Genetics of Fetal Arrhythmias
Susan Etheridge
Lindsay Meyers
INTRODUCTION
The fetus of a normal pregnancy was delivered emergently by cesarean delivery at 31 weeks’ gestation for bradycardia which was thought to be caused by fetal distress. At birth, the infant’s Apgar scores were 9 and 9, cord blood pH was 7.27, and the base excess was −5. The 12-lead electrocardiogram (ECG) demonstrated 2:1 atrioventricular (AV) block due to severe QT prolongation (FIG. 1.3.1A), and syndactyly of the fingers on both hands was noted (FIG. 1.3.1B), which are all features of long QT syndrome (LQTS) 8, a channelopathy also known as Timothy syndrome. The infant was intubated due to lung disease of prematurity and numerous episodes of torsades de pointes (TdP) (FIG. 1.3.1C). He ultimately succumbed to complications of prematurity.
The cardiac channelopathies are but one of the inherited arrhythmia disorders. They are caused by disturbed function of cardiac ion channel subunits or the proteins that regulate them and include LQTS, short QT syndrome, Brugada syndrome (BrS), catecholaminergic polymorphic ventricular tachycardia (CPVT), early repolarization syndrome, and some forms of inherited sinus bradycardia. The inherited arrhythmia disorders may be suspected from a detailed family or obstetrical history, supported by features of the current pregnancy including heart rhythm analysis of the fetus and confirmed by genetic testing. While genetic testing for aneuploidy and other chromosome anomalies has become routine in obstetrical practice, and despite availability of gene mutation panels for inherited arrhythmias at commercial laboratories, genetic testing for inherited arrhythmias is vastly underutilized. Given the prevalence of inherited channelopathies, this is an important oversight. By way of comparison, trisomy 21 occurs in 1/700 live births1 and the prevalence of the most common inherited channelopathy, LQTS, is 1/2000 individuals.2
The purpose of this chapter is to outline the fetal presentation of the inherited arrhythmias. We will review current findings of disease inheritance and genetic disease modifiers. Since the most is known about LQTS, we will describe not only the three most common types but also the specific and unique clinical aspects of more uncommon but unique and highly lethal forms including Timothy syndrome and Jervell and Lange-Nielsen syndrome. This is followed by other inherited arrhythmias relevant to the fetus including CPVT, the calmodulinopathies, BrS, and channelopathies of the sinoatrial node resulting in sinus bradycardia. Management of the pregnant mother with channelopathy is discussed in Part 5, Chapter 1, and treatment of the symptomatic fetus with channelopathy is discussed in Part 3, Chapter 6.
LONG QT SYNDROME (LQTS)
LQTS is an inherited disease of cardiac repolarization characterized by a prolonged QTc interval on the ECG (FIG. 1.3.2). LQTS is usually the result of single pathogenic variant in one of the genes that encode for a cardiac ion channel or an accessory ion channel subunit. These single variants can cause life-threatening arrhythmias, seizures, syncope, and sudden death in heterozygous mutation carriers.3 The clinical events are a consequence of the signature ventricular arrhythmia, TdP, that can terminate spontaneously or degenerate into ventricular fibrillation (FIG. 1.3.1C). Once recognized, primary prevention with beta-adrenergic blocking agents is highly successful; therefore, early recognition can save lives.
Fetal Presentation
Ideally, LQTS would be diagnosed before birth and primary prevention initiated during infancy, but unless LQTS is suspected because of fetal arrhythmia or a family history, prenatal diagnosis is difficult. One should have a high index of suspicion in the fetus with ventricular tachycardia and/or 2:1 AV block, as the combination of the two arrhythmias is highly suggestive of LQTS. However, these LQTS rhythms occur in <25% of fetal LQTS cases, and the most common presentation is a fetal heart rate <3rd percentile for gestational age. This mild bradycardia can be easily overlooked and felt to be normal.4,5 In a fetus with mild sinus bradycardia or a signature LQTS rhythm, close attention should be paid to a history of a family member, including the mother, who had syncope without a prodrome, seizures, a history of stillbirth, or multiple fetal losses, and those with an internal cardiac defibrillator or pacemaker. Not only should history be collected for any sudden, unexplained deaths at a young age, but also accidents in the family that include single motor vehicle accidents or drowning/near drowning of an otherwise good swimmer, as these may be a sign of a syncopal event or cardiac arrest. Lastly, if one of the parents has a known LQTS variant, each offspring has a 50% chance of also carrying the variant (see later). If the family history is negative, a de novo LQT variant is possible and should be investigated further, as the arrhythmia profile tends to be more serious and the QTc interval longer than in familial LQTS.6
Fetal Diagnosis of LQTS
Fetal LQTS can be diagnosed by fetal magnetocardiography (fMCG).7,8 Confirmation of the signature rhythms, measurement of the QTc interval, and identification of repolarization abnormalities can all be shown on fMCG performed between 18 to 40 weeks of gestation (FIG. 1.3.3). While the type of LQTS can only be determined by genetic testing (see later), confirming (or refuting) suspected fetal LQTS is invaluable for pregnancy management (discussed in Part 5, Chapter 1). In brief, if LQTS is confirmed by fMCG, fetal surveillance is increased, LQT-prolonging medications are avoided, and delivery is recommended in a cardiac center.9
Inheritance
Most commonly, LQTS is inherited in an autosomal dominant fashion. Thus, there is a 50% risk for an individual to inherit a disease-causing variant from an affected parent or to transmit this variant to their offspring. The exceptions are Jervell and Lange-Nielsen syndrome and the newly recognized TRDN-related LQTS, both with autosomal recessive inheritance.10,11 Jervell and Lange-Nielsen syndrome is caused by the presence of two pathogenic variants in KCNQ1 and/or KCNE1 (FIG. 1.3.4), and even though it is a recessive disease, parents and siblings may have LQTS having inherited one of the two variants. In these cases, those with a single mutation usually have mild disease.12
Occasionally, the fetus can be the proband (the first individual identified with the pathogenic variant). Identifying the underlying genetic variant can aid in treating and counseling the parents of the proband and allows for cascade screening, a process in which at-risk relatives are tested for a known genetic variation in a stepwise fashion until all at-risk individuals are identified. Additionally, guidelines support genetic testing for those with a clinical diagnosis of LQTS.13,14
Genetic Testing
To date, at least 17 genes have been reported to be associated with LQTS. However, the majority of known disease-causing variants are identified in genes KCNQ1 (30%-35%), KCNQ2 (25%-30%) encoding for potassium channels, and SCN5A (5%-10%) encoding for a sodium channel causing LQTS types 1, 2, and 3, respectively.15 The majority of
variants identified are single nucleotide variants. Presently, 25% of people with phenotype positive LQTS remain genetically elusive, as commercial gene testing fails to uncover a known pathogenic variant.
variants identified are single nucleotide variants. Presently, 25% of people with phenotype positive LQTS remain genetically elusive, as commercial gene testing fails to uncover a known pathogenic variant.
Interestingly, the presence of a pathogenic variant alone does not predict clinical outcome because LQTS is characterized by both reduced penetrance and variable expressivity. Reduced penetrance means not every individual who has a pathogenic variant will express the LQTS phenotype. It is estimated that approximately 25% of individuals with a pathogenic variant will have a normal QTc interval on resting ECG.16 Even though these patients with “concealed” LQTS have a lower risk of sudden cardiac arrest, they do still have an increased risk over the general population.16 Additionally, almost half of individuals with pathogenic variants will not experience a syncopal episode nor report a family history of either LQTS or sudden death.17 This highlights the utility of cascade genetic testing in apparently unaffected at-risk relatives, as even though they may be phenotypically negative, they may still be genotype positive and at increased risk for cardiac arrest under certain circumstances. Furthermore, they can pass the variant to future children who may be more severely affected.
Variable expressivity refers to a difference in the clinical phenotypic or the severity of the phenotype, not only between families but also within families (FIG. 1.3.5). As an example, one individual may have a normal resting QTc and never develop a symptom over their lifetime and their sibling may experience a sudden cardiac arrest at the age of 16 years. Additionally, the same variant in SCN5A may present clinically as LQTS in one individual and as BrS in another. Variable expressivity is likely secondary to other gene-environment interactions that modify a given individual’s disease expression.
These modifiers include gender, age, and the presence of common variants in the general population that in and of themselves are not disease causing but either act as protective or increase disease susceptibility when in combination with a rare pathogenic variant.18,19
These modifiers include gender, age, and the presence of common variants in the general population that in and of themselves are not disease causing but either act as protective or increase disease susceptibility when in combination with a rare pathogenic variant.18,19
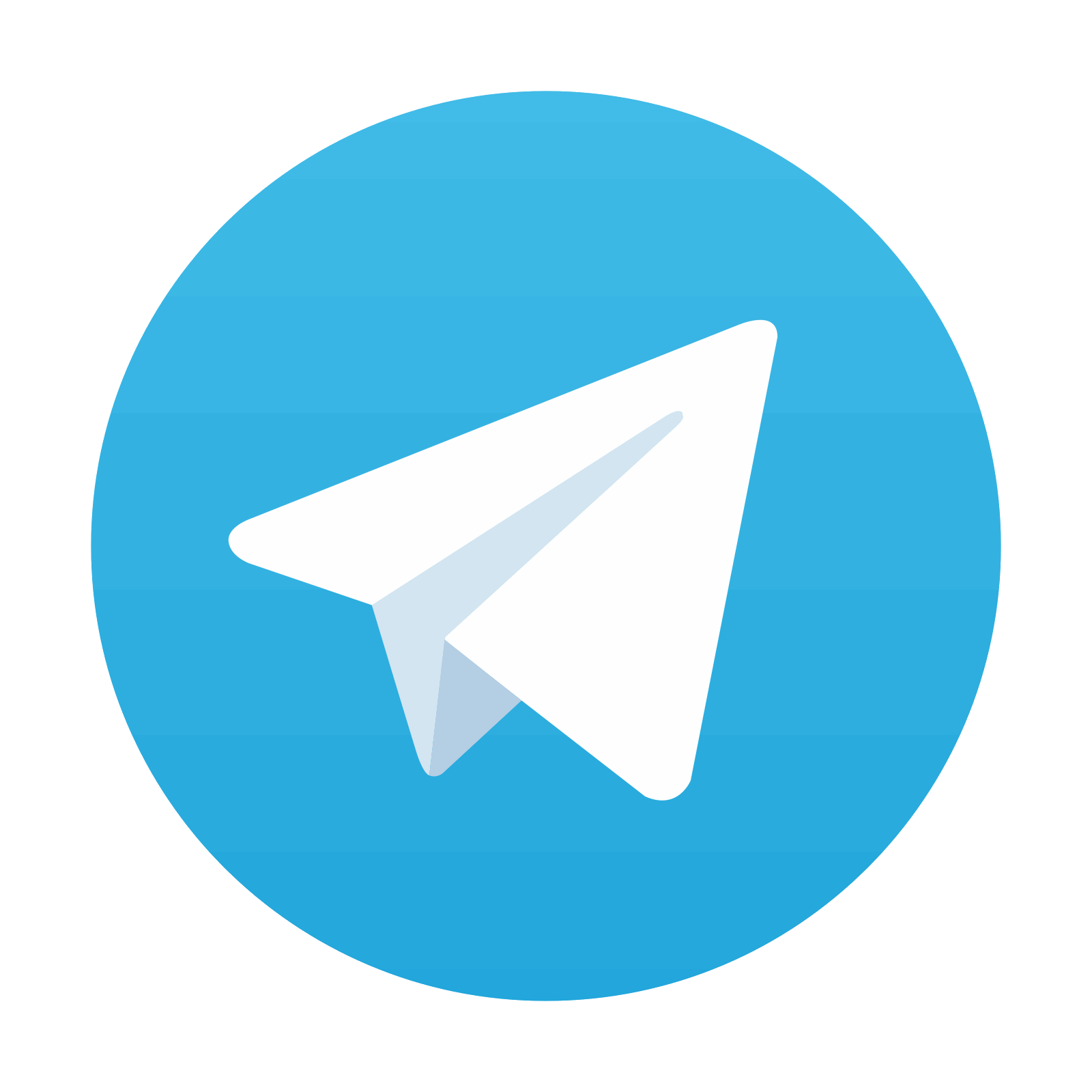
Stay updated, free articles. Join our Telegram channel

Full access? Get Clinical Tree
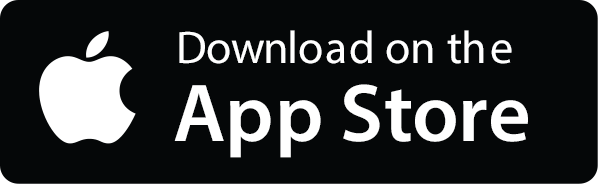
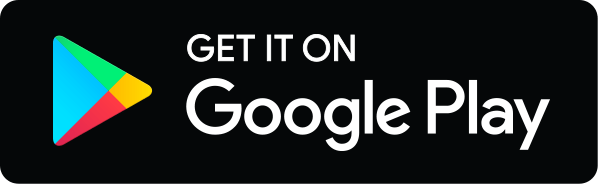
