests. Kappa statistics were used to evaluate concordance in imaging interpretation. Sensitivity and specificity were evaluated using standard definitions. P values < .05 were considered statistically significant.
Results
Clinical Characteristics and Exercise Echocardiography
The clinical characteristics and hemodynamic response to exercise are listed in Tables 1 and 2 .
Variable | Value |
---|---|
Men | 98 (84.5%) |
Age (y) | 61 ± 12 |
Current smokers | 28 (24%) |
Diabetes | 24 (21%) |
Hypertension | 72 (62%) |
Hypercholesterolemia | 68 (59%) |
History of CAD | 63 (54%) |
Prior myocardial infarction | 47 (40.5%) |
Prior coronary revascularization | |
PCI | 37 (32%) |
CABG | 4 (3%) |
Chest pain | |
Typical angina | 15 (13%) |
Atypical/probable angina | 44 (38%) |
Nonanginal chest pain | 13 (11%) |
Dyspnea | 2 (2%) |
Asymptomatic | 42 (36%) |
Atrial fibrillation | 5 (4%) |
Left bundle branch block or paced rhythm | 5 (4%) |
Abnormal resting ECG findings | 26 (22%) |
Medications ∗ | |
β-blockers | 15 (13%) |
Calcium channel blockers | 15 (13%) |
Nitrates | 36 (31%) |
ACE inhibitors/ARAs | 59 (51%) |
Variable | SBE | TME | P |
---|---|---|---|
Systolic blood pressure (mm Hg) | |||
Rest | 135 ± 24 | 128 ± 20 | .001 |
Peak | 180 ± 36 | 159 ± 31 | <.001 |
Heart rate (beats/min) | |||
Rest | 76 ± 14 | 79 ± 15 | .002 |
Peak | 124 ± 24 | 143 ± 25 | <.001 |
Rate-pressure product (mm Hg · beats/min) | |||
Rest | 10,218 ± 2,674 | 10,102 ± 2,525 | .62 |
Peak | 22,399 ± 6,201 | 23,012 ± 7,138 | .30 |
Submaximal test | 77 (66%) | 37 (32%) | <.001 |
Metabolic equivalents | 6.6 (5.2–7.1) | 9.7 (7–11) | <.001 |
Angina during the test | 22 (19%) | 34 (29%) | .06 |
Positive ECG findings | 32 (28%) | 38 (33%) | .39 |
Positive exercise test results | 44 (38%) | 54 (47%) | .18 |
Accuracy of Peak SBE, Peak TME, and Post-TME Echocardiography
Of the 116 patients studied, 23 had resting WMAs during TME and 22 during SBE, and 75 had significant CAD. The number of patients with exercise WMAs was 63 with peak TME imaging, 56 with peak SBE imaging, and 45 with post-TME imaging ( P = .001 between peak and post-TME imaging, P = .055 between peak SBE and post-TME imaging). Sensitivity for patients without resting WMAs was 79% for peak TME, 66% for peak SBE, and 46% for post-TME echocardiography ( P < .05 between post-TME imaging and the other modalities). In the 40 patients with multivessel disease, results on peak TME echocardiography were positive in 38 patients, on peak SBE imaging in 32, and on post-TME imaging in 29 ( P < .05 between peak TME imaging and the other modalities). Among them, peak TME echocardiography detected multiple-territory involvement in 27 patients, peak SBE imaging in 17, and post-TME imaging in 12 ( P < .05 between peak TME imaging and the other modalities). In the 35 patients with single-vessel CAD, peak TME echocardiographic findings were abnormal in 25 patients, peak SBE findings in 24, and post-TME findings in 16 ( P < .05 between post-TME imaging and the other modalities). The median numbers of involved territories in these 35 patients were 3 (IQR, 0–7) by peak TME imaging, 3 (IQR, 0–6) by peak SBE imaging, and 0 (IQR, 0–3) by post-TME imaging ( P < .01 between post-TME and the two peak imaging modalities). Peak TME echocardiographic results were negative in 10 of these 35 patients, correctly showed one-vessel disease in 14, and suggested multivessel disease in 11. Corresponding figures were 11, 14, and 10 for peak SBE imaging and 19, 11, and 5 for post-TME imaging. Among the 12 patients with only intermediate lesions (50%–70%), peak TME echocardiographic results were positive in eight, peak SBE results in six, and post-TME results in three. A total of 41 patients had normal coronary arteries or nonsignificant CAD (<50%). Peak TME, peak SBE, and post-TME echocardiographic results were normal in 26, 33, and 32 of these patients, respectively ( P = NS). ST-segment changes in patients with CAD and normal baseline ST segments were higher during TME (median 1 mm [IQR, 0–1.9 mm] vs 0 mm [IQR, 0–1.5 mm]; P = .006). Figure 1 shows graphically the sensitivity, specificity, and accuracy of the three methods, and Table 3 shows the ischemic burden with the three methods. Figure 2 and Videos 1 and 2 (view video clips online) show the peak TME and peak SBE studies in two patients with CAD.
Variable | Coronary angiographic results | |||||
---|---|---|---|---|---|---|
Normal | Abnormal | |||||
Peak TME | Peak SBE | Post-TME | Peak TME | Peak SBE | Post-TME | |
WMSI | ||||||
Rest | 1.0 (1.0–1.0) | 1.0 (1.0–1.12) | — | 1.0 (1.0–1.09) | 1.0 (1.0–1.13) | — |
Peak | 1.0 (1–1.41) ‖ | 1.0 (1.0–1.0) | 1.0 (1.0–1.0) | 1.45 (1.13–1.75) ∗,‡ | 1.25 (1.0–1.56) § | 1.13 (1.0–1.38) |
Left ventricular ejection fraction | ||||||
Rest | 59 ± 6 | 60 ± 7 | — | 58 ± 6 | 57 ± 6 | — |
Peak | 63 ± 10 ‖ | 66 ± 10 | 67 ± 9 | 55 ± 11 ∗,† | 58 ± 10 § | 61 ± 9 |
Number of abnormal segments during exercise | 0 (0–5.5) ‖ | 0 (0–0) | 0 (0–0) | 5 (2–12) ∗,‡ | 3 (0–8) § | 2 (0–4) |
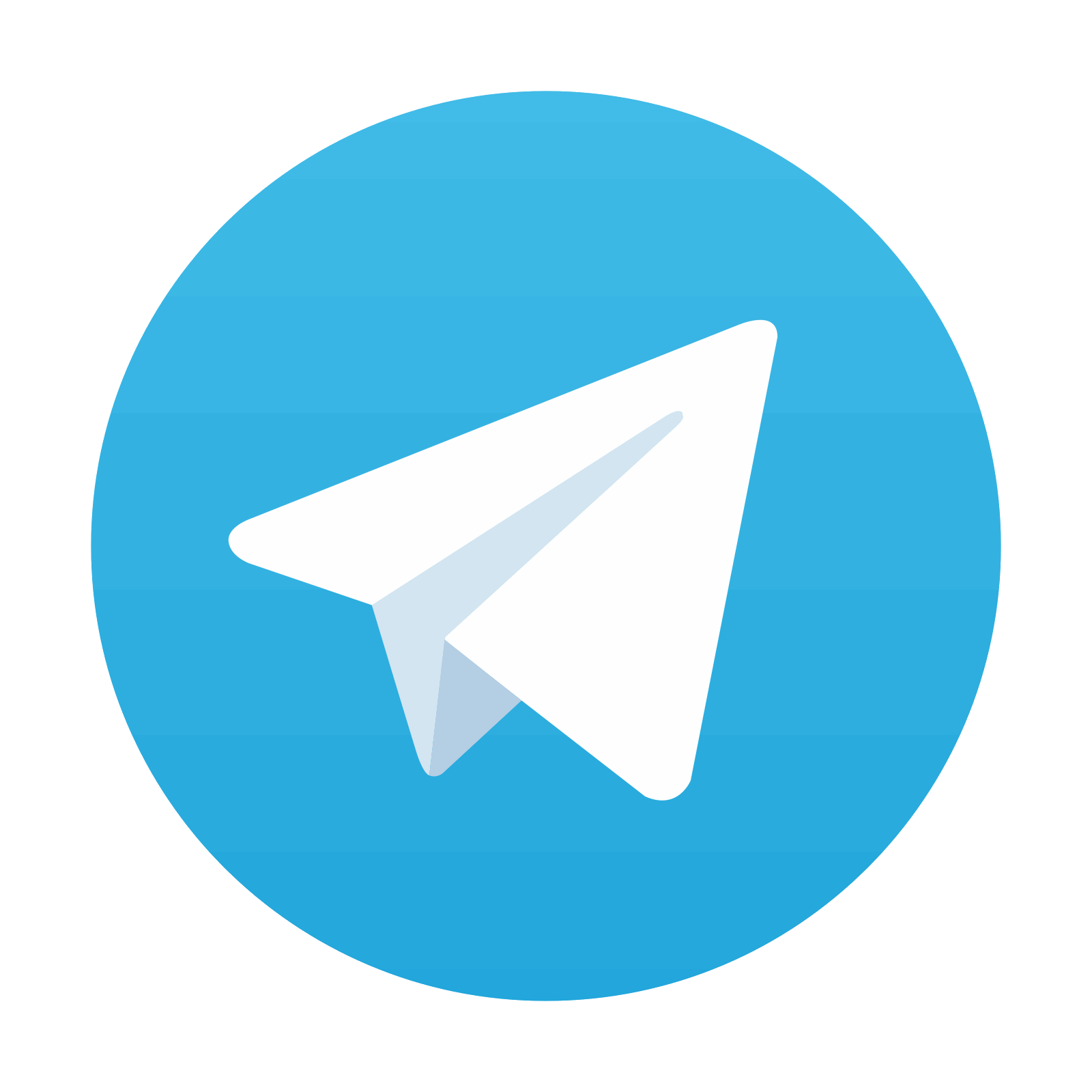
Stay updated, free articles. Join our Telegram channel

Full access? Get Clinical Tree
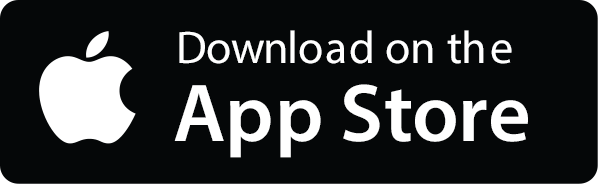
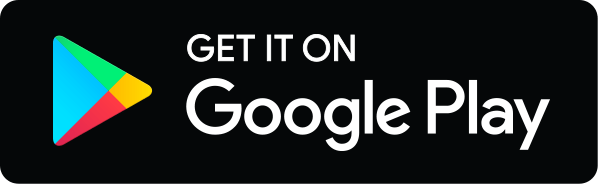
