General Techniques in Molecular Cardiology
Burns C. Blaxall
Mark B. Taubman
Historical Perspective
A series of critical experiments in the 1950s and early 1960s established the central dogma of molecular biology: deoxyribonucleic acid (DNA) is the genetic material responsible for transmitting heritable information; DNA is “transcribed” into messenger ribonucleic acid (mRNA); and mRNA is then “translated” into proteins. However, several crucial discoveries were required before detailed analyses of these processes could be routinely made. One of these was the identification of DNA-processing enzymes from bacteria and bacteriophages. These enzymes included the following: restriction endonucleases, which cleave DNA at sequence-specific sites; polymerases, which synthesize new DNA from a DNA template; and ligases, which can join two pieces of DNA together. This technology ushered in the era of recombinant DNA, thereby allowing small pieces of DNA to be isolated, sequenced, ligated to other pieces of DNA, radiolabeled, and used as molecular probes.
The discovery of the enzyme reverse transcriptase had a particularly great impact on the field of molecular biology. Reverse transcriptase synthesizes DNA from an RNA template, thus generating a strand of DNA complementary to the mRNA. The ability to synthesize complementary DNA (cDNA) from mRNA isolated from a specific tissue was critical to the development of cDNA libraries, ushering in the era of molecular cloning. Other important technical advances included the development of nucleic acid and protein gel electrophoresis to separate different species by size, systems for “blotting” nucleic acids and proteins on filters so they can be subjected to a variety of analyses, the development of “hybridization” techniques to identify specific DNA and RNA molecules, and the advent of DNA sequencing. The development of viral and plasmid “vectors” that are designed to introduce DNA into a variety of cells where it can be transcribed into mRNA and then translated into proteins has provided powerful tools for the functional analysis of specific genes as well as the generation of recombinant proteins, some of which are now routinely used in clinical medicine. The development of appropriate experimental models was essential to the application of molecular techniques to basic investigation in cardiovascular disease. A critical breakthrough was the establishment of culture systems for studying the individual cellular components of the cardiovascular system. The ability to study each cell type as an essentially “pure” culture provided the initial stimulus for a vast array of studies that examined cell surface receptors, transmembrane signals, gene regulation, and the pathways involved in growth, migration, and control of shape. The breakthroughs in tissue culture techniques have been paralleled by the development of a variety of animal models of cardiovascular disease. These include models of vessel injury, heart failure, and dyslipidemia, as well as animals with genetic preponderance toward hypertension, atherosclerosis, and other cardiac diseases. These in vivo models have allowed investigators to verify findings in culture using a more clinically relevant setting. The ability to generate “transgenic” animals—animals whose genetic makeup has been altered using recombinant DNA techniques—has allowed investigators to examine the pathophysiologic function of specific genes in in vivo models and to develop small animal models that more closely mimic human disease.
The recent sequencing of entire mammalian genomes has revolutionized our ability to investigate global sequence variation and differential gene expression in cardiovascular disease, thus ushering in the era of genome-wide, or “omic” investigation. Whereas previous approaches often focused on a small subset of genes or molecules, investigators can now survey the entire genome for functionally related or disease-related genes and proteins. This chapter and others in this section describe numerous methods of “omic” investigation, including genomics, functional genomics, and proteomics.
This chapter is designed as an overview of some of the major molecular and cellular techniques used in contemporary cardiovascular research. The revolution in molecular biology over the previous century has provided the tools to examine biologic processes at their most basic level. As is evident throughout this text, the application of molecular techniques to cardiovascular research has led to major advances in our understanding of the pathobiology of cardiovascular disease. Although the techniques described are widely used in many areas of biologic investigation, our focus is on their application to cardiovascular investigation; as such, we hope that it provides a frame of reference for understanding the experiments described in the other chapters of this book. The following portions of this chapter and others in this section describe the cell culture and in vivo models that have been developed to allow the application of basic molecular techniques to the study and modulation of gene expression in the context of cardiovascular disease.
Models Used in Cardiovascular Research
The development of appropriate experimental models was critical to the application of molecular techniques to cardiovascular research. Hundreds of models have been developed over the past few decades to help further our understanding of cardiovascular pathology. This section provides an overview of some of the models that are most commonly used.
Cell Culture
Cardiovascular diseases involve complex interaction among multiple cell types. As discussed in later sections, state-of-the-art techniques are being increasingly refined to allow gene expression to be monitored in individual cells in their natural milieu. In spite of these advances, the ability to examine pure populations of cells in an environment that can be experimentally controlled has provided a wealth of information about the biologic properties of each cell type and continues to be a powerful approach. The relevance of data obtained from cell culture systems to the intact organism must be established experimentally. Hence, the ability to move rapidly from cell culture, to animal models, to clinical studies, and back is an important feature of contemporary molecular cardiology. The following paragraphs outline some of the procedures that are used to study cells in culture, the problems inherent in such cell culture systems, and more recent approaches to addressing cell-cell interactions.
Endothelial Cell Culture
Studies on vascular endothelium have extensively availed themselves of cell culture. Endothelial cells are present as a single layer lining the intima. Therefore, only small amounts of endothelial cells can be obtained from a single blood vessel. These cells also tend to develop senescence more quickly than other types, such as smooth muscle, and therefore provide a greater challenge in obtaining large numbers of cells. A favorite choice for endothelial cell culture has been the human umbilical vein endothelial cell. This cell can usually be passaged four to five times, and large numbers of cells can be obtained from individual placentas (1). The ready availability of placentas has been instrumental in the popularity of these cells. Other sources include arterial and microvascular endothelial cells from bovine, rat, and human sources. Unlike smooth muscle cells, endothelial cells grow poorly on plastic or glass and are usually grown on a matrix of gelatin, collagen, or fibronectin.
Two particularly important properties of cultured endothelial cells have received considerable attention. The first is the ability of endothelial cells to respond directly to shear or turbulence (2). This has led to the development of a variety of cell culture systems to study endothelial cell biology under various flow conditions. The second property is the ability of microvascular endothelial cells to form capillaries under appropriate cell culture conditions (3). This has allowed investigators to use cell culture to model capillary formation, such as that seen in tumor angiogenesis and in angiogenesis associated with ischemia.
Cardiac Myocyte Culture
Cardiac myocytes isolated by enzymatic digestion of neonatal rat or mouse hearts maintain their contractile phenotype in culture and have been particularly useful for electrophysiologic as well as molecular biologic studies (4). Adult cardiac myocytes have also proven to be a very useful model, and they avoid the potential complication of the “fetal gene expression program of heart failure,” which is absent in healthy adult cardiac myocytes but is present in neonatal cardiac myocytes and in myocytes derived from hypertrophied or failing myocardium. Technology exists to analyze both contractile function and intracellular calcium concentration concurrently in adult cardiac myocytes, thus providing an ex vivo/in vitro model to investigate the functional role of specific genes and molecules in cardiomyocytes isolated from both healthy and diseased cardiac tissue. Cardiac myocytes isolated from late stages of development do not divide in culture and therefore can only be used as primary cultures. Isolation of relatively pure populations of myocytes requires mechanical and enzymatic disruption, dissection to isolate the chamber of interest (usually the left ventricle), and serial plating or density centrifugation to remove fibroblasts (which grow rapidly in culture, so many investigators utilize growth-inhibitory compounds to prevent proliferation of any remaining fibroblasts). The passage of cardiac myocytes in serial culture has thus far been limited to transformed or cardiac tumor–derived cells (e.g., H9C2, HL-1) that show varying and often disappointing degrees of myocyte structure and differentiation in culture (5).
Other Cell Types
The cell types described previously in this section represent a selection of the more commonly studied cells in the cardiovascular literature. The list is by no means exhaustive. Most of the cell types involved in the pathogenesis of cardiovascular diseases, including fibroblasts, lymphocytes, macrophages, and circulating stem cells, can be grown in culture. The amplification and use of stem cells in the study and treatment of cardiovascular disease are discussed in detail in Chapter 101.
In Vivo Models
Despite the invaluable contribution of cell culture, the utility of molecular cardiology to improve the treatment of human disease is dependent on the development of animal models that accurately mimic these diseases. Although naturally occurring animal models of cardiovascular disease have been exploited, many of the common human diseases are rarely seen in animals. In recent years, the increasing application of transgenic technology to cardiovascular research has allowed the development of more sophisticated mouse models of cardiovascular pathology. Although the small size of the mouse presents challenges, various diagnostic techniques have been adapted for its use, including echocardiography (6,7), micromanometry (8), in vivo magnetic resonance imaging (9), and micropositron emission tomography (microPET) (10).
Atherosclerosis
One model that is commonly used to study atherosclerosis and arterial injury involves the use of balloon catheters to denude the endothelium and to injure the media (11). The approach to catheterization is similar to that used in human angioplasties, involving cannulation of a distal artery and passage of a balloon-tipped catheter or, in mice, a wire into the area to be injured. Injuries can range from gentle endothelial denudation to injuries that tear the internal elastic lamina and damage the media. Alternative approaches include external injury to the artery, for example, using forceps to crush the artery (12,13), or internal injury using air (14) or electrical stimulation (15). Such models have been used in rats, rabbits, transgenic mice, dogs, pigs, and nonhuman primates. The hallmark of this model is the development of intimal hyperplasia, characterized by the migration and proliferation of smooth muscle cells in the intima. An example is shown in Figure 93.1.
Balloon injury models have been instrumental in elucidating the early molecular and cellular events following vessel injury and have also been used widely for studying approaches to inhibit intimal hyperplasia. Because intimal hyperplasia is a major component of the atherosclerotic plaque and is thought to play a role in restenosis after angioplasty, balloon injury models have received considerable attention. One criticism of most models is that they involve the use of normal vessels, whereas atherosclerosis occurs and angioplasty is performed in the setting of abnormal vessels that contain lipid-rich, calcified plaques. Studies that use injury to normal vessels have contributed greatly to understanding cell biology in vivo but have been less useful for predicting the effects of pharmacologic interventions in human disease.
In addition to acute arterial injury, various in vivo systems have been used to model the more chronic development of atherosclerosis, such as that seen in human disease. These have included the use of high-fat/high-cholesterol diets in rabbits (16), pigeons (17), pigs (18), and nonhuman primates (19,20,21). Although these diets are often associated with the types of lesions seen in early atherosclerosis, these lesions are not as severe as those seen in human disease (22). A variety of genetically engineered hyperlipidemic transgenic mice that more closely resemble the complex lesions of human atherosclerosis has been developed (23). The study of genetically altered mice has led to dramatic advances in the study of lipoprotein metabolism and atherosclerosis (24). In spite of these advances, challenges still remain. A critical feature of human unstable coronary syndromes is the rupture of atherosclerotic plaques, and the lack of plaque rupture, except in unusual circumstances, is a shortcoming of the dyslipidemic animal models.
Heart Failure
Various large animal models that were developed to study the hemodynamic aspects of heart failure have been modified for use in small rodents. Recent advances in noninvasive echocardiographic imaging and in invasive hemodynamic methods provide more accurate analysis of the cardiovascular effects of a specific surgical or genetic manipulation or intervention. Aortic banding is a well-established model of left ventricular (LV) hypertrophy resulting from pressure overload. The progression of LV hypertrophy to progressive LV dysfunction and overt heart failure has been documented in guinea pigs and rats. Similar effects have been observed following renal artery ligation (25) and after the administration of a high-salt diet to Dahl salt-sensitive rats (26
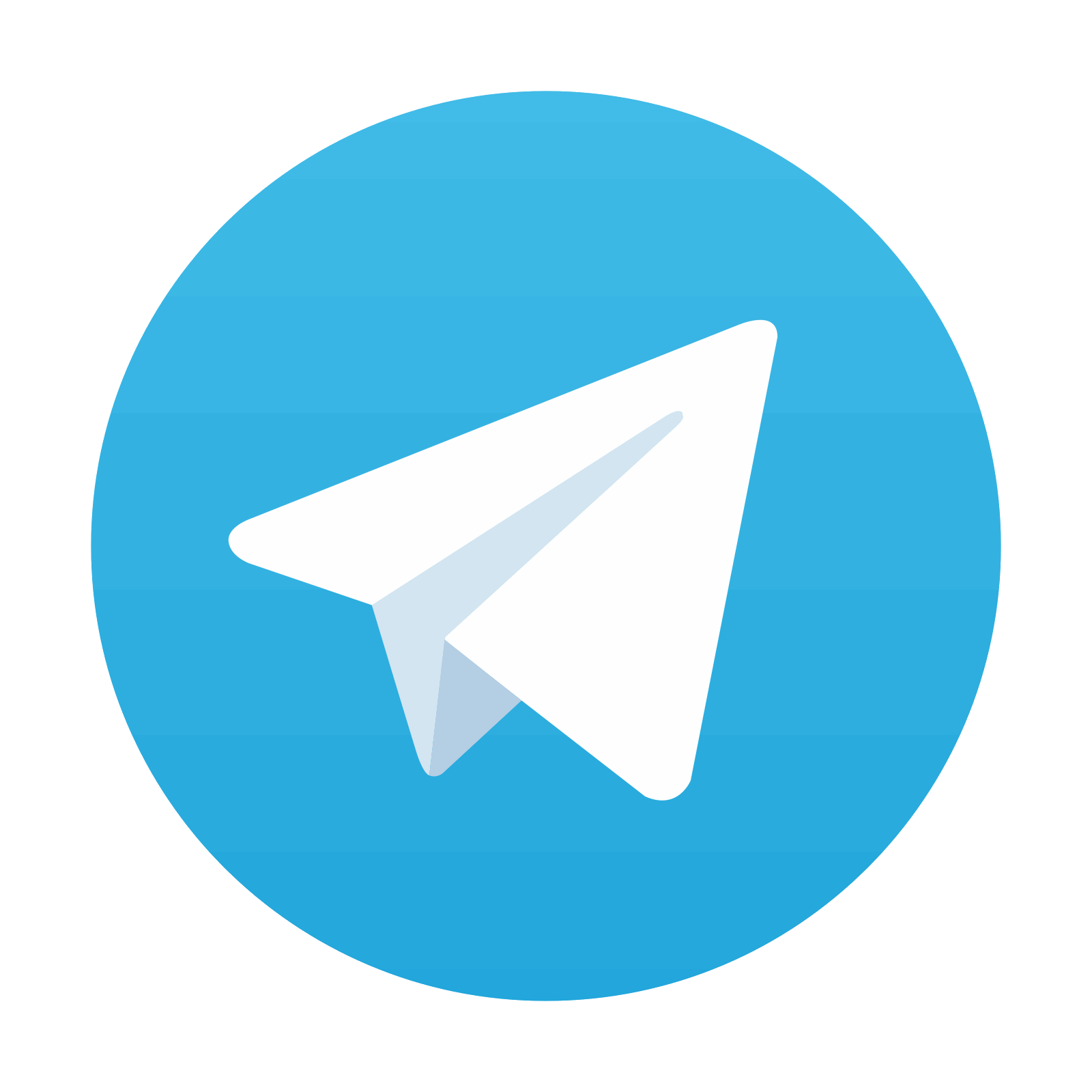
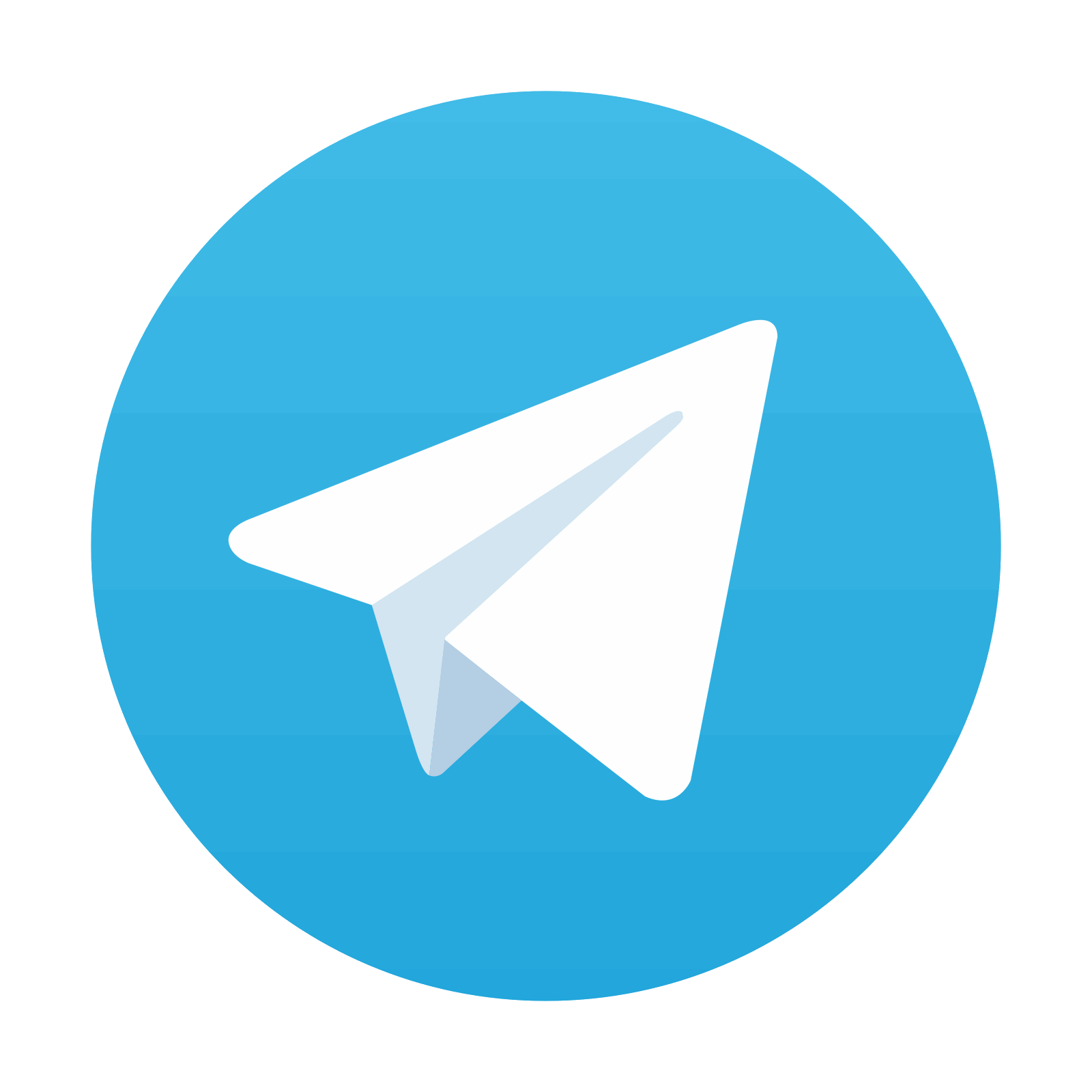
Stay updated, free articles. Join our Telegram channel

Full access? Get Clinical Tree
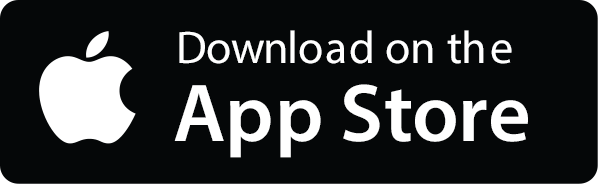
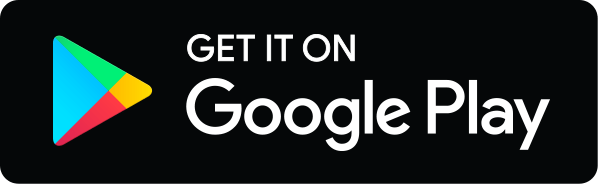
