The Elusive Fetal Electrocardiogram
Helena M. Gardiner
INTRODUCTION
The major adverse pregnancy outcomes include intrapartum mortality at 0.35 per 1000 births; stillbirth affecting 4.1 per 1000 births a year; birth asphyxia occurring in about 2% of all deliveries contributing to cerebral palsy and lifelong handicap with high litigation costs per damaged child; and prematurity affecting about 5% of all pregnancies, and these adverse outcomes have changed little over the past 20 years.1 In addition, insecurity of continuous fetal heart rate (FHR) monitoring during labor contributes to an increasing caesarean delivery rate, which is above 30% in many developed countries.
A reliable recording of fetal cardiac activity, the fetal electrocardiogram (fECG), could bring important advances into clinical practice, including noninvasive continuous monitoring of FHR; FHR variability and a reliable indication of hypoxemia during labor; long-duration monitoring “at home” during normal and pathological pregnancies (to provide greater insight into the mechanisms leading to growth restriction and stillbirth); and more accurate diagnosis of fetal arrhythmias to allow administration of the most effective drugs. These fundamental clinical issues remain challenging in the current era and continue to stimulate research to develop better technologies to solve them. As the heart’s electrical signal is produced by events occurring at cellular level, it could provide information on mechanisms controlling cardiovascular responses and their neural interaction in the developing fetus, in both normal and pathological pregnancies. The current challenge is to detect the electrical signals produced by the developing fetus consistently and over long enough periods to gain a better understanding of the maturational processes occurring in normal pregnancy. Understanding these responses is the cornerstone to more sophisticated prediction of health and disease in the developing fetus. Furthermore, knowledge of the antecedents of stillbirth and birth asphyxia would increase our understanding of the potential for programming of the neuroendocrine systems that drive later responses and in turn may have important implications for personalized medicine in future years. However, there are major challenges, not yet completely resolved, that provide barriers to fECG acquisition and its implementation as an “at-home” monitoring system and as an improvement on the Doppler cardiotocogram (CTG) in pregnancy and during labor.
CLINICAL APPLICATION OF FETAL ECG
The important clinical parameters that can be recorded from the fetal heart’s electrical signals include the basal FHR, its accelerations and decelerations, and heart rate variability. These parameters provide information about fetal well-being during pregnancy and labor. If the fECG morphology can be captured during pregnancy, its time intervals can be measured including atrial and ventricular rates; the P-, QRS-, and T-wave durations; and PR, QRS, and QT time intervals. These could provide information on physiological developmental changes, as well as fetal distress and, in cases of arrhythmias, more accurate assessment leading to improved treatment.
Current Methods of Fetal Heart Monitoring
The current methods of fetal heart monitoring include Doppler, electrocardiographic, and magnetocardiographic methods. Magnetocardiography, cooled by liquid helium, was developed during the 1990s to record and separate the small signals produced by the fetus from its environment, including the mother.2 Developments in this tool will be discussed fully in Part 2, Chapter 3, while this chapter will discuss the Doppler and fetal ECG methods of monitoring and diagnosis.
DOPPLER METHODS OF FETAL HEART MONITORING
The most commonly used clinical method of FHR monitoring during pregnancy and in labor uses the Doppler principle to produce a semicontinuous recording of fetal cardiac activity, the CTG.3,4 This method uses transducers mounted on an abdominal belt that emit a weakly focused ultrasound beam of about 1.5 MHz frequency directed toward the fetal thorax with the reflected ultrasound detected within the same transducer. The received signal is complex, including signals from the fetal heart walls and valves. This is demodulated and produces an audio signal, ranging from 200 to 1500 Hz, which the FHR algorithms process to assess periodicity (FIG. 6.1.1A). In order to avoid missing or creating heart beats, the algorithms set maximum and minimum heart rates to produce an averaged FHR. The processing is effectively similar to setting a low-pass filter.
Advantages and Limitations of Doppler Methods of Fetal Heart Monitoring
Although this is an inexpensive, portable technique and has been in use for decades, there are important practical drawbacks. The equipment uses belts that are cumbersome, and so the fetal heart trace is easily lost. Monitoring multifetal pregnancies is particularly complicated. Furthermore, the algorithms used in this Doppler technique produce an averaged FHR, and the resultant trace shows no beat-to-beat variability and has limited ability to assess accelerations and decelerations, an important parameter of fetal well-being. Additionally, the Doppler method cannot function reliably if there
is more than one strong fetal signal as it may produce a doubled FHR or semblance of fetal tachycardia. These factors contribute to the difficulty in interpretation of the CTG, and experienced personnel are required to read the tracings and make clinical decisions affecting management of the pregnancy. Adverse pregnancy outcomes are frequently attributed to suboptimal monitoring and interpretation of fetal heart traces. Computerized CTG systems attempt to improve the interpretation of traces and provide guidance but cannot alter the deficiencies of the underlying Doppler technique.
is more than one strong fetal signal as it may produce a doubled FHR or semblance of fetal tachycardia. These factors contribute to the difficulty in interpretation of the CTG, and experienced personnel are required to read the tracings and make clinical decisions affecting management of the pregnancy. Adverse pregnancy outcomes are frequently attributed to suboptimal monitoring and interpretation of fetal heart traces. Computerized CTG systems attempt to improve the interpretation of traces and provide guidance but cannot alter the deficiencies of the underlying Doppler technique.
ST Analysis Method of Fetal Heart Monitoring
A single scalp electrode inserted into the fetal scalp in labor has been shown capable of recording the fetal ECG. Components of the ECG trace are influenced by ion shifts, some of which are oxygen dependent, and alteration of the morphology of the ECG occurs in response to physiological stimuli such as hypoxemia.
There are several practical restrictions: this technique is invasive and only suitable for in-labor monitoring once the membranes have ruptured; the fetus must be in a cephalic presentation to allow access to the fetal scalp; and maternal movements are restricted because of the electrode. The development of this method is based on animal experiments that demonstrated that a reduction in oxygen led to an increase in adrenaline to induce glycogenolysis, which is accompanied by a potassium shift into the fetal myocardial cells. This was shown to alter their relaxation phase resulting in increased T-wave amplitude. ST analysis (STAN) has been assessed in combination with CTG monitoring to determine whether a change in fetal electrical signals can provide additional information to the CTG traces and improve assessment of fetal well-being. While the initial randomized controlled trial reported the combination of STAN and CTG resulted in a reduction in operative delivery and incidence of metabolic acidosis, the results could not be reproduced in other studies. There are several technical issues that contribute to the lack of reproducibility among which the one-dimensional presentation of the cardiac electrical activity and influence of fetal lie are the most important. In the 1960s, Larks described the normal fetal cardiac axis to lie between 100° and 160°, with a mean of 134° at term. The importance of fetal lie in assessing the electrical cardiac axis may be illustrated by the alteration of calculated axis in different fetal presentations. This report assumed the fetal presentation was cephalic and frontal, whereas the mean axis could be calculated to lie closer to 90° in the sagittal plane and a negative axis between 180° and 0° in a breech presentation.5
In the STAN technology, an event signaling possible fetal distress occurs if there is an alteration in the ratio of the baseline T-wave size relative to the QRS-wave (the T/QRS ratio) produced by potassium shifts at the cellular level. However, recognition and interpretation of such events are challenging: the propagation in the contraction (QRS) phase differs from that in the relaxation (T) phase, and the magnitude of alteration of the T/QRS ratio depends on the orientation of the electrical heart axis with reference to the fetal scalp electrode. If the fetal scalp lead is perpendicular to the fetal cardiac axis, the T-wave is of small amplitude producing low T/QRS values at baseline. However, if the fetal scalp lead is parallel to the fetal cardiac axis, the T-wave will be larger and produce higher T/QRS ratios at baseline. Thus for a
similar fetal response to hypoxemia, the resultant potassium shift could produce very little change in the T/QRS axis if the scalp electrode is perpendicular to the cardiac axis, yet trigger many events if they are parallel to each other.6 The disadvantage of a single-axis recording system to detect the fECG is clear from its use during in-labor monitoring, but assessment of the signal still proves challenging, even using multichannel recorders.
similar fetal response to hypoxemia, the resultant potassium shift could produce very little change in the T/QRS axis if the scalp electrode is perpendicular to the cardiac axis, yet trigger many events if they are parallel to each other.6 The disadvantage of a single-axis recording system to detect the fECG is clear from its use during in-labor monitoring, but assessment of the signal still proves challenging, even using multichannel recorders.
METHODS OF SIGNAL ACQUISITION OF FETAL ECG
Historical Review of ECG Acquisition
TABLE 6.1.1 details the chronology of acquisition of the electrocardiogram.7 The first human electrocardiogram recording from the body surface was by Augustus Desire Waller in 1887.8 He also demonstrated that the electrical signals preceded muscular contraction, and that it was not essential for the electrodes to be applied to the subject’s chest, but the ECG could be obtained from two peripheries (hands or feet) immersed in saline. Willem Einthoven developed these techniques further in 1902 using the string galvanometer to enable recognizable recordings of the P, QRS, and T components of the modern ECG waveform from a surface electrode.9
The first fetal ECG recording was made in 1906 by Cremer from various maternal sites including a combination of vaginal and abdominal electrodes, as well as from large esophageal leads.10 However, it was not until the 1950s that reports of human fetal ECG acquisition became more frequent: Smyth developed a silver wire electrode that could be attached directly to the fetus after membrane rupture in 1953,11 and a year later, Davis and Meares reported successful fECG recording using a Grass Electroencephalograph in a 19-week fetus. They used a multilead system combining abdominal and vaginal electrodes.12 In 1957, Southern published fECG time intervals recorded from maternal abdominal electrodes. He correlated P and PR time intervals with fetal oxygen saturation and bradycardic episodes and made observations on the ST segment.13
TABLE 6.1.1 SUMMARY OF ELECTROCARDIOGRAPHY MILESTONES | |||||||||||||||||||||||||||||||||
---|---|---|---|---|---|---|---|---|---|---|---|---|---|---|---|---|---|---|---|---|---|---|---|---|---|---|---|---|---|---|---|---|---|
|
However, the intense filtering required to separate the fECG from maternal signals used at that time is likely to have reduced the quality of the fECG morphology. Concurrently, intrapartum recordings were also made by placing electrodes within the uterine cavity following rupture of the membranes.14,15 During the 1960s and 1970s, attempts were made to obtain fECG from maternally sited abdominal electrodes. However, intensive high-pass filtering of the mixed maternal and fetal signal from abdominal electrodes usually led to loss of P- and T-waves from the fECG, as noted previously by Smyth. The desire to monitor the fetal heart in early labor has resulted in a pragmatic approach: use of the averaged R-R interval acquired by Doppler in FHR monitoring that persists until the present day.
Hon reported on the use of the fetal scalp electrode in 1962 and worked on improving the signal-to-noise ratio, describing peaked biphasic P-waves and shortened PR intervals in cases of human fetal hypoxemia.16 However, small sample size, the use of anencephalic fetuses, and inadequate computing power and filtering methods resulted in major difficulties with signal separation and a concern that responses of anencephalic fetuses to hypoxemia may not be the same as normal fetuses. The chances of detecting P- and T-waves within the fECG were first improved by using a bandwidth of 0.1 to 100 Hz to filter the abdominal signals.17 However, research still failed to report the anticipated fECG changes (lengthening of PR or QR intervals and ST segment depression or T-wave inversion) in hypoxic subjects.18,19 Continuing technical challenges into the 1970s resulted in conflicting data from animal studies, particularly in interpretation of the ST segment: some data finding the response to hypoxemia were an elevation of the ST segment and augmented T-waves20,21; the latter study corroborated the fECG findings with postmortem studies showing reduced neonatal myocardial glycogen and creatinine phosphate. Other researchers reported the response to hypoxemia manifests as ST segment depression and reversal of the T-waves and also demonstrating an elevated T/QRS ratio on postnatal ECG.22,23 Only one human study during this era correlated fetal scalp electrode recordings with scalp blood and cord acid-base status after delivery, reporting lengthening of the corrected QT interval and T-wave inversion with fetal acidosis.24
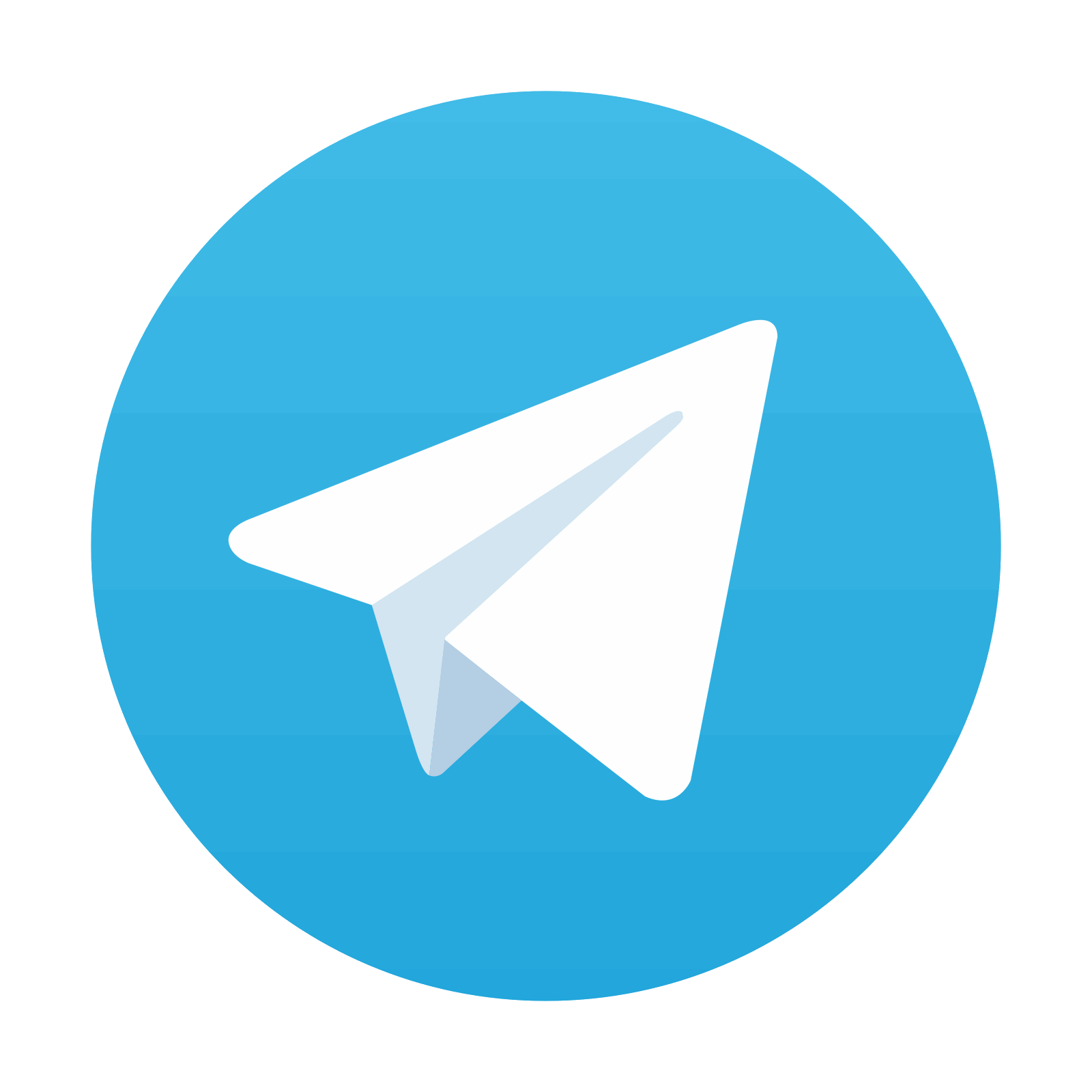
Stay updated, free articles. Join our Telegram channel

Full access? Get Clinical Tree
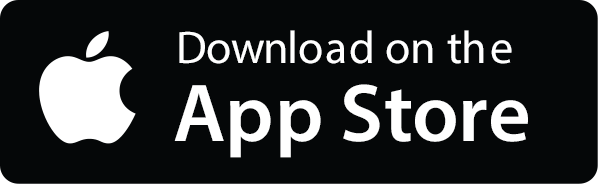
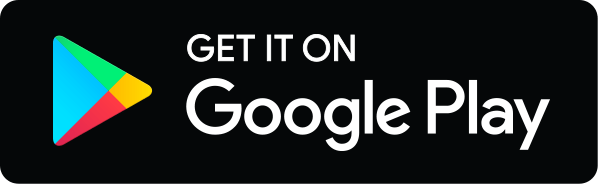