Background
Right ventricular (RV) systolic function is an important prognostic determinant of cardiopulmonary pathologies in premature infants. Measurements of dominant RV longitudinal deformation are likely to provide a sensitive measure of RV function. An approach for image acquisition and postacquisition processing is needed for reliable and reproducible measurements of myocardial deformation by two-dimensional (2D) speckle-tracking echocardiography. The aims of this study were to determine the feasibility and reproducibility of 2D speckle-tracking echocardiographic measurement of RV peak global longitudinal strain (pGLS) and peak global longitudinal strain rate in premature infants and to establish methods for acquiring and analyzing strain.
Methods
The study was designed in two phases: (1) a training phase to develop methods of image acquisition and postprocessing in a cohort of 30 premature infants (born at 28 ± 1 weeks) and (2) a study phase to prospectively test in a separate cohort of 50 premature infants (born at 27 ± 1 weeks) if the methods improved the feasibility and reproducibility of RV pGLS and peak global longitudinal strain rate measurements to a clinically significant level, assessed using Bland-Altman analysis (bias, limits of agreement, coefficient of variation, and intraclass correlation coefficient).
Results
Strain imaging was feasible from 84% of the acquisitions using the methods developed for optimal speckle brightness and frame rate for RV-focused image acquisition. There was high intraobserver (bias, 3%; 95% limits of agreement, −1.6 to +1.6; coefficient of variation, 2.7%; intraclass correlation coefficient, 0.97; P = .02) and interobserver (bias, 7%; 95% limits of agreement, −4.8 to +4.73; coefficient of variation, 3.9%; intraclass correlation coefficient, 0.93; P < .05) reproducibility, with excellent linear correlation between the two pGLS measurements ( r = 0.97 [ P < .01] and r = 0.93 [ P < .05], respectively).
Conclusions
This study demonstrates high clinical feasibility and reproducibility of RV pGLS and RV peak global longitudinal strain rate measurements by 2D speckle-tracking echocardiography in premature infants and offers methods for image acquisition and data analysis for systolic strain imaging that can provide a reliable assessment of global RV function.
Right ventricular (RV) systolic function is an important prognostic determinant of cardiopulmonary pathologies in premature infants. The recommended methods to quantitatively assess RV systolic function in neonates include tricuspid annular plane systolic excursion and fractional area change in apical four-chamber views, but they require further validation in the neonatal population. RV myocardial performance index has also been used to assess systolic RV function in neonates, but this measure also requires validation, because its impact on management and outcomes remains uncertain. Therefore, qualitative assessment of RV function is still used in clinical practice. However, unlike the left ventricle, the tripartite morphology and different myofiber architecture of the right ventricle do not always lend themselves to clinically applicable qualitative assessment.
The RV myofiber architecture is composed of superficial circumferential and dominant deep longitudinal layers aligned base to apex that allow greater longitudinal than radial shortening and minimum twisting and rotational movements. The longitudinal shortening is the dominant deformation of the right ventricle that provides the major contribution to stroke volume during systole. Myocardial strain that describes this longitudinal deformation under an applied force is likely to provide a new sensitive measure of RV function in neonates. Two-dimensional (2D) speckle-tracking echocardiography (STE) is a relatively new angle-independent method for myocardial strain measurement that does not require geometric assumptions for its estimations. Myocardial strain measurements have been used to characterize RV function in adults but have not yet been applied to newborn and premature infants. The quantification of the uncertainties associated with speckle-tracking-based estimates of myocardial deformation, as well as the lack of “standardization” in acquisition and postacquisition processing with 2D STE, have introduced elements of variation and imprecision in STE-measured strain and precluded its clinical application in premature infants. Therefore, the objective of this study was to determine the clinical feasibility and reproducibility of 2D STE–measured global longitudinal strain imaging of the right ventricle in premature infants and establish methods for image acquisition and data analyses of strain measurements. This study was focused on developing a methodology that is likely to enhance the feasibility and reproducibility of RV peak global longitudinal strain (pGLS) and peak global longitudinal strain rate (pGLSr) measurements in premature infants. We did not evaluate the precision and accuracy of these measurements with respect to normal and pathologic mechanisms of physiologic adaptation in newborn infants.
Methods
Study Population
The factors that modulate variability in strain measurement by 2D STE are (1) variability in image acquisition, (2) intraobserver and interobserver variability in postacquisition processing, and (3) differences between echocardiographic equipment and proprietary software for image analysis. In this study, we addressed only the first two of these factors. The image acquisition and data-processing methods were developed using one commercially available ultrasound scanner (Vivid 9; GE Medical Systems, Milwaukee, WI) and one vendor-customized software workstation package (EchoPAC version 110.0.x; GE Vingmed Ultrasound AS, Horten, Norway).
To address the first two factors (variability in image acquisition and intraobserver and interobserver variability in postacquisition processing), we developed a cardiac strain imaging and postprocessing analysis protocol ( Appendix ) from a training phase cohort of 30 echocardiographic images in 30 premature infants (born at 28 ± 1 weeks) between August 2010 and August 2011. We then tested this protocol for variability in a separate study phase cohort with 100 images from 50 premature infants (born at 27 ± 1 weeks), obtained at two time points 4 weeks apart, between August 2011 and August 2012. To measure the reproducibility of myocardial strain indices, we selected a random sampling of 60% of the feasible images from the study phase cohort. We obtained informed consent from parents, and the institutional review board of Washington University approved the study.
Training Phase
Cardiac imaging acquisition “uncertainties” were identified in the training phase cohort to help develop a protocol. The standard apical four-chamber view used to acquire strain measurements for the left ventricle does not always include the entire RV free wall in its imaging plane and leads to poor speckle-tracking capabilities ( Video 1 ; available at www.onlinejase.com ). This uncertainty was addressed by acquiring RV-focused images. In this view, the right ventricle is centered in the imaging plane sector to define the full extent of RV free wall with no visible gaps. This RV-focused view avoids plane shifts that lead to the loss of the optimum number of RV segments throughout diastole and systole for tracking, particularly at the basal region. The uncertainty regarding optimal myocardial speckle brightness (defined by clear demarcation of the endocardial border and brightness of the myocardium in B-mode 2D optimum grayscales) was addressed by multiple adjustments in imaging parameters ( Appendix ). This view also minimized near-field artifacts and echo reverberations.
Semiautomated postprocessing strain data analysis uncertainties were identified and quantified in the protocol. Poor manual placement of the tracing of the endocardial border by an observer often resulted in inaccurate software-generated regions of interest (ROIs) and unreliable strain data. To ensure the efficiency of the software-generated ROIs and strain curves, we identified three specific apical and basal landmarks for the right ventricle to aid the observer in the manual placement of the endocardial tracing. A “sail sign” was manually traced from the (1) septal side of the tricuspid annular plane (septal-tricuspid annular hinge point) to (2) the apical-septal point and then to (3) the RV free wall side of the tricuspid annular plane (lateral-tricuspid annular hinge point). These three landmarks enhanced the software tracking of the RV myocardial tissue ( Figure 1 ). The ROI covered the endocardium and entire width of myocardium, excluding pericardium. The right ventricle was then divided into six standard segments (at the basal, middle, and apical levels on the septal and RV free wall sides), and the software generated six corresponding time-strain curves. The peak of the average curve of all the segments was considered the pGLS ( Figure 2 ).


Frame rate has been considered an important aspect of image acquisition. We demonstrated previously that reproducibility in children is most robust for images obtained with frame rates between 60 and 90 frames/sec. Because resting heart rates in premature infants are relatively high, we balanced between a relative low frame rate that affects the precise measurements of magnitude of strain and strain rate and a high frame rate that potentially would compromise spatial resolution because of sacrifices in line density. Thus, we kept the frame rate of image acquisition between 90 and 120 frames/sec in this cohort of premature infants ( Appendix ).
Study Phase
The 50 premature infants (born at 27 ± 1 weeks) in the study phase were prospectively enrolled from among infants participating in the Prematurity and Respiratory Outcomes Program, a seven-center initiative sponsored by the National Heart, Lung, and Blood Institute to identify physiologic and biochemical markers of adverse pulmonary outcome at 1 year of age ( ClinicalTrials.gov identifier NCT01435187 ). All infants in the study phase were enrolled in the Washington University/St. Louis Children’s Hospital neonatal intensive care unit between September 2011 and August 2012 ( Table 1 ). The inclusion criterion was premature birth (born between 23 weeks and 28 weeks 6 days gestational age). Infants with congenital heart disease (except patent ductus arteriosus and hemodynamically insignificant ventricular septal defects or atrial septal defects) or other congenital anomalies were excluded. Each premature infant underwent an echocardiographic evaluation at two time points 4 weeks apart ( n = 100 total imaging studies). The timing of the first echocardiographic study was carefully selected to occur at 32 weeks postmenstrual age (PMA) to avoid the early postnatal period of clinical and cardiopulmonary instability, frequent occurrence of patent ductus arteriosus, and early mortality associated with extreme preterm birth. Choosing to study all infants at a common PMA, rather than chronologic age, optimized the determination of the impact of gestational and chronologic age on cardiac structure and function at a specific developmental stage. The second echocardiographic study was performed 4 weeks later, at 36 weeks PMA.
Variable | Training phase | Study phase | P |
---|---|---|---|
Demographics | |||
Number of infants enrolled | 30 | 50 | |
Numbers of images acquired | 30 ∗ | 100 † | |
Gestational age at birth (wk) | 27 ± 1 | 27 ± 1 | .34 |
Weight at birth (kg) | 1.0 ± 0.2 | 0.96 ± 0.2 | .28 |
Weight at first echocardiographic study (kg) | 1.7 ± 0.4 | 1.42 ± 0.3 | .13 |
Weight at second echocardiographic study (kg) | NA | 2.21 ± 0.3 | |
Male/female | 15/15 | 16/34 | .15 |
Cardiovascular | |||
Patent ductus arteriosus | 4/30 | 10/48 ‡ | .18 |
Heart rate (beats/min) | 153 ± 18 | 164 ± 16 | .4 |
Systolic blood pressure (mm Hg) | 75 ± 11 | 76 ± 9 | .2 |
Diastolic blood pressure (mm Hg) | 44 ± 10 | 45 ± 8 | .14 |
Mean arterial pressure (mm Hg) | 54 ± 10 | 54 ± 7 | .19 |
Respiratory | |||
Respiratory rate (breaths/min) | 58 ± 12 | 49 ± 8 | .37 |
Pulse oximetry (%) | 96 ± 3 | 97 ± 2 | .29 |
Respiratory support | 18/30 | 32/48 § | .18 |
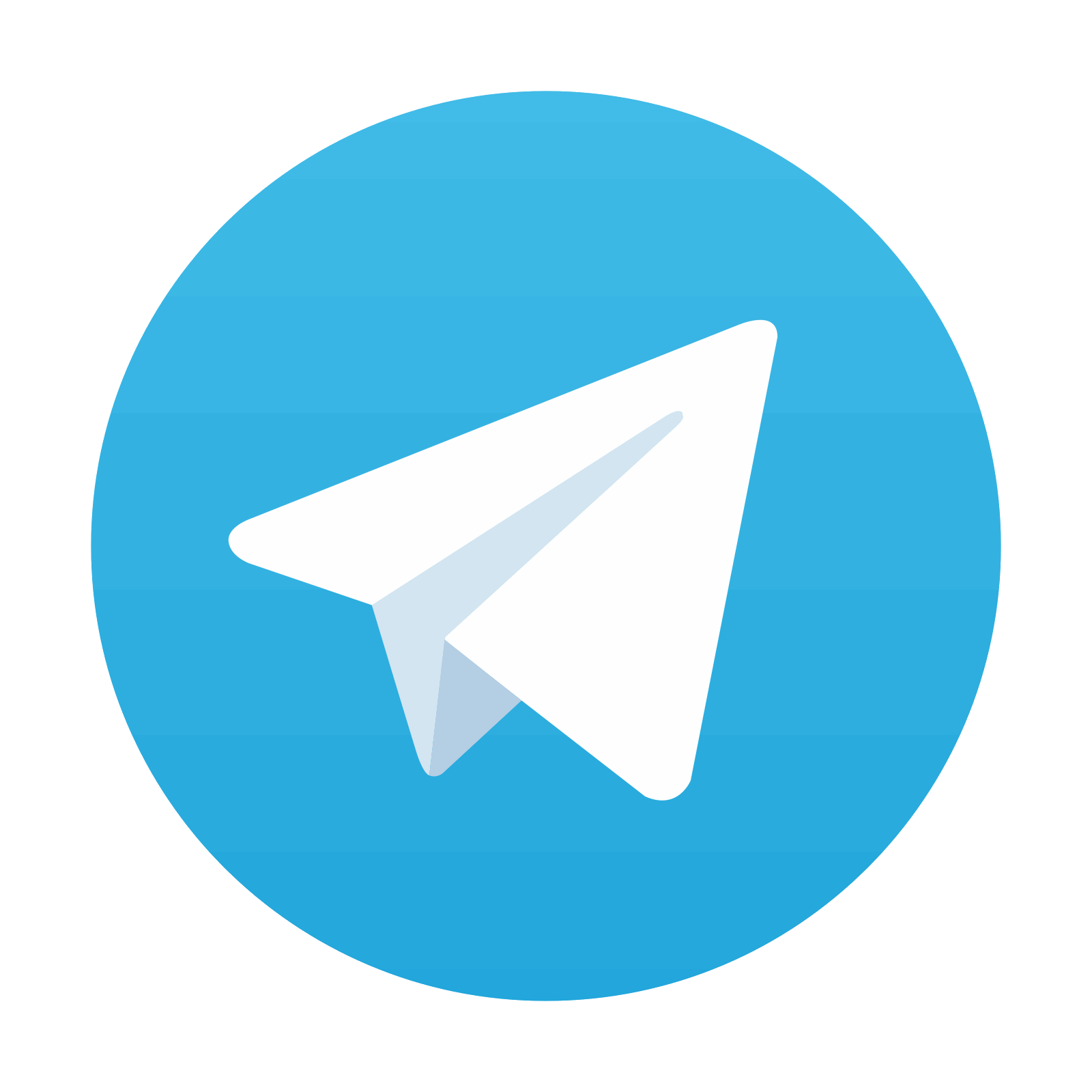
Stay updated, free articles. Join our Telegram channel

Full access? Get Clinical Tree
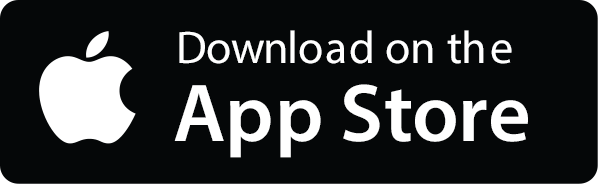
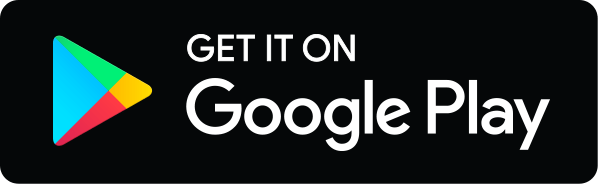
