Abstract
Our understanding of the role of disease-causing variants in CFTR has culminated in two new variant-specific CFTR modulator therapies approved by the US FDA. These modulators target defects in CFTR biogenesis and in channel gating. Clinical trials are directed to improving the efficacy of these first-generation molecules. However, other CFTR variants that lead to nonsense-mediated decay, aberrant splicing, and reduced gene expression are refractory to CFTR modulators and will require new therapeutic approaches. To this end, new CF animal models are yielding key insights into tissue-specific disease mechanisms, such as impaired mucociliary clearance and pancreatic dysfunction. Although infection and inflammation remain challenging to treat, progress in targeting CFTR directly will result in individuals with CF living longer. To maximize life span, earlier interventions, soon after diagnosis, are needed. Finally, the advances in precision medicine for CF may benefit more common disorders such as COPD and pancreatitis where CFTR dysfunction contributes to pathophysiology.
Keywords
CFTR, DNA variant, splicing, chloride, sodium, nonsense-mediated decay, protein folding
The genetic basis of cystic fibrosis (CF) has been recognized by the medical community since the 1940s. A genetic etiology and autosomal-recessive inheritance was suggested by the recurrence of CF in siblings and the absence of the illness in parents. Genetic linkage analysis confirmed that a single locus was responsible for classic CF. In 1989, technical breakthroughs allowing identification of disease-causing genes on the basis of position rather than function enabled Tsui and colleagues to clone the gene responsible for this disorder. The identified gene was aptly named the cystic fibrosis transmembrane conductance regulator (CFTR). During the past three decades, our understanding of the molecular basis of CF has exploded. We now recognize that deleterious variants in CFTR give rise to a range of phenotypes, extending from classic CF to single-organ pathology. The successful use of therapies directed at the complications of CF has raised the question as to the potential efficacy in disease phenotypes that mimic CF, including idiopathic bronchiectasis, chronic Pseudomonas aeruginosa airways colonization in tracheostomy patients, or chronic obstructive pulmonary disease. The assumption that all carriers of a single disease-causing variant in CFTR are asymptomatic also has been questioned. The consequences of many variants on the function of the encoded protein have been determined, allowing correlation of altered function of CFTR with CF pathophysiology. Two variant-specific CFTR modulator therapies are approved by the Food and Drug Administration (FDA) in the United States. Additional investigational molecules capable of repairing several different classes of CFTR variants are in clinical trials in North America, Europe, and Israel, potentially increasing the number of patients eligible for drugs directed at the primary cause of CF.
CFTR Gene
Structure
The CFTR gene resides on the long arm of human chromosome 7 and encompasses approximately 189,000 base pairs of DNA. The coding portion of the gene is divided into 27 exons that are transcribed into a messenger RNA (mRNA) of approximately 6500 base pairs. The exon and intron structure of the CFTR gene is well conserved among mammals and evolutionarily distant species such as amphibians (Xenopus) and fish (killifish and dogfish).
Transcription of CFTR is initiated 80 base pairs upstream from the start site of translation. The CFTR gene demonstrates exquisite temporal and spatial differences in expression. For example, specific cell types within the human lung express high levels of CFTR, while surface epithelial cells have a very low level of CFTR expression. CFTR expression is also regulated during organ development. Despite considerable evidence that the expression of CFTR is highly selective, the precise elements that regulate CFTR expression have not been identified. Sequences immediately upstream of the transcriptional start site of CFTR generate low-level expression in most tissues. This pattern of expression is consistent with the presence of basal promoter elements. A cyclic adenosine monophosphate (cAMP) response element has been identified within this region of CFTR, but this element does not appear to account for the spatial and temporal expression patterns. Assays that search for binding of protein to DNA have identified several regions elsewhere in the CFTR gene that may be involved in transcriptional regulation. Strain-specific differences in CFTR expression in mice have been mapped to sequences about 700 base pairs upstream of the CFTR gene, indicating the existence of regulatory elements in this region. Epigenetic mechanisms such as methylation do not appear to play a major role in regulating CFTR expression. However, there is growing evidence that microRNAs (miRNA) modulate the level of CFTR mRNA transcripts. Thus, despite extensive efforts to locate transcriptional elements, the molecular mechanisms regulating CFTR expression are not completely understood.
Splicing
Variations in CFTR splicing patterns have been identified by the reverse transcription-polymerase chain reaction technique. There are relatively few alternatively spliced transcripts that create functional isoforms of CFTR. RNA transcripts resulting from alternative splicing of exon 5 CFTR create a functional form of CFTR in the rabbit heart but have not been identified in other species or organs. Transcripts missing a portion of exons 13 and 14a encode a functional isoform of CFTR expressed in mouse and human kidney. Neither the heart nor the kidney appears to be primarily involved in CF pathophysiology; thus the role of these alternatively spliced forms of CFTR is unclear.
In contrast to alternative splicing, many aberrantly spliced versions of CFTR have been identified. Aberrantly spliced CFTR RNA transcripts do not produce functional CFTR. Aberrant splicing occurs as a result of variation in intronic or exonic sequences required for normal splicing of CFTR. The aberrant splicing of exon 10 (legacy exon 9) is a notable example. Variation in the length of the polythymidine tract, an element in the 3′ splice site of intron 9, is correlated with the amount of properly spliced CFTR mRNA. Longer versions of this tract called 9T and 7T are common in the population and are associated with high levels of normally spliced CFTR mRNA. A shorter version of this tract called 5T is associated with a substantial reduction in full-length CFTR RNA. While abbreviated polythymidine tracts in 3′ splice sites do not usually cause mis-splicing of the subsequent exon, it appears that a special situation occurs in the CFTR gene. Sequence surrounding exon 9, including unusual variation in splice sites surrounding exon 9 and splice-silencing sequences in intron 9, appear to make exon 9 vulnerable to mis-splicing. Thus alterations in secondary splicing signals of this exon such as the polythymidine tract have an exaggerated effect on splicing. The splicing efficiency of CFTR genes bearing 5T also varies by tissue. The vas deferens splices CFTR genes bearing the 5T variant less efficiently than respiratory epithelia, which explains the existence of congenital bilateral absence of the vas deferens (CBAVD) without other symptoms.
Individuals carrying the 5T variant in their other CFTR gene manifest a variety of phenotypes, ranging from healthy to nonclassic CF (discussed later). The latter phenomenon is due to the variable effect that 5T has on the splicing of CFTR exon 9. This splicing variability is caused by variation in the number of TG dinucleotides adjacent to the polythymidine tract. The role of the TG tract in splicing of 5T alleles is controversial. It may serve as a site for binding of a splicing repressor, or the RNA may form a secondary structure such as a hairpin that affects gene expression. Either way, there is a robust correlation between the length of the TG tract and the phenotypes associated with a 5T variant.
Many other CFTR transcripts derived from aberrant splicing of exons 9 through 12 have been reported, yet none appear to be correlated with phenotype. Another example of aberrant CFTR splicing involves exons 23 and 24, resulting in a prematurely truncated CFTR protein that is nonfunctional. The many variants that affect CFTR splicing and result in disease are summarized in the following section.
DNA Variants
More than 2000 variants in the CFTR gene associated with disease have been reported to the Cystic Fibrosis Genetic Analysis Consortium ( http://www.genet.sickkids.on.ca/cftr/ ). The Consortium website has implemented variant nomenclature according to Human Genome and Variome Society (HGVS) guidelines. Variants are now provided in legacy and HGVS format ( http://www.genet.sickkids.on.ca/cftr/ ). Legacy names are used in this chapter, and the HGVS nomenclature is provided with the first mention of a variant. The vast majority of CFTR variants reported to be associated with disease involve only one or a few nucleotides. Nearly 50% of variants change an amino acid, while variants that affect splicing or introduce a nonsense codon account for approximately 25%. Deletion and insertion variants that alter the reading frame and usually result in the introduction of a premature termination codon account for about 20%. Deletions and insertions involving multiples of three nucleotides are much less common (2%). The latter variants do not shift the reading frame, so a protein missing one or more amino acids is usually produced (e.g., CFTR with the F508del [HGVS p.Phe508del] variant). Most of the remaining disease-associated variants (3%) involve the deletion of larger regions of DNA involving hundreds to thousands of base pairs. Finally, a few variants (1%) have been purported to occur in sequences thought to be involved in transcriptional regulation of the CFTR gene, although a convincing case has been made in only a few instances. In addition to the disease-associated variants, almost 270 variants in CFTR have not been associated with disease. There are some variants in CFTR (e.g., M470V HGVS p.Met470Val) that may affect phenotype. Variants that are “silent” (that do not change an encoded amino acid or known splicing signal) are not predicted to alter CFTR function. However, the elucidation of intronic and exonic signals that enhance or repress exon splicing raises the possibility that some “silent” changes may affect CFTR splicing.
The distribution of disease-associated variants in the CFTR gene among human populations has been extensively reviewed. One variant, a deletion of three nucleotides that leads to loss of a single phenylalanine residue at codon 508 (F508del), accounts for approximately 70% of CF alleles in Northern European Caucasians. The frequency of the F508del variant varies on the European continent from less than 50% of CF alleles in Southern Europe to as high as 88% of CF alleles in Denmark. The F508del variant is quite rare in native Africans and native Asians. However, the F508del variant is found in racially admixed populations. For example, 30% of the CFTR genes in American CF patients of African descent have the F508del variant. On the other hand, the second most common variant in African Americans with CF (3120 + 1G>A; HGVS c.2988 + 1G>A) appears to be a common CF allele in native Africans. Deleterious CF alleles also have been found in CF patients of Asian ancestry. These variants have not been observed in Caucasians or native African populations. Thus rare deleterious variants in the CFTR gene occur in all human populations. However, it is the frequency of the F508del variant in Europeans that accounts for the high incidence of CF in Caucasians.
In addition to F508del, only about 25 other variants occur, with a frequency of 0.1% or greater in the Caucasian CF population. Other variants are seen with some frequency in African Americans. Finally, some ethnic groups have one or more variants that reach a frequency of ≥1% that are rare in the general population. The higher frequency of the latter variants is probably the consequence of a founder effect. The remainder of the reported variants (~1000) has been reported in only a small number of individuals. Screening for the F508del variant and 22 variants that have a frequency of greater than 0.1% detects about 85% of variants in Caucasian CF patients. Variant screens involving 50–100 CF variants produce a minimal increase in detection rate in the general CF population (1%–3%). Because there are so many rare variants, in order to achieve a detection rate for classic CF over 95%, screening of the entire coding region of the CFTR gene is required.
CFTR Protein
Characteristics
The CFTR protein is composed of a linear stretch of 1480 amino acids that form an integral membrane glycoprotein. A comparison of the nucleotide sequence across available databases places this protein as a member of the ABC transporter superfamily. The topology of the protein contains the common features of twofold symmetry with six transmembrane domains and an intracellular nucleotide-binding fold. The CFTR is unique in the presence of a central intracellular R domain enriched in phosphorylation sites used by the protein kinases A and C. Although most members of the superfamily are transporters (e.g., the multidrug resistance transporter (MDR) transports chemotherapy agents), the CFTR is a cAMP-regulated chloride and bicarbonate channel that must function for the outwardly rectifying chloride channel (ORCC) to transport chloride. Functional CFTR also downregulates the epithelial sodium channel (ENaC) in those cells in which both reside. More controversial functions have been reported, including transport of nucleotides and fatty acids.
Biogenesis
The CFTR polypeptide is translated from the mRNA in the endoplasmic reticulum (ER) as a linear chain of amino acids with hydrophilic (water-soluble) and hydrophobic (lipid-soluble) domains. The nascent polypeptide is assisted by interactions with chaperone proteins within and outside the ER, to assume a tertiary or folded structure that buries the membrane-spanning domains in the ER membrane. The process is relatively slow, taking approximately 10–15 minutes to complete, and is followed by two different fates. Depending on the cell type making the CFTR, the wild-type (WT) chain could be ubiquitinated and sent to the proteasome for degradation, or it could continue along the trafficking pathway to the Golgi apparatus. Chloride transport through the CFTR can be detected in the ER using patch-clamping techniques, but it is not known whether the immature functional channel is the one protected from proteasomal degradation. The successful CFTR is packaged into vesicles for transport. Adequate anion channel transport through cell surface CFTR depends not only on the characteristics of the channel pore or degree of phosphorylation and ATP hydrolysis but also on numbers of CFTR proteins and residence time at the surface. CFTR is actively recycled from the plasma membrane, and some mutants are much less stable than WT- CFTR.
Function
The three-dimensional topology of CFTR is under investigation. The two nucleotide binding domains (NBDs) and the R domain probably interact such that phosphorylation of the R domain is required for chloride channel opening and probably affects the affinity of the NBDs for adenosine triphosphate (ATP). ATP hydrolysis at NBD1 is associated with opening the channel, but hydrolysis at NBD2 is associated with closure. The chloride pore is formed by transmembrane domains, and the single-channel conductance is low, at 6–10 picosiemens. The current-voltage relationship is linear, meaning that it is just as easy for chloride to leave as to enter the cell, depending on the direction of the voltage applied across the membrane. This bidirectionality is possible because ATP is the ligand that is hydrolyzed to gate the channel.
The most common variant in CFTR, the F508 deletion of the code for phenylalanine at position 508, has two consequences. First, the channel has a low open time for chloride—about 10% of normal. Second, the newly translated CFTR is unstable in the ER, leading to premature proteolysis and a reduced half-life in the cell. The variant appears to affect intermolecular interactions within CFTR rather than NBD1 folding.
Cellular Distribution and Function
Developmental Expression and Function
CFTR mRNA is detectable in many fetal tissues in the first trimester. Data have been collected for fetal lung, intestine, pancreas, and kidney from humans, rats, rabbits, and mice. During early mammalian embryonic tissue development, CFTR may be more important as a regulator of other ion channels rather than as a pathway for chloride transport. CFTR protein becomes detectable in the second trimester, still quite early in development. CFTR expression declines in postnatal lung, and this decline begins even earlier in the rat, during the last trimester. There is a bronchial centrifugal expression gradient and a developmental shift from nonpolar localization to apical localization, with a timing that matches that of CFTR regulation of the ENaC. In other words, since ENaC function is required to resorb fetal lung fluid at birth as an adaptation to air-breathing, CFTR expression, a downregulator of ENaC function, declines and ENaC expression increases.
Postnatal Expression and Function
Regulated airway fluid and ion composition become more important for the air-breathing mammal than they were during lung development. CF lungs are morphologically normal at birth, in part because there are sufficient alternative chloride transport pathways in fetal lung to support tissue expansion and differentiation. After birth, airway mucociliary clearance is affected by the depth and characteristics of the periciliary fluid layer, which in turn is dependent on ENaC function. Over time, the human infant with pancreatic-insufficient CF is increasingly vulnerable to infection and inflammation in the airways, which lead to the classic lung phenotype of airways obstruction.
Tissue Distribution
Absent or reduced function of CFTR can be measured quantitatively in the sweat duct, where the reabsorptive coil is designed to conserve chloride, sodium, and water to protect the mammal from dehydration. The diagnostic sweat test takes advantage of the cholinergic pathway for generation of sweat by the sweat gland coil. CFTR is only responsible for at most 1% of sweat generation but is required for chloride reabsorption in the duct, thus leading to elevated sweat chloride concentrations.
CFTR plays a vital role in several other organs, tissues where epithelial proteins are secreted along with electrolytes. The most important organs are the airways, bile ducts, pancreatic ducts, and vas deferens. Interestingly, although CFTR is highly expressed in the kidney, its absence is not detrimental. The simplified scheme in which CFTR plays an electrophysiologic role to control the ion and water content of luminal secretions is insufficient to explain the nature of CF lung disease. In CF lungs, additional abnormalities of fluid are associated with chronic inflammation and infection.
Genotype-Phenotype Correlations
Molecular Consequences of Variants
The implementation of newborn carrier screening for CF and the development of CFTR targeted therapies has brought renewed interest in the clinical and functional consequences of variants in CFTR. To increase the sensitivity and specificity of genetic testing for CF, a project entitled the Clinical and Functional Translation of CFTR or CFTR 2 was undertaken. In its first phase, CFTR 2 coordinated the collection of clinical data on almost 40,000 individuals with CF from 25 countries. These individuals carried 1,036 unique CFTR variants. Comprehensive clinical and functional analysis of 159 variants that achieved a frequency of 0.0001 (0.01%) revealed that only 127 (80%) could be definitively assigned as CF-causing. Of the remaining 32 variants, 12 (7.5%) lacked clinical and functional evidence to be CF-causing, while the remaining 20 could not be assigned. The latter group consisted of variants that had varying clinical consequence (VCC) and a few variants of uncertain significance (VUS) that lack sufficient clinical or functional evidence to be assigned. The CFTR 2 project has undertaken a second round of data collection, thereby increasing enrollment to almost 90,000 individuals with CF from 40 countries, of which 69,745 were alive in 2015. The additional clinical data, along with more extensive functional analysis of variants that affect CFTR splicing, have allowed for the assignment of disease status to 306 variants (272 CF-causing, 19 VCC, non-CF causing, and 3 VUS as of August 2016; http://www.cftr2.org ).
The functional consequences of CF-causing variants have historically been grouped into five (or six) classes that are described as follows. Variants that have consequences that do not fall into one of the five classes are discussed at the end of this section. This scheme has proven useful for relatively coarse correlation with disease severity in the lung, pancreas, and sweat gland. However, a number of variants, including F508del, display effects on CFTR that fall into two or more of these classes. Recognizing that variants can have multiple effects is important for optimizing CFTR -targeted treatments.
Class I variants cause changes in the synthesis of CFTR by affecting CFTR transcription. These variants manifest their effect within the nucleus and usually involve the processing of transcripts. In most cases, the introduction of a premature termination codon, whether by a change of a codon to a nonsense variant or by frameshift, results in degradation of the transcript by a nonsense-mediated mRNA decay mechanism. G542X (HGVS p.Gly542X) is an example of this type of variant. In some cases, the premature termination codon does not lead to nonsense-mediated decay, and a truncated but nonfunctional protein is produced (e.g., R1162X [HGVS p.Arg1162X]). A third consequence of nonsense variants is that they can cause skipping of the exon in which they occur. An example of this phenomenon is seen with the R553X (HGVS p.Arg553X) variant. Variants in the highly conserved splicing signals 5′ and 3′ of exons invariably lead to a loss of full-length transcript due to exon skipping. Loss of exons can also cause a shift in the reading frame, leading to the introduction of a premature termination codon and subsequent RNA degradation. In each case, these types of variants lead to complete, or almost complete, loss of functional CFTR protein and produce a classic form of CF ( Fig. 49.1 ). Some of the nonsense variants in CFTR have been shown to be rescued in cell lines with application of aminoglycoside antibiotics that can induce read-through of premature termination codons. Atalauren (PTC124), an investigational drug reported to suppress termination codons in some studies but not others, is in phase III clinical trials in CF worldwide. The premature termination codon mutants are relatively frequent in Israel, where early phase clinical trials with Ataluren have shown some promise, whereas a double-blind placebo controlled phase III study did not show improvement in lung function.

The second class of variants involves those that affect the processing of CFTR (see Fig. 49.1 ). A prime example of a class II variant is F508del. Loss of the phenylalanine residue at codon 508 within the first nucleotide-binding fold leads to a misfolding of CFTR. Misfolded CFTR is recognized by chaperones that shunt the misfolded protein to degradation pathways. The folding defect can be partially overcome by reduction in temperature. Cells grown at 21°C instead of 37°C can produce properly folded CFTR. Folded CFTR bearing F508del is functional in the cell membrane, but it is not stable and is more rapidly removed from the membrane than its WT counterpart. Because F508del is found in at least 70% of CF patients, the mechanisms underlying CFTR degradation have been studied intensively, in hopes of finding approaches that permit CFTR bearing the F508del variant to evade degradation (see Chapter 53 ). Other class II variants have been found that reduce folding efficiency, resulting in a small amount of properly folded functional CFTR at the cell membrane. Two new molecules have been developed for correction (lumacaftor) and potentiation (ivacaftor) of the F508del mutant protein. Phase III clinical trials demonstrated that the two drugs in combination produce improvement in lung function, decrease in pulmonary exacerbations, and increase in body mass index in CF patients homozygous for the F508del variant.
Disease-associated variants in class III affect the regulation of CFTR (see Fig. 49.1 ). Binding of ATP to the NBDs is required to activate CFTR. Variants that affect CFTR interaction with ATP have been shown to alter its function. An example is the G551D (HGVS p.Gly551Asp) variant, in which the glycine (G) at codon 551 is substituted with aspartic acid (D). The G551D variant severely affects interaction with ATP, creating a form of CFTR that is properly folded and inserted in the cell membrane, yet unable to be activated. Multiple clinical trials of individuals carrying at least one CFTR gene with the G551D variant show that the novel drug ivacaftor (Vertex compound VX770) is safe and produces substantial improvements in lung function, reduction in sweat chloride concentration and infection with P. aeruginosa, and increase in body mass index. Since G551D affects the gating behavior of CFTR, variants that have similar function effects on CFTR have been tested for response to VX770. A group of “gating” variants that respond to VX770 in cell-based studies showed significant clinical response in vivo. These results suggest that the hundreds of CFTR variants without current approved treatment might be grouped according to functional effect and cellular response to drugs, so-called theratypes. Such an approach could expedite the use of approved drugs for the many CFTR genotypes carried by CF patients.
Class IV variants alter the chloride conduction properties of CFTR, and in some cases affect the magnitude and the ion selectivity of the channel pore. Most class IV variants occur in the transmembrane domains. Since class IV variants may reduce but do not eliminate the flow of ions, some CFTR function is preserved ( Fig. 49.2 ), which in turn leads to a milder phenotype or a nonclassic form of CF. However, by the same token, it is recognized that altering this property of CFTR produces CF. Thus loss or alteration of the chloride channel function of CFTR is key to the development of the CF phenotype, demonstrating that a defect in chloride transport is the primary abnormality in cells of CF patients.

Class V variants are also associated with a milder form of CF, because they can also result in the production of a reduced amount of normally functional protein (see Fig. 49.2 ). An example of the latter type of variant is 3849 + 10 kbC > T (HGVS c.3717 + 12191C > T). This variant occurs within intron 22 (legacy intron 19), approximately 10 kilobases from the nearest splice site of exon 22, and leads to the aberrant splicing of CFTR, a nonfunctional protein. However, the aberrant splicing mechanism is incomplete, and a small amount of normally spliced CFTR transcript is made, leading to the synthesis of some normal CFTR and a CF phenotype that is moderate in severity.
CF-causing variants have been shown to affect other functions of CFTR. CFTR regulates other ion channels, such as the ENaC and the ORCC. Variants in the first NBD have been shown to variably affect CFTR regulation of these channels. Variants in the N terminus or in the C terminus of CFTR can affect the trafficking, membrane insertion, or stability of CFTR. These genotypes are sometimes classified as class VIA or VIB. Finally, variants in ENaC have been shown to cause cases of atypical CF.
Clinical Consequences of Variants
Correlation of the CFTR genotype with the CF phenotype has been investigated using three methods. In genotype-driven studies, patients are grouped according to genotype, and clinical features are compared. When patients who were homozygous for the F508del variant were compared with patients who carry one copy of F508del and a different CF variant, it was shown that a number of the less common variants produce the same degree of disease severity as the F508del variant. Some variants cause less severe organ disease, or disease in a subset of organ systems affected in classic CF, resulting in a “nonclassic” CF phenotype. The CFTR genotype demonstrates a consistent association with the severity of pancreatic disease. A less consistent correlation has been noted with abnormalities in sweat chloride concentration. However, only one variant, A455E (HGVS p.Ala455Glu), has been unequivocally correlated with severity of lung disease. A few other variants, particularly those in class IV and V, may associate with less severe lung disease. In the phenotype-driven method, CFTR variants are identified in patients grouped according to disease severity. This approach has identified relatively uncommon variants that are associated with nonclassic forms of disease. A third approach has been to correlate the class of variant with the severity of disease. Those who carry variants that cause a complete or nearly complete loss of function, such as class I, II, and III variants, usually manifest classic CF. Variants that permit some residual function, such as those in classes IV and V, have been associated with milder phenotypes usually associated with pancreatic sufficiency. However, it is important to recognize that many exceptions exist. For example, CFTR containing A455E affects the processing of CFTR (class II) but is associated with pancreatic-sufficient CF and milder lung disease.
Diagnosis of atypical forms of CF can be difficult, especially when one or more alleles fails to demonstrate a disease causing variant. Sweat chloride may be normal in some combinations of CF genotypes, making the test less reliable for nonclassic CF. Standardization of protocols to measure nasal epithelial chloride and sodium transport has proven useful in the diagnosis of CF. The nasal potential difference (NPD) test can detect both classic and nonclassic forms of the disease, and has been a secondary outcome measure in clinical trials of ataluren, VX809, and VX770.
Pathophysiology
CF is caused by the reduction or dysfunction of the CFTR, a cAMP-regulated chloride and bicarbonate channel and a master regulator of other ion channels that coexist in the apical membranes of cells lining the airways, sweat glands, hepatobiliary system, and reproductive tracts. In the absence of disease, CFTR activity downregulates or tempers the sodium absorption through ENaC. In CF, the combination of a reduction in chloride transport and unregulated excessive sodium transport leads to the classic ion and water hydration defects that occur in the pancreas and vas deferens in utero, and the airways, biliary tract, intestines, reproductive tracts, and sweat glands after birth. CFTR is also expressed in other organs that do not succumb to disease, such as the heart and kidneys. Abundant alternative channels exist in those tissues to compensate for lack of CFTR -mediated chloride transport.
Airways Dehydration and Disruption of Mucociliary Clearance
Airway surface liquid (ASL) bathes the cilia of the upper airway, and the depth and composition of this fluid is regulated by CFTR and ENaC. In CF, the ASL height is reduced and cilia are less efficient at moving particles and mucus. Secondary effects of CFTR dysfunction include abnormal mucus secreted by the submucosal glands and epithelial cells. The blanket of airway surface mucus becomes excessive, but does not help eradicate bacteria that colonize and inflame the CF airways.
CFTR controls both the ENaC and the ORCC. When CFTR is absent, the ORCC cannot secrete chloride, amplifying the secretion defect. The calcium-activated chloride channel (CaCC) and the pH-activated ClC-2 coexist and are independent of CFTR. The latter are therapeutic targets in CF. A phase III program testing aerosolized denufosol, which activates the CaCC through binding to P2Y2 receptors and increasing intracellular calcium, failed to improve lung function over 1 year. It is yet unclear whether activation of an alternative chloride secretion pathway will be sufficient to overcome the defects associated with classic CF.
CF airways quickly become colonized with bacteria after birth. Defective mucociliary clearance likely plays a major role, but additional defects in bacterial clearance related to the importance of CFTR in the lipid raft region of the plasma membrane are emerging. Inflammation in response to bacterial colonization is excessive and ineffective. The ASL becomes infiltrated with neutrophils that respond to IL-8 secretion. As the neutrophils die, they liberate their DNA, which contributes to the viscosity of the mucus. Aerosolized DNase thins this mucus and improves lung function. Aerosolized hypertonic saline also facilitates clearance and improves lung function by a different mechanism.
Cystic Fibrosis Animal Models
CF research is perhaps the only field that benefits from having five mammalian genetic animal models, including the mouse, rat, rabbit, ferret, and pig. While the phenotype of the CF rabbit model has not been published, scientists have learned much from the differences and similarities between species. Broadly speaking, phenotypes can be broken down into lung, gastrointestinal (intestine, pancreas, liver, and gallbladder), and reproductive (vas deferens).
Lung Phenotypes
With more than two decades of research on CF mice of various CFTR genotypes, the general consensus is that they have a negligible or mild lung phenotype Early research implicated a CaCC in the airways of mice as compensating for the lack of CFTR. More recent research has suggested that CFTR counterbalances H+ secretion through the H+/K+ exchanger ATP12A, which acidifies ASL and impairs innate immunity in CF humans, but not mice, due to the differential expression of this exchanger. Despite the lack of a robust lung phenotype in CF mice, there is evidence that they have a hyperinflammatory phenotype when challenged with pseudomonas-laden agarose beads. While the CF rat model is relatively new, it does not develop a spontaneous bacterial colonization phenotype in the lung, despite having a reduced ASL volume and a reduction in submucosal gland mass.
The lung phenotype in CF ferrets and pigs is considerably more robust than in CF rodents and clearly demonstrates similarities to the CF human phenotype. When challenged with bacteria, both newborn CF pigs and ferrets have impaired antibacterial killing in comparison to non-CF animals. Interestingly, impaired innate immunity in newborn CF ferrets appears to be selective for P. aeruginosa, despite the fact that other species of bacteria colonize the lung later in life. Studies in CF pigs have suggested that the lack of CFTR -dependent bicarbonate secretions leads to a reduced ASL pH, and that this impairs bacterial killing at the airway surface. By contrast, impaired antibacterial killing of ASL from CF ferrets is unaltered by bicarbonate. Unlike CF pigs, the lungs of newborn CF ferrets are rapidly colonized by bacteria after birth and die within the first week of life if not reared on antibiotics. These differences in early innate immunity between CF pigs and ferrets may be due to the fact that ferrets postnatally develop submucosal glands and ciliated cells, whereas pig airways are fully developed at birth. By a week of life, mucociliary clearance rates decline in CF as compared with non-CF ferret airways as ciliogenesis increases. There remains a debate as to whether the ASL is dehydrated in these CF models with supporting evidence in the ferret and pig models using microoptical coherence tomography and other refuting evidence in the pig model using histologic endpoints.
Inflammation in the CF lung is thought to be an important component of the disease process, with evidence in CF mice for a hyperinflammatory phenotype. While inflammation is not observed in the newborn CF pig lung, newborn CF ferret lungs have elevated IL-8 and TNFα and reduced IL-1β in the bronchoalveolar lavage fluid (BALF), despite having no differences in bacterial load as compared with non-CF animals. While sterile BALF harvested from breathing C-sectioned ferrets lacked genotype-specific differences in these cytokines, IL-8 induction in the BALF of naturally born as compared with C-section animals was only observed in CF animals, suggesting that first bacterial exposure to the lung induces IL-8 in the absence of CFTR. Interestingly, proteomics analysis of CF and non-CF newborn and C-section BALF suggests that certain pathways that control immunity and inflammatory (including the complement system, macrophage functions, mammalian target of rapamycin signaling, and eukaryotic initiation factor 2 signaling) are repressed in the naive CF ferret lung, and that these pathways are accentuated at birth following exposure to bacteria Similarly, gene expression array studies on 15-day-old CF ferret lungs demonstrate significant elevations in IL-8-mediated inflammatory pathways. More recent transcriptome analysis of newborn CF pig lungs challenged with heat-killed bacteria also suggests dysregulation of acute inflammatory responses, as compared with non-CF littermates.
Gastrointestinal Phenotypes
The intestine, pancreas, liver, and gallbladder are all adversely affected in CF, although the penetrance of clinically apparent disease varies for each of these organs. All CF animal models have intestinal disease that manifests either in utero (ferret and pigs) or at weaning (mice and rats). Meconium ileus, an in utero complication of gut obstruction, is observed in ~15%–20% of CF patients. The incidence of meconium ileus is higher in CF ferrets (75%) and pigs (100%), while CF mice and rats generally manifest gut obstruction at weaning. Genetic modifiers of meconium ileus have been identified in CF humans, and this also appears to occur for meconium ileus in CF ferrets and gut obstruction at weaning in CF mice. Juvenile CF ferrets also present with other intestinal CF phenotypes, including rectal prolapse and distal intestinal obstruction syndrome (DIOS).
Exocrine pancreatic disease has a significant impact on the health of CF patients, with ~85%–90% being pancreatic-insufficient within the first 2 years of life. CF animal models have a diverse range of exocrine pancreas phenotypes. CF rodents (mice and rats) generally lack apparent disease in the exocrine pancreas, and in mice this is thought to be due to an alternative CaCC that compensates for the lack of CFTR. Disease of the exocrine pancreas begins in utero in CF pigs, with significant acinar cell atrophy and pancreatic remodeling by birth. In contrast, CF ferrets are born with minor abnormalities of pancreatic histopathology but have progressive loss of acinar cells within the first month of life. While nearly all CF ferrets are born pancreatic-insufficient, with impaired growth and a lack of fecal elastase in the stool, a small percentage of CF ferrets have been found to be pancreatic-sufficient with normal growth and fecal elastase present in the stool. Such findings suggest that genetic modifiers of pancreatic disease also exist in this species. However, it has not been possible to capture this pancreatic-sufficient phenotype through breeding to date, suggesting that multiple genetic modifiers may be responsible. The progression of exocrine pancreatic disease in CF ferrets occurs within the first several months of life and is associated with a robust inflammatory and fibrotic response that transitions to adipogenic remodeling by 3 months of life.
Liver abnormalities are not uncommon in CF patients and are characterized by biliary cirrhosis (25%), hepatic steatosis (30%), and clinical cirrhosis (5%). These disease processes are considerably more variable in CF animal models. Both CF mice and rats lack major pathologies in the liver, and CF ferrets also lack signs of liver disease at birth. However, with age, ferrets develop variable signs of steatosis, necrosis, biliary hyperplasia, and biliary fibrosis in ~39% of animals. Newborn CF pigs demonstrate evidence of focal biliary cirrhosis and a reduced pH of bile collected from the biliary ducts.
Gallbladder disease occurs in ~30% of CF patients. While both the CF mouse and ferret gallbladder lack cAMP-inducible chloride currents indicative of CFTR, they have grossly different histopathology. In CF mice, the extent of gallbladder disease is quite variable, with some backgrounds showing evidence of decreased size and inflammatory infiltrates. Although newborn CF ferrets demonstrated little gallbladder pathology, as CF ferrets age, they develop significant mucous plugging and mucosal proliferation (~80%–90%). CF pigs have the most severe gallbladder disease, presenting with a microgallbladder at birth. Interestingly, rats lack a gallbladder, and thus CF-associated disease in this organ is irrelevant in this species.
Vas Deferens Phenotype
Nearly all male adults with CF suffer from infertility caused by bilateral absence of the vas deferens. While CF male mice have normal fertility, newborn CF rats, ferrets, and pigs lack a vas deferens bilaterally and thus appear to model this component of the human disease.
Cystic Fibrosis-Related Diabetes
Cystic fibrosis-related diabetes (CFRD) is a common complication of CF and affects 20%–25% of adolescents and 40%–50% of those older than 30 years of age There have been several reports suggesting that CFTR plays a functional role within the beta-cell to facilitate insulin secretion. Furthermore, chemical-induced killing of beta-cells in CF mice exacerbates islet cell dysfunction and leads to an altered inflammatory response. By contrast, other findings have suggested that CF mice harboring the F508del- CFTR variant do not have an intrinsic beta-cell secretory defect, but rather acquire insulin resistance and a reduced beta-cell mass with age. Interestingly, lung bacterial clearance in diabetic CF mice, following streptozotocin (STZ)-induced beta-cell injury, is significantly diminished compared with nondiabetic CF mice.
While CF mice appear to have some abnormalities that affect glucose metabolism, their lack of significant exocrine pancreatic disease is likely the reason they do not develop classical CFRD seen in humans. Both CF ferrets and pigs are born with impaired glucose tolerance (IGT). IGT in newborn CF pigs appears to be due to a reduced glucose-stimulated insulin secretion. By contrast, IGT in newborn CF ferrets appears to be due to a lack of first phase insulin secretion despite a greatly accentuated later phase. However, the poorly regulated insulin secretion in newborn CF ferrets does not appear to be due to insulin resistance. These early differences in glucose tolerance between the two models are likely due to the more severe exocrine pancreatic damage and islet remodeling at birth in the CF pigs, as compared with CF ferrets.
The CF ferret model has been most extensively studied in terms of CFRD pathogenesis. Isolated islets from newborn CF ferrets have reduced glucose-stimulated insulin secretion due to a heightened level of insulin secretion in the presence of low glucose, demonstrating that islet-intrinsic defects in insulin secretion exist in CF ferrets. This dysregulated insulin secretion by CF ferret islets appears to mirror that seen in nursing newborn CF ferrets and is thought to be a result of the emerging inflammation at birth in the pancreas. Recently, rising pancreatic inflammation in juvenile CF ferrets has been shown to significantly reduce beta-cell mass and lead to spontaneous diabetic-level hyperglycemia at 1–2 months of age, a time at which proinflammatory cytokines (IL-1β, TNFα, IL-6, CXCL10) peak in the pancreas. Interestingly, at 2–3 months of age, CF ferrets undergo a rapid recovery in their ability to regulate blood glucose following a meal with a coincident increase in beta-cell mass and islet cell function. This novel finding of productive islet remodeling following peak exocrine inflammation in the CF ferret pancreas was associated with a fibrotic to adipogenic transition, marked by elevation in PDX1, PPARγ, and adiponectin. Such findings suggest that the early CF pancreas has a remarkable ability to repair damaged islets, although formal proof of regenerating beta-cells is still lacking. Despite this unique “honeymoon” period observed in CF ferrets following glycemic crisis, with age they go on to develop the more classically studied CFRD phenotype in CF patients with impaired insulin secretion in response to hyperglycemic clamp and to arginine. However, insulin sensitivity was normal, as measured by using a euglycemic hyperinsulinemic clamp. An interesting clinically relevant observation from this study was that mixed meal tolerance was impaired at all ages in CF ferrets, while oral glucose intolerance was not detected until 4 months.
Characteristics of Systemic Disease
Airways, Upper and Lower
Lung disease remains the major expression of morbidity and mortality in CF. Infants with CF are born with normal lungs, and pulmonary disease develops over a variable time course. The earliest lesion is obstruction of the small airways by abnormally viscous airway mucus. A secondary bronchiolitis with plugging of the airways invariably follows and develops into bronchiectasis as the respiratory epithelium becomes chronically infected. A striking feature of CF lungs is that the parenchyma is virtually untouched for much of the course, while the airways are severely afflicted. The airways become a reservoir for chronically infected mucopurulent secretions, first by Staphylococcus aureus and Haemophilus influenzae, and later by a distinctive mucoid form of P. aeruginosa. Once mucoid P. aeruginosa colonizes the lungs, it is virtually impossible to eradicate. Emergence of methicillin-resistant strains of S. aureus (MRSA) is increasing and a cause for concern. Respiratory failure is the major cause of death, with the median life span now increased to 41.6 years in the United States (Cystic Fibrosis Foundation Registry Data, 2015).
The respiratory disease is usually progressive, with superimposed acute exacerbations. Cough is an early symptom and may be nonproductive and mistaken for asthma. Production of sputum develops and is an indicator of chronic inflammation and infection. A typical course is characterized by intermittent acute pulmonary exacerbations, in which there is an increased volume of sputum, a change in the color of sputum, decreased exercise tolerance, and weight loss. Digital clubbing is a universal finding with progression of the lung disease. The chest radiograph becomes abnormal early in the course of the disease and demonstrates hyperinflation ( Fig. 49.3 ) and patchy atelectasis ( Fig. 49.4 ). The chest computed tomography (CT) scan is even more sensitive to changes ( Fig. 49.5 ) and may be preferred over the chest radiograph for a quantitative assessment of progression of lung disease. Bronchiectasis develops from progressive chronic infection and destruction of the airways ( Fig. 49.6 ). Pulmonary function tests early in the disease reflect small airways obstruction but then progress toward a decrease in the forced expiratory volume in 1 second (FEV 1 ) and increases in residual volume, functional residual capacity, and the ratio of residual volume to total lung capacity. The vital capacity is eventually reduced. With treatment of the acute exacerbations, variable improvements in some of these lung functions can be documented.

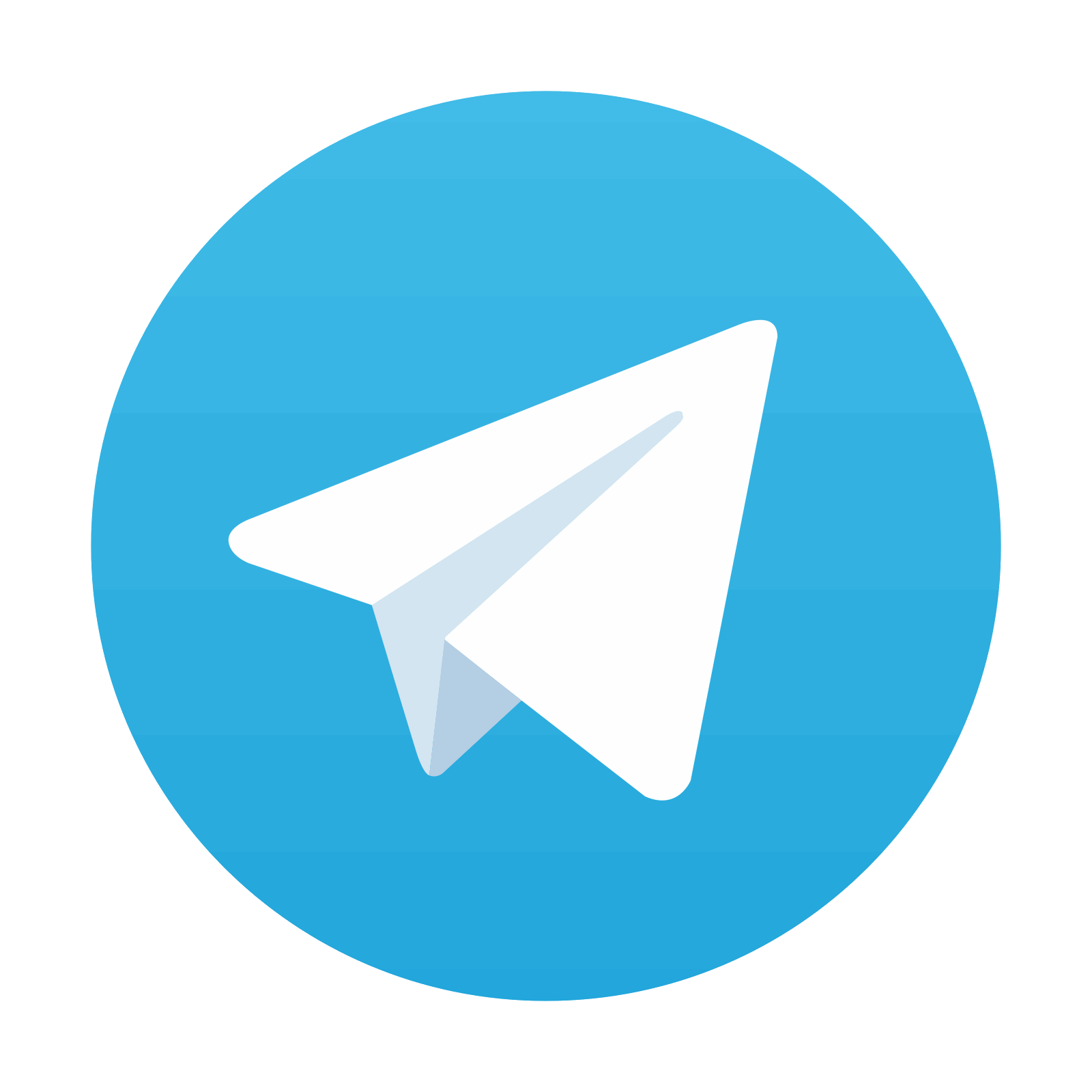
Stay updated, free articles. Join our Telegram channel

Full access? Get Clinical Tree
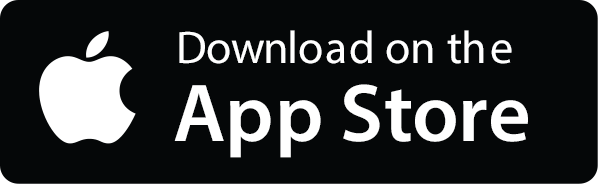
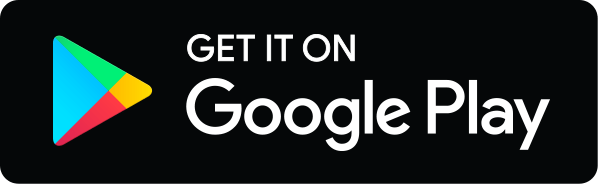
