Background
Three-dimensional (3D) speckle-tracking echocardiography (STE) for myocardial strain imaging may be superior to two-dimensional STE, especially with respect to rotational mechanics. Automated strain measurements from nonstitched 3D STE may improve work flow and clinical utility. The aim of this study was to test the feasibility of model-based 3D STE for the automated measurement of voxel circumferential strain (E cc ) and myocardial rotation.
Methods
Thirty-five individuals (12 healthy volunteers, 12 patients with dilated cardiomyopathy, and 11 patients with hypertensive left ventricular [LV] hypertrophy) were prospectively studied. The latter two groups did not have significant coronary artery disease on coronary arteriography. Tagged cardiovascular magnetic resonance (CMR) and feature-tracking CMR were used as reference standards. Regional (apex and mid left ventricle) and slice (within a region) E cc and rotation were measured by real-time volume transthoracic echocardiography (nonstitched) using an automated algorithm.
Results
Compared with both CMR techniques, apical and mid-LV E cc (concordance correlation coefficients [CCCs], 0.84–0.95 and 0.48–0.68) and rotation (CCCs, 0.70–0.95 and 0.42–0.68) showed excellent, good, and moderate agreement, respectively. At the LV base, rotation showed poor agreement with CMR methods (CCC, 0.04–0.21), consistent with previous descriptions, but calculated LV twist showed moderate to good correlation with CMR techniques (CCC, 0.61–0.84). However, the 95% CI for measurements between techniques was wide, emphasizing the challenges in comparing voxel deformation by 3D echocardiography with CMR, compounded by differences in approaches to measuring deformation, and matching regional and slice measurements between techniques. Reproducibility ( n = 10, including test-retest variability) of automated 3D strain and rotation measurements was good to excellent (coefficient of variation < 10%) and was comparable with that of CMR methods (coefficient of variation < 10%) in the same patients.
Conclusions
The data from this study show that automated measurements of voxel rotational mechanics by real-time volume transthoracic echocardiography is feasible and comparable with tagged CMR and feature-tracking CMR strain measurements, albeit with wide limits of agreement, emphasizing the differences between the modalities. Furthermore, this automated 3D speckle-tracking echocardiographic approach shows excellent reproducibility, including test-retest variability, comparable with that of the CMR methods.
Highlights
- •
This is the first clinical validation of model-based, automated, voxel strain imaging using real-time 3D (nonstitched) echocardiography.
- •
The authors measured regional and slice 3D rotational mechanics and compared this to tagged and feature-tracking CMR.
- •
This novel automated 3D strain technique is accurate and reproducible (including test-retest variability) compared with CMR.
- •
Automation may enhance work flow and clinical adoption and has a positive impact on reproducibility.
Graphical abstract

Two-dimensional (2D) myocardial strain measurements by speckle-tracking echocardiography (STE), especially global longitudinal strain (GLS), have emerged as an important tool to measure myocardial function in overt and occult left ventricular (LV) dysfunction. Improved standardization of strain measurements have further enhanced the clinical applicability of myocardial strain. A particular advantage of three-dimensional (3D) speckle-tracking myocardial strain is the improved accuracy of rotational strain measurements compared with 2D STE. But applications of 3D STE thus far have involved electrocardiographically gated subvolumes to construct the entire volume of the left ventricle, which limits clinical applicability and adoption and potentially affects the accuracy of 3D strain measurements. Furthermore, manual identification of the myocardial borders is time consuming, is prone to errors, and may have adversely affect the reproducibility of measurements.
Thus, we tested the feasibility of the automated measurement of voxel rotational myocardial mechanics using a model-based 3D speckle-tracking echocardiographic algorithm applied to real-time, full-volume transthoracic echocardiography (RT-VTTE) (nonstitched acquisition) in normal volunteers and patients with abnormal left ventricles. We used tagged and feature-tracking cardiovascular magnetic resonance (CMR) imaging techniques as reference standards. Although tagged CMR (TAG-CMR) is the conventional reference standard to measure myocardial mechanics, feature-tracking CMR (FT-CMR) is more practical and is being increasingly used in routine CMR practice as an alternative to TAG-CMR.
Methods
Patient Population
We included healthy volunteers, patients with dilated cardiomyopathy (DCM), and patients with LV hypertrophy (LVH) with normal LV ejection fractions (EFs). Normal volunteers were those with normal results on resting electrocardiography and transthoracic echocardiography, no histories of cardiac disease, no cardiopulmonary symptoms, and no risk factors for coronary artery disease (CAD). Patients with DCM were those without significant epicardial coronary artery obstructions who had stable heart failure symptoms. Patients with LVH included those with controlled systemic hypertension without any heart failure symptoms or epicardial CAD. The absence of significant epicardial CAD was established by coronary arteriography done as part of clinically indicated evaluation of heart failure in the DCM group and in the LVH group as part of evaluation of chest pain or dyspnea or abnormal stress test results. The study was approved by the institutional review board at the PLA General Hospital, and all participants consented to the study.
Transthoracic Echocardiography
Standard, comprehensive 2D transthoracic echocardiographic studies (SC2000, 4V1c, 3.5 Hz harmonic; Siemens Medical Solutions USA, Mountain View, CA) were performed in all individuals. LV internal dimension in end-diastole and end-systole, septal and posterior wall thickness, and EF by the biplane Simpson method were measured. Real-time volume imaging (nonstitched) of the left ventricle in the four-chamber orientation (RT-VTTE) was then performed using the 4Z1c transducer (3.5 Hz harmonic). Imaging depth, volume sector size, and imaging mode (harmonic vs fundamental) were optimized to maximize temporal resolution, and three consecutive cardiac cycles were acquired. The blood pressure (BP) and heart rate (HR) was recorded during the study.
CMR
A 3.0-T magnetic resonance imaging scanner (GE Healthcare, Milwaukee, WI) was used, and standard steady-state free precession cine CMR sequences were performed in all subjects to obtain a short-axis (SAX) stack of the left ventricle from the base to the apex. Typical imaging parameters were as follows: echo time, 1.5 msec; repetition time, 3.5 msec; flip angle, 45°; field of view, 360 mm; slice thickness, 8 mm; slice gap, 2 mm; 20 phases per cardiac cycle; matrix size, 256 × 256; and reconstructed pixel size, 1.41 mm/1.41 mm. The temporal resolution of the cine steady-state free precession images ranged from 30 to 40 msec. For TAG-CMR, a standard grid spatial modulation of magnetization CMR sequence was performed in all individuals to derive detailed motion information of the myocardium in selected SAX slices. Typical parameters for this were as follows: fast cardiac gated gradient-echo sequence (echo time, 1.9 msec; repetition time, 4 msec; flip angle, 15°; field of view, 360 mm; slice thickness, 7 mm; 20 phases per cardiac cycle; matrix size, 256 × 256; and reconstructed pixel size, 1.41 mm/1.41 mm) and spatial modulation of magnetization activated with tag spacing of 7 mm. The basal, middle, and apical SAX slices were positioned at 25%, 50%, and 75% of the distance between the mitral valve annulus and the apex on an LV four-chamber view, respectively. Acquisition time was approximately 15 to 20 sec/slice, and temporal resolution was 30 to 40 msec.
All echocardiographic and CMR imaging studies were done on the same day to minimize the effect of changes in clinical conditions and hemodynamics on the measured parameters.
Three-Dimensional Regional and Slice Strain Analysis
A detailed description and validation of the model-based approach to myocardial segmentation and deformation analysis has been previously published. A prediction-based collaborative tracker algorithm (eSie Mechanics; Siemens Medical Solutions USA) was used to track the myocardium in the 3D volume data set. Marginal space learning was used to identify the epicardial and endocardial borders in the first frame (end-diastole). Then cues from speckle patterns, image gradients, template trackers, detection trackers, LV shape, and learned motion patterns are fused into a single Bayesian function to improve myocardial tracking accuracy and robustness. The prediction-based collaborative tracker algorithm used in this version of the software was able to process one 3D volume data set (160 × 148 × 208 = 4,925,440 voxels) in approximately 1 to 1.5 sec, requiring about 20 sec for dynamic tracking through the entire cardiac cycle. On the basis of this dense 3D myocardial tracking, voxel displacement and velocity were measured in both Cartesian and local cardiac coordinate systems. Manual changes to the tracked myocardium were possible if the observer disagreed with the automated tracking. Then the length of the volume of the left ventricle was divided into three equidistant regions (apex, middle, and base), as shown in Figure 1 . Circumferential strain (E cc ) and rotation were measured for the entire apex and the mid left ventricle and constituted the 3D echocardiographic regional measurements. Each region was then subdivided into three equidistant slices, and 3D slice E cc and rotation were measured at the LV apex, the mid left ventricle, and the base (rotation only) using the midslice in each region ( Figure 1 ). LV twist was calculated as the difference between apical and LV basal rotation. For all 3D speckle-tracking echocardiographic measurements, we first identified data that had three consecutive beats and was free of artifacts from excessive cardiac translation or ectopic beats. If we did not find such data, we used data in which there were at least two beats from which we could average the measurements. We were able to use three-beat averages for all measurements except in one patient with DCM, in whom we used two-beat averages.

FT-CMR and TAG-CMR Analysis
Steady-state free precession SAX images were used for FT-CMR analysis, as previously described. The endocardial border was manually traced in a single frame, and the feature-tracking algorithm (Velocity Vector Imaging; Siemens Medical Solutions USA) automatically tracked the endocardial border in all the frames of the cardiac cycle. Then perpendicular and clockwise-counterclockwise displacement of 5-pixel-wide equidistant points was tracked along the endocardial contour. The final 2D vector display represented the myocardial displacement in all directions in the scan plane, with the length of the arrow representing the magnitude of frame-to-frame displacement (velocity) and the direction of the vectors representing the predominant direction of displacement: radial and circumferential in the SAX view. TAG-CMR analysis was done in the SAX images corresponding to those used for FT-CMR using a template-based tracking algorithm that was adapted to derive the displacement vectors of the crossing points on the tagging grids, where the appearance template at each crossing point was modeled using two tunable Gabor filters. The coordinates of the crossing points were further smoothed and interpolated spatially and temporally by a cubic B-spline function. The myocardial displacements were thus calculated through subtraction and were further transformed from the Cartesian to the polar coordinates, from which the myocardial rotation and E cc were calculated at the inner half of the myocardium (“endocardium”). LV twist was calculated as the difference between apical and LV basal rotation.
Interobserver, Intraobserver, and Test-Retest Variability
For inter- and intraobserver variability analysis, the initial 3D echocardiographic and CMR data from the 10 patients who consented to test-retest studies were used. These included data from four normal volunteers and three patients each from the DCM and LVH groups. For the variability analysis, measurements were made using the same cardiac cycles used for the initial measurements. For test-retest variability, data from the initial study (which were used for observer variability analysis) and subsequent study were used. The specific data that were chosen from the retest for test-retest variability analysis were based on similar criteria as the test (initial) data: averages of three cardiac cycles except in one patient with DCM, in whom we used averages of two beats because of ectopic beats. Two observers (X.Z. and H.H.) analyzed all 3D echocardiographic data independently. Observers Z.Q. and S.L. independently analyzed all CMR data. For intraobserver reproducibility, the observers analyzed the data 1 week apart and were blinded to the data from the first read and to the clinical data.
Statistical Analysis
Normality for each variable was tested using a combination of quantile-quantile plots and the Kolmogorov-Smirnov test. Depending on the normality, variables are expressed as mean ± SD or as median and interquartile range. Concordance correlation coefficients (CCCs) were used to describe the correlations between various echocardiographic and CMR techniques, while the Bland-Altman method was used to assess agreement. From the Bland-Altman plots, the bias and 95% limits of agreement (LOA) are reported. The 95% LOA was calculated as the average difference ± 1.96 SDs of the differences. The Wilcoxon rank sum test was used to compare continuous data between imaging methods. The Kruskal-Wallis test was used to compare strain measurements among normal control subjects, patients with LVH, and patients with DCM. Inter- and intraobserver variability was assessed using CCCs and coefficients of variation (COVs). P values < .05 were considered to indicate statistical significance, but in cases of multiple comparisons among three groups, P values < .0167 was considered to indicate significance. All statistical analyses were performed using MedCalc version 11.4.2 (MedCalc Software, Mariakerke, Belgium).
Results
Study Population
A total of 24 normal volunteers, 17 patients with DCM, and 19 patients with systemic hypertension were screened, among whom adequate acoustic windows for RT-VTTE were available in 20 (83%), 14 (82%), and 15 (79%) individuals, respectively. From this total of 49 eligible individuals, only 36 patients (12 in each group) consented to undergo CMR studies. However, one patient with LVH chose not to undergo CMR after undergoing 3D echocardiography, so complete data for 35 patients were available for analysis. The mean ages of the patients in the normal, DCM, and LVH groups were 27.6 ± 6.9, 49.4 ± 16.1, and 52.5 ± 6.6 years, respectively ( P < .0001, one-way analysis of variance [ANOVA]). The mean LV EFs in the groups were 56.1 ± 3.5%, 26.1 ± 6.8%, and 57.9 ± 7.7%, while the mean LV septal wall thicknesses were 9.5 ± 1.1, 9.3 ± 1.4, and 13.4 ± 1.0, respectively ( P < .0001, one-way ANOVA for both EF and wall thickness). Mean BPs were 115 ± 11/65 ± 9 mm Hg, 108 ± 7/64 ± 6 mm Hg, and 128 ± 9 mm Hg ( P < .0001, one-way ANOVA) in the normal, DCM, and LVH groups. Mean HRs were 76 ± 6, 61 ± 9, and 73 ± 8 beats/min ( P < .001, one-way ANOVA).
Imaging Data
The mean volume rate for RT-VTTE was 22.7 ± 3.9 volumes/sec (range, 20–36 volumes/sec), and the frame rate for CMR was 20 frames/sec. The mean time taken for acquisition on RT-VTTE was 5 ± 3 min (range, 1–5 min), depending on acoustic window and need for breath holding, while the mean time for CMR data acquisition was 27 ± 7 min. The average time taken for automated 3D strain analysis was about 1 min, but in three LVH and two DCM patients in whom automated tracking of the apical myocardium needed manual adjustment, it took up to 3 min to complete the analysis. FT-CMR analysis took 9 ± 3 min and TAG-CMR analysis took 12 ± 5 min. The mean BP at the time of the first study was 130.5 ± 25.5/70.5 ± 14.5 mm Hg, and the mean HR was 80.5 ± 5 beats/min. Repeat imaging for test-retest assessment was done 1 ± 0.6 days later, and the mean BP and HR during repeat testing were 129 ± 22/67.5 ± 15.5 mm Hg and 77 ± 5 beats/min.
Correlation and Agreement of 3D Echocardiography and CMR for E cc , Rotation, and Twist
Three-dimensional echocardiographic regional and slice E cc at the LV apex and mid left ventricle showed good correlation (CCC, 0.84–0.95) compared with TAG-CMR and FT-CMR ( Table 1 ) in the whole study population ( P < .0001 for all). The two CMR methods also showed good to excellent correlation (CCC, 0.84–0.95) for E cc ( Table 1 ) ( P < .0001 for all). The mean differences (absolute) and the 95% LOA for E cc measurements between the 3D echocardiographic and CMR methods are shown in Table 2 . Both 3D echocardiographic regional (bias [LOA], 1.6% [−10.3 to 13.6] vs TAG-CMR and 2.2% [−10.8 to 15.2] vs FT-CMR) and slice (bias [LOA], 1.4% [−9.4 to 12.2] vs TAG-CMR and 1.9% [−10.4 to 14.2] vs FT-CMR) methods showed higher E cc compared with CMR methods at the LV apex. But at the mid left ventricle, E cc values measured by both 3D echocardiographic methods were similar compared with those obtained by CMR ( Table 2 ). Last, E cc was similar by TAG-CMR compared with FT-CMR (bias [LOA], −0.5% [−14.9 to 13.8] at the apex and −0.2% [−6.4 to 6.0] at the mid left ventricle).
Variable | 3D echocardiography (regional) | 3D echocardiography (slice) | FT-CMR |
---|---|---|---|
E cc (%) ( n = 35) | |||
LV apex | |||
TAG-CMR | 0.85 (0.73–0.92) | 0.89 (0.80–0.94) | 0.84 (0.70–0.91) |
FT-CMR | 0.84 (0.71–0.91) | 0.87 (0.74–0.92) | — |
Mid left ventricle | |||
TAG-CMR | 0.93 (0.88–0.96) | 0.91(0.84–0.95) | 0.95(0.92–0.98) |
FT-CMR | 0.93 (0.87–0.96) | 0.92 (0.84–0.96) | — |
Rotation (°) ( n = 35) | |||
LV apex | |||
TAG-CMR | 0.70 (0.53–0.81) | 0.78 (0.64–0.87) | 0.75 (0.61–0.85) |
FT-CMR | 0.90 (0.81–0.95) | 0.90 (0.82–0.95) | — |
Mid left ventricle | |||
TAG-CMR | 0.42 (0.24–0.58) | 0.44 (0.26–0.59) | 0.68 (0.48–0.82) |
FT-CMR | 0.51 (0.31–0.67) | 0.52 (0.31–0.68) | — |
LV base | |||
TAG-CMR | 0.09 (-0.08–0.25) | 0.04 (-0.10–0.18) | 0.08 (-0.25–0.40) |
FT-CMR | 0.21 (0.04–0.36) | 0.07 (-0.07–0.23) | — |
Twist (°) | |||
Left ventricle | |||
TAG-CMR | 0.63 (0.44–0.77) | 0.61 (0.40–0.75) | 0.73 (0.54–0.85) |
FT-CMR | 0.84 (0.72–0.91) | 0.84 (0.71–0.91) | — |
Variable | 3D echocardiography (regional) | 3D echocardiography (slice) | FT-CMR |
---|---|---|---|
E cc (%) ( n = 35) | |||
LV apex | |||
TAG-CMR | 1.6 (−10.3 to 13.6) | 1.4 (−9.4 to 12.2) | −0.5 (−14.9 to 13.8) |
FT-CMR | 2.2 (−10.8 to 15.2) | 1.9 (−10.4 to 14.2) | — |
Mid left ventricle | |||
TAG-CMR | −0.3 (−7.6 to 6.9) | −0.2 (−8.7 to 8.4) | −0.2 (−6.4 to 6.0) |
FT-CMR | −0.1 (−7.2 to 6.9) | 0.0 (−7.7 to 7.7) | — |
Rotation (°) ( n = 35) | |||
LV apex | |||
TAG-CMR | 1.4 (−7.7 to 10.6) | −1.1 (−5.1 to 2.9) | 2.4 (−5.4 to 10.2) |
FT-CMR | −0.9 (−4.9 to 3.0) | 1.3 (−6.8 to 9.4) | — |
Mid left ventricle | |||
TAG-CMR | −1.2 (−8.0 to 5.7) | −0.5 (−7.3 to 6.3) | 0.2 (−5.8 to 6.2) |
FT-CMR | −1.3 (−6.2 to 3.5) | −0.7 (−5.7 to 4.3) | — |
LV base | |||
TAG-CMR | −2.3 (−8.3 to 3.7) | −2.7 (−8.9 to 3.5) | −0.1 (−8.0 to 7.8) |
FT-CMR | −2.2 (−7.4 to 3.0) | −2.6 (−8.4 to 3.3) | — |
Twist (°) | |||
LV twist | |||
TAG-CMR | 3.7 (−6.0 to 13.5) | 4.0 (−6.3 to 14.2) | 2.5 (−7.1 to 12.0) |
FT-CMR | 1.3 (−5.3 to 7.8) | 1.5 (−5.1 to 8.0) | — |
The correlations between the 3D echocardiographic methods and TAG-CMR and FT-CMR for myocardial rotation ( Table 1 ) were moderate to good at the LV apex (CCC, 0.70–0.95; P < .0001 for all), poor to moderate (CCC, 0.42–0.68; P < .0001 for all) at the mid left ventricle, and poor at the LV base (CCC, 0.04–0.21; 3D regional vs TAG-CMR, P = .29, and vs FT-CMR, P = .013; 3D slice vs TAG-CMR, P = .56, and vs FT-CMR, P = .33). Similarly, the correlation between the two CMR methods ( Table 1 ) was best at the LV apex (CCC, 0.75; P < .0001), modest at the mid left ventricle (CCC, 0.68; P < .001), and poor at the LV base (CCC, 0.08; P = 0.64). The mean absolute difference and the 95% LOA in the rotation measurements between the 3D echocardiographic and CMR methods are shown in Table 2 . At the LV apex, 3D regional rotation was smaller than on TAG-CMR (bias [LOA], 1.4° [−7.7 to 10.6]) and greater than on FT-CMR (bias [LOA], −0.9° [−4.9 to 3.0]). But 3D slice rotation was greater than on TAG-CMR (bias [LOA], −1.1° [−5.1 to 2.9]) and smaller than on FT-CMR (bias [LOA], 1.3° [−6.8 to 9.4]). At the mid left ventricle, both 3D echocardiographic methods provided measures of rotation that were greater than those obtained with the CMR methods ( Table 2 ). Apical LV rotation was smaller by TAG-CMR compared with FT-CMR, but midsegment rotation was similar ( Table 2 ). Basal LV rotation was greater by the two 3D echocardiographic methods compared with the CMR methods, with larger biases between the techniques but similar LOAs as the apex and mid left ventricle ( Table 2 ). Illustrative examples of LV apical and mid-LV measurements are shown in Videos 1 to 3 (available at www.onlinejase.com ).
LV twist measurements showed modest correlation between the two 3D echocardiographic methods and TAG-CMR (CCC, 0.61–0.63) and good correlation compared with FT-CMR (CCC, 0.84; P < .0001 for all) ( Table 1 ). Also, the two CMR methods showed good correlation (CCC, 0.73; P < .0001) ( Table 1 ). The bias and the 95% LOA for the 3D regional and slice methods were smaller compared with FT-CMR than TAG-CMR ( Table 2 ). LV twist by TAG-CMR was greater than by FT-CMR (bias [LOA], 2.5° [−7.7 to 12.0]).
Comparison of 3D Echocardiographic and CMR E cc , Rotation, and Twist in Normal Controls, Patients with DCM, and Patients with LVH
Table 3 shows the mean values for E cc measurements by all methods in healthy volunteers and patients with DCM and LVH. Both 3D echocardiographic regional and slice methods showed no significant differences ( P > .0167 for all) compared with CMR methods in healthy volunteers and in patients with LVH. Illustrative examples are shown in Figures 2 and 3 . Videos 1 and 2 provide stepwise illustration of data acquisition and automated measurements of regional and slice voxel mechanics from 3D echocardiographic and comparable data from FT-CMR and TAG-CMR for the mid left ventricle in a normal subject and a patient with LVH. In patients with DCM, there were no significant differences between 3D echocardiographic methods and TAG-CMR and between the two CMR methods ( P > .0167 for all). There were small but significant differences at the mid left ventricle between the two 3D echocardiographic methods and TAG-CMR ( P < .0167) and between FT-CMR and TAG-CMR ( P < .0167). Figure 4 shows an illustrative example, and Video 3 demonstrates data acquisition and measurements of regional and slice voxel mechanics by all modalities for the mid left ventricle in a patient with DCM. There were, however, no differences between the echocardiographic methods and the FT-CMR method. LV regional rotation and twist, however, showed significant differences between techniques in the three groups ( Table 4 ). When comparisons were made among normal subjects, patients with DCM, and patients with LVH for E cc , rotation, and twist, there were significant differences (summarized in Tables 3 and 4 ) among the groups by all methods studied.
Variable | Normal ( n = 12) | DCM ( n = 12) | LVH ( n = 11) |
---|---|---|---|
E cc (%) | |||
LV apex | |||
3D echocardiography (regional) | −31.5 ± 5.2 | −10.2 ± 4.5 | −31.7 ± 2.5 |
3D echocardiography (slice) | −31.5 ± 4.1 | −9.4 ± 3.7 | −32.4 ± 3.6 |
TAG-CMR | −30.2 ± 5.8 | −10.4 ± 6.2 | −28.8 ± 7.7 |
FT-CMR | −28.7 ± 5.6 | −7.4 ± 3.2 | −32.7 ± 8.4 |
Mid left ventricle | |||
3D echocardiography (regional) | −26.4 ± 3.0 | −8.8 ± 2.5 ∗ | −27.4 ± 4.7 |
3D echocardiography (slice) | −26.2 ± 4.1 | −9.6 ± 3.4 ∗ | −27.3 ± 5.1 |
TAG-CMR | −28.7 ± 2.3 | −6.8 ± 2.1 | −28.1 ± 6.0 |
FT-CMR | −26.8 ± 3.8 | −8.3 ± 2.4 ∗ | −27.9 ± 4.5 |



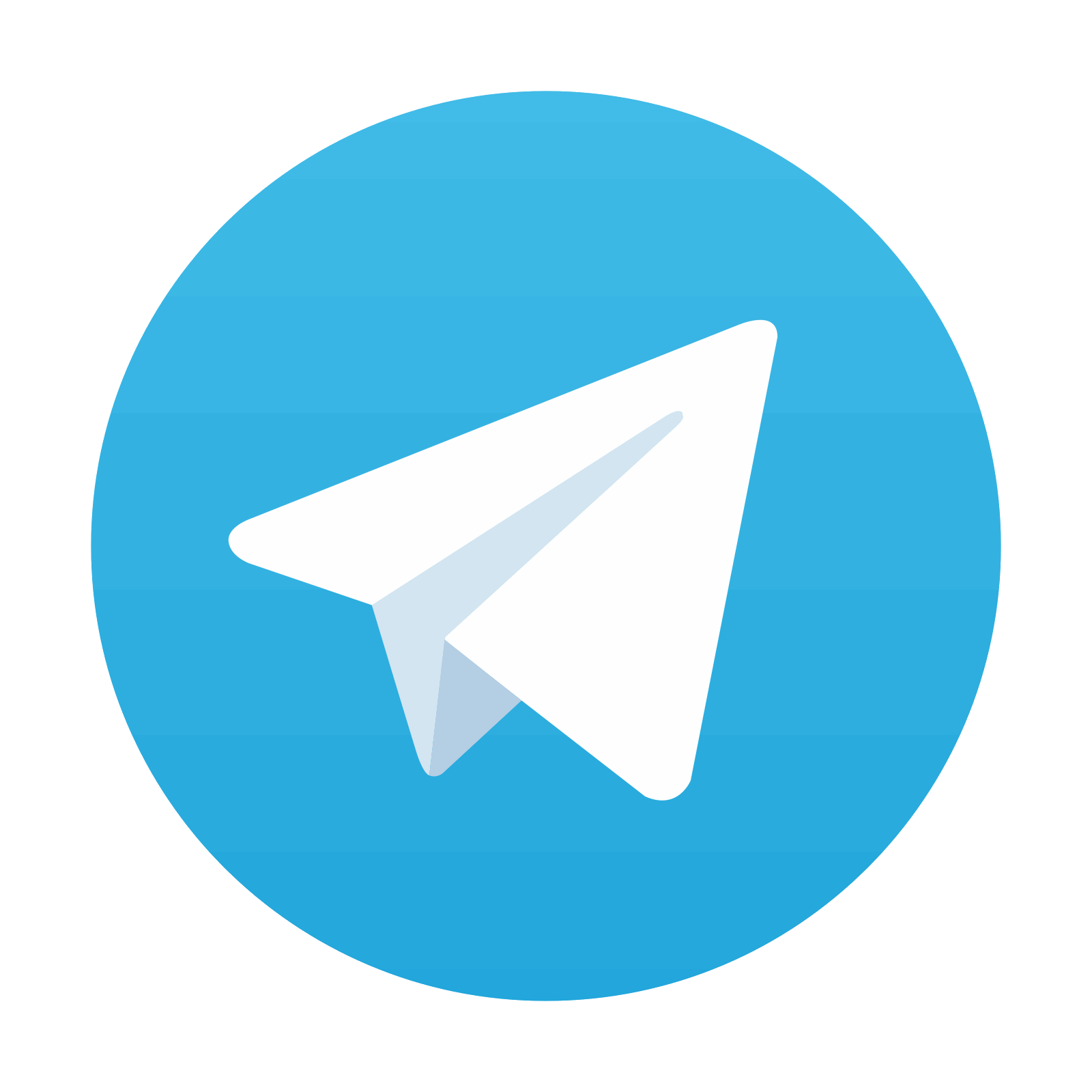
Stay updated, free articles. Join our Telegram channel

Full access? Get Clinical Tree
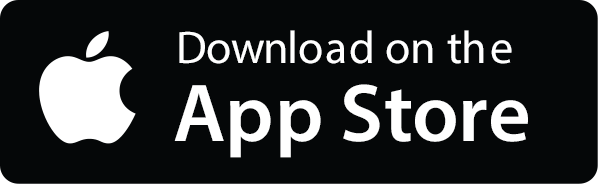
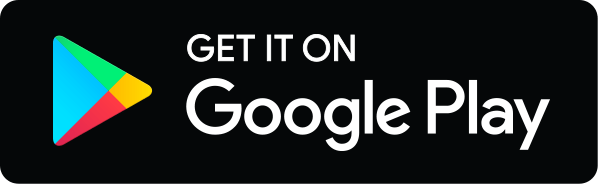
