Background
The significance of reduced right ventricular (RV) deformation reported in endurance athletes (EAs) is unclear, highlighting the ambiguities between physiologic RV remodeling and pathology. The aim of this study was to test the hypothesis that RV functional reserve would be normal in EAs despite reduced deformation measures at rest.
Methods
Forty EAs and 15 nonathletes (NAs) performed maximal incremental exercise with simultaneous echocardiographic measures of RV function. Two-dimensional (2D) and color-coded Doppler acquisitions were used to quantify peak systolic strain and strain rate (SRs) for the basal, mid, and apical RV free wall. A second surrogate of contractility, the RV end-systolic pressure-area relationship, was calculated from the tricuspid regurgitant velocity and the RV end-systolic area. Changes in multiple measures obtained throughout exercise were used to assess the affect of exercise on RV contractility.
Results
Compared with NAs at rest, basal RV strain and SRs were reduced in EAs, with good agreement between 2D and Doppler methods. During exercise, there was a strong linear correlation between heart rate and global SRs ( r = −0.74 and r = −0.84 for Doppler and 2D methods, respectively, P < .0001), which was similar for EAs and NAs ( P = .21 and P = .97 for differences in mean regression slopes by Doppler and 2D echocardiography, respectively). Exercise-induced increases in the RV end-systolic pressure-area relationship were also similar for EAs and NAs ( P = .42). There was a strong correlation between RV global SRs and the RV end-systolic pressure-area relationship during exercise ( r = 0.71, P < .0001).
Conclusions
Comparable RV contractile reserve for EAs and NAs suggests that the lower resting values of RV in EAs may represent physiologic changes rather than subclinical myocardial damage.
Endurance athletic training results in remodeling of both the left and right ventricles. Remodeling may be structural, electrical, and/or functional. Some studies that have assessed right ventricular (RV) function in healthy endurance athletes (EAs) have found measures of myocardial function that are lower than those seen in nonathletes (NAs) and have concluded that RV function may be reduced. They have raised the possibility that this finding reflects myocardial pathology. Given that resting measures may, therefore, be confused with RV pathology, we hypothesized that exercise measures may prove a more instructive means of identifying normal RV function in EAs.
The need for improved characterization of RV function in EAs results from the overlay between the athlete’s heart phenotype and that of arrhythmogenic RV cardiomyopathy (ARVC). Both athlete’s heart and ARVC are associated with an increased prevalence of ventricular arrhythmias, and both conditions are associated with cardiac dilatation, which, in some athletes, may be relatively greater in the right ventricle. Furthermore, Heidbuchel et al. reported a high prevalence of electrophysiologic, structural, and functional abnormalities of the right ventricle in a cohort of athletes with complex ventricular tachycardia, making it virtually indistinguishable from ARVC except for the lower rate of heritability and genetic mutations. Thus, in athletes with cardiac enlargement and frequent ventricular ectopy, a significant diagnostic dilemma may exist that current imaging techniques do not resolve. There have been a number of attempts to find RV functional measures that may provide greater distinction between pathology and normal physiologic adaptation to endurance sports. Teske et al. reported that RV strain and strain rate were lower in healthy athletes, particularly among those who were most trained and had the greatest RV dilation, and we have reported that RV ejection fractions determined by magnetic resonance imaging were lower in EAs relative to NAs. Thus, resting measures do little to resolve ambiguity when assessing the right ventricle in athletes.
The contribution of RV function to overall cardiac performance increases with exercise. At rest, the work requirement of the right ventricle is minimal because of the very low RV afterload. However, with increasing exercise intensity, RV systolic pressures increase progressively, and this translates to increases in wall stress, coronary perfusion, and oxygen extraction that are relatively greater for the right ventricle than the left ventricle. Thus, it would seem that it is far more relevant to study RV function during exercise, when its work requirements are greatest and when any functional limitation is likely to be most apparent. However, although the idea of studying RV function during exercise is attractive, there are very few studies in which it has been assessed, either in normal subjects or in pathology. One of the major constraints is that the marked change in ventricular load confounds interpretation of all echocardiographic estimates of underlying contractility. Strain rate is potentially less load dependent than traditional RV measures, while another candidate surrogate of contractility is the end-systolic pressure-volume relationship. These measures have been assessed during exercise for the left ventricle, but as far as we are aware, there are only two studies in which the right ventricle was assessed by either method during exercise.
We hypothesized that healthy athletes would demonstrate normal RV functional reserve, even if resting measures of RV function were low. We assessed RV systolic contractility during exercise by means of strain rate (both Doppler and two-dimensional [2D] methods) and by assessment of the end-systolic pressure-area relationship (ESPAR).
Methods
The first 40 EAs (36 men, four women) aged 18 to 60 years who responded to advertisements at local sporting clubs and who were performing >10 hours of intensive exercise training per week in active training for an endurance sporting event (marathon, endurance cycling, or triathlon) were recruited. Fifteen NAs (13 men, two women) were recruited from advertisements among hospital staff members and were included if they were engaged in regular recreational activity (mild to moderate noncompetitive exercise for <3 hours/week). None of the respondents met the exclusion criteria of known cardiovascular disease, symptoms, or risk factors. One subject was subsequently found to have bicuspid aortic valve disease without hemodynamic consequence and was not excluded. Written informed consent was obtained, and the protocol was approved by the St. Vincent’s Hospital Human Research Ethics Committee.
A maximal cardiopulmonary exercise test (upright cycle ergometer, ER900 and Oxycon Alpha; Jaeger, Würzburg, Germany) was performed to characterize exercise conditioning in these groups. Within the same week, real-time echocardiography was performed on a semisupine cycle ergometer (Lode, Groningen, The Netherlands) with simultaneous 12-lead electrocardiographic recording (Marquette; GE Healthcare, Milwaukee, WI). At rest, left ventricular (LV) interventricular septal thickness, posterior wall thickness, and end-diastolic diameter were measured from M-mode images acquired from a parasternal long-axis view. Atrial areas were analyzed from 2D four-chamber apical images, and LV volumes were determined using Simpson’s biplane method.
Exercise was performed with an incremental increase in workload of 25 W every 2 min, and during each increment of exercise, the following images were acquired using a Vivid 7 Dimension echocardiograph (GE Vingmed Ultrasound AS, Horten, Norway) and stored for offline analysis (EchoPAC version 108; GE Vingmed Ultrasound AS).
RV area was obtained by endocardial tracings from 2D images acquired from a focused four-chamber apical view (see Figure 1 a). The RV areas at end-diastole and at end-systole enabled RV fractional area change to be determined as (RV area at end-diastole − RV area at end-systole)/RV area at end-diastole. RV imaging was also performed with colloid contrast to enhance endocardial border definition as required.

Systolic pulmonary artery pressure was calculated from maximal tricuspid regurgitant velocities with colloid contrast enhancement as validated previously at rest and during exercise, without the addition of right atrial pressure estimates (see Figure 1 b). We have previously reported good interobserver variability for echocardiographic estimates of systolic pulmonary artery pressure during exercise.
The RV ESPAR was calculated as for the left ventricle, except that area was substituted as a surrogate of volume. That is, RV ESPAR (mm Hg/cm 2 ) = systolic pulmonary artery pressure (mm Hg)/RV area at end-systole (cm ). The end-systolic pressure-volume relationship is an established measure of RV contractility. Under resting conditions in this same cohort, we validated 2D RV areas acquired by echocardiography with RV volumes derived by cine magnetic resonance imaging (see supplementary content ). The interclass correlation coefficient of r = 0.77 for end-systolic volumes and areas would suggest that RV area may serve as a reasonable approximate of volumes in the absence of a means of noninvasively determining RV volume during exercise. Although the values for area and volume will differ, as will the ratio against pressure, the extent of change in this relationship with exercise may be expected to behave in a similar manner.
Strain and peak systolic strain rate (SRs) were analyzed using two methods. Color-coded tissue Doppler acquisitions were performed with the narrowest sector possible to include the right ventricle while maximizing temporal resolution (frame rate, 120–160 frames/sec). The velocity range was optimized to avoid aliasing during exercise, and care was taken to align the RV free wall with the line of insonation. For analysis, a 10 × 3 mm region of interest with an offset distance of 12 mm was placed in the base, mid, and apical segments of the RV free wall. The region of interest was manually tracked throughout the cardiac cycle to follow myocardial motion. Segments were excluded if there were reverberation artifacts, dropout, or deviation of insonation angle of >20%. The peak strain and peak systolic SRs for each segment were recorded as the average of three consecutive cycles. Global peak SRs was calculated as the mean of the three RV free wall segments and was not calculated if any of the segments could not be analyzed.
Two-dimensional “speckle tracking” was performed on narrow-sector grayscale images of the right ventricle from an apical four-chamber view with typical temporal resolution of 60 to 90 frames/sec. For analysis, the four-chamber algorithm was selected from the EchoPAC software, and the endocardial borders were defined as previously described. Tracking was visually inspected throughout systole and accepted even if diastolic tracking was suboptimal. Importantly, drift compensation was deselected to ensure that diastolic tracking inaccuracies did not influence systolic results. Global strain and global peak SRs were recorded if all free wall segments could be tracked.
During exercise, heart rate (HR) was used as a surrogate of exercise-induced increases in inotropic state, given the strong association between chronotropy and inotropy during exercise. Thus, in the absence of invasive measures of contractility, exercise increases in strain rate were related to exercise-induced increases in HR.
Statistical Analysis
Comparisons of Doppler and 2D derivations of strain and SRs were performed according to the methods of Bland and Altman, with interclass correlation coefficients also reported. EAs and NAs were compared using independent t tests after Levene’s test to ensure equal variance.
To compare the response to exercise between groups of subjects, a two-stage within-subject trend analysis was performed whereby a linear regression of repeated measures was performed on every subject after ensuring acceptable linearity by Poon’s analysis. For each measure and in each subject, there were at least three values from which to derive this linear regression. Slope and intercept values were then used for group comparisons (independent t tests), and the mean values are quoted for each relationship.
Variability in repeated measures of strain and strain rate was assessed on a sample of 55 acquisitions (15 resting and 40 exercise measures). After >3 months and blinded to the original results, all stages of analysis (starting from placement of the region of interest) were repeated by the original investigator (intraobserver variability) and by a second investigator (interobserver variability) using the same cine loops as those used for the original analysis. The mean value of the two observations ( x ) and the absolute value of the difference between observations ( e ) ± SD were determined. Reproducibility was assessed by the coefficient of variation, ( e / x )100%; the 95% limits of agreement, x ± 1.96 SDs; and Pearson’s correlation coefficient.
All values are expressed as mean ± SD unless otherwise stated, and P values < .05 were considered significant. Statistical analysis was performed using SPSS version 16.0 (SPSS, Inc., Chicago, IL).
Results
Age, gender, and body surface area were similar between EAs and NAs. The age range of the athletes (22–60 years) is typical of the demographic commonly involved in endurance sporting events and was similar to that of the NAs (28–57 years). As expected, HRs were lower and all measures of cardiac size were greater in EAs than NAs (see Table 1 ).
Variable | EAs ( n = 40) | NAs ( n = 15) | P |
---|---|---|---|
Age (y) | 37 ± 8 | 38 ± 6 | .61 |
Men | 90% | 87% | .47 |
Body mass index (kg/m 2 ) | 23.6 ± 1.9 | 25.8 ± 2.8 | .002 |
Body surface area (m 2 ) ∗ | 1.94 ± 0.16 | 1.94 ± 0.14 | .45 |
Weekly exercise (h) | 16.3 ± 5.1 | 1.7 ± 0.4 | <.0001 |
Resting HR (beats/min) | 52 ± 8 | 65 ± 8 | .001 |
Echocardiographic measures | |||
IVS (mm) | 11.0 ± 1.6 | 9.2 ± 1.0 | <.0001 |
LVPW (mm) | 10.9 ± 1.3 | 9.7 ± 1.1 | .003 |
LVDd (mm) | 56.0 ± 5.1 | 51.2 ± 4.3 | .003 |
LV EDV (mL) | 141 ± 24 | 93 ± 20 | <.0001 |
LV ESV (mL) | 60 ± 12 | 38 ± 9 | <.0001 |
LV EF (mL) | 57.2 ± 4.5 | 58.9 ± 5.1 | .23 |
RV diastolic area (cm 2 ) | 26.7 ± 4.2 | 20.4 ± 4.1 | <.0001 |
RV systolic area (cm 2 ) | 12.8 ± 2.2 | 9.8 ± 2.3 | <.0001 |
RV FAC (%) | 51.7 ± 6.0 | 52.2 ± 7.3 | .80 |
LA area (cm 2 ) | 23.1 ± 3.5 | 17.3 ± 3.0 | <.0001 |
RA area (cm 2 ) | 23.5 ± 4.6 | 17.0 ± 3.3 | <.0001 |
Exercise measures | |||
Maximum HR (beats/min) † | 161 ± 13 | 160 ± 13 | .89 |
Watts performed (W) | 284 ± 34 | 190 ± 30 | <.001 |
Exercise duration (min) | 21.7 ± 2.8 | 14.2 ± 2.2 | <.001 |
V o 2 max (% predicted) ∗ | 146 ± 18 | 92 ± 17 | <.001 |
∗ Predicted from age-matched and sex-matched nomograms.
† HR at maximal exertion in semisupine exercise echocardiographic test (lower than for upright exercise).
Resting RV Deformation: Athletes Versus NAs
As demonstrated in Figure 2 , there was a reduction in basal RV free wall deformation in EAs compared with NAs that was consistent for SRs and strain and by both analytic techniques. There was also consistency between techniques in demonstrating an increase in apical RV strain in EAs relative to NAs. However, there was a clear difference in the measurement of RV apical SRs with augmentation in EAs relative to NAs by Doppler measures but not by 2D echocardiography. Overall, there were discrepant findings in global SRs between techniques due largely to this inconsistency in apical measures.
Exercise RV Deformation: Athletes Versus NAs
Using Doppler measures of deformation, the relationship between global RV SRs and HR during exercise was assessed using multiple linear regressions. A linear relationship could be defined for each subject (mean correlation coefficient, r = −0.74), which did not differ between NAs (mean regression equation, RV SRs = −0.021 × HR − 0.10) and EAs (RV SRs = −.018 × HR − 0.5) ( P = .21 for comparison in regression variables; see Figure 3 A). Using 2D methods, global RV SRs also increased with HR during exercise in a linear manner (mean regression coefficient, r = −0.84) and in an almost identical manner between groups (NAs, RVSRs = −0.0115 × HR − 0.69; EAs, RVSRs = −0.0114 × HR − 0.78; P = .97; Figure 3 B).
Regression analyses between SRs and HR were also performed for the basal, mid, and apical segments of the RV free wall ( Table 2 ). Using 2D methods, SRs increased with exercise similarly in EAs and NAs for all segments, whereas increases in the basal segment were greater in NAs than EAs using Doppler methods. During exercise, there appeared to be a greater difference in the pattern of segmental function according to methodology, with the base-to-apex gradient remaining evident by Doppler techniques, whereas it seemed to reverse (i.e., greater negative values at the base) using 2D techniques.
RV strain rate (sec −1 ) | Variable | Constant | ||||
---|---|---|---|---|---|---|
EAs | NAs | P | EAs | NAs | P | |
Base | ||||||
Doppler | 0.0059 | −0.0094 | .023 | −1.6 | −1.3 | .17 |
2D | −0.017 | −0.0088 | .11 | −0.94 | −0.27 | .13 |
Mid | ||||||
Doppler | −0.016 | −0.020 | .18 | −0.94 | −0.23 | .16 |
2D | −0.014 | −0.012 | .61 | −1.2 | −1.2 | .65 |
Apex | ||||||
Doppler | −0.019 | −0.010 | .11 | −0.64 | −1.1 | .42 |
2D | −0.011 | −0.0068 | .291 | −0.80 | −1.2 | .25 |
Comparison with RV ESPAR
There was a progressive increase in RV ESPAR with HR, and the mean regression variables obtained for NAs (ESPAR = −0.054 × HR + 1.5) and EAs (ESPAR = −0.060 × HR + 2.1) were similar ( P = .42). Comparing the two surrogate measures of contractility, there was a moderately strong correlation between RV ESPAR and RV global SRs by both 2D and Doppler methods ( r = 0.71, P < .0001, and r = 0.52, P < .0001, respectively; see Figure 4 ).
Comparison between Doppler and 2D Analysis of Strain and Strain Rate
Acceptable reproducibility of intraobserver and interobserver variability was demonstrated for SRs and strain, with less variability evident using the 2D methodology ( Table 3 ).
Variable | Intraobserver | Interobserver | ||||
---|---|---|---|---|---|---|
CV (%) | Correlation coefficient ( r ) | 95% limits of agreement | CV (%) | Correlation coefficient ( r ) | 95% limits of agreement | |
SRs (DTI) | 26.1 | 0.86 | 1.3–2.5 sec −1 | 33.0 | 0.76 | 0.8–3.0 sec −1 |
SRs (2D) | 19.0 | 0.88 | 1.1–2.3 sec −1 | 18.1 | 0.81 | 1.1–2.3 sec −1 |
Strain (DTI) | 20.8 | 0.90 | 18%–38% | 28.5 | 0.81 | 14%–42% |
Strain (2D) | 13.0 | 0.87 | 15%–31% | 12.8 | 0.88 | 16%–30% |
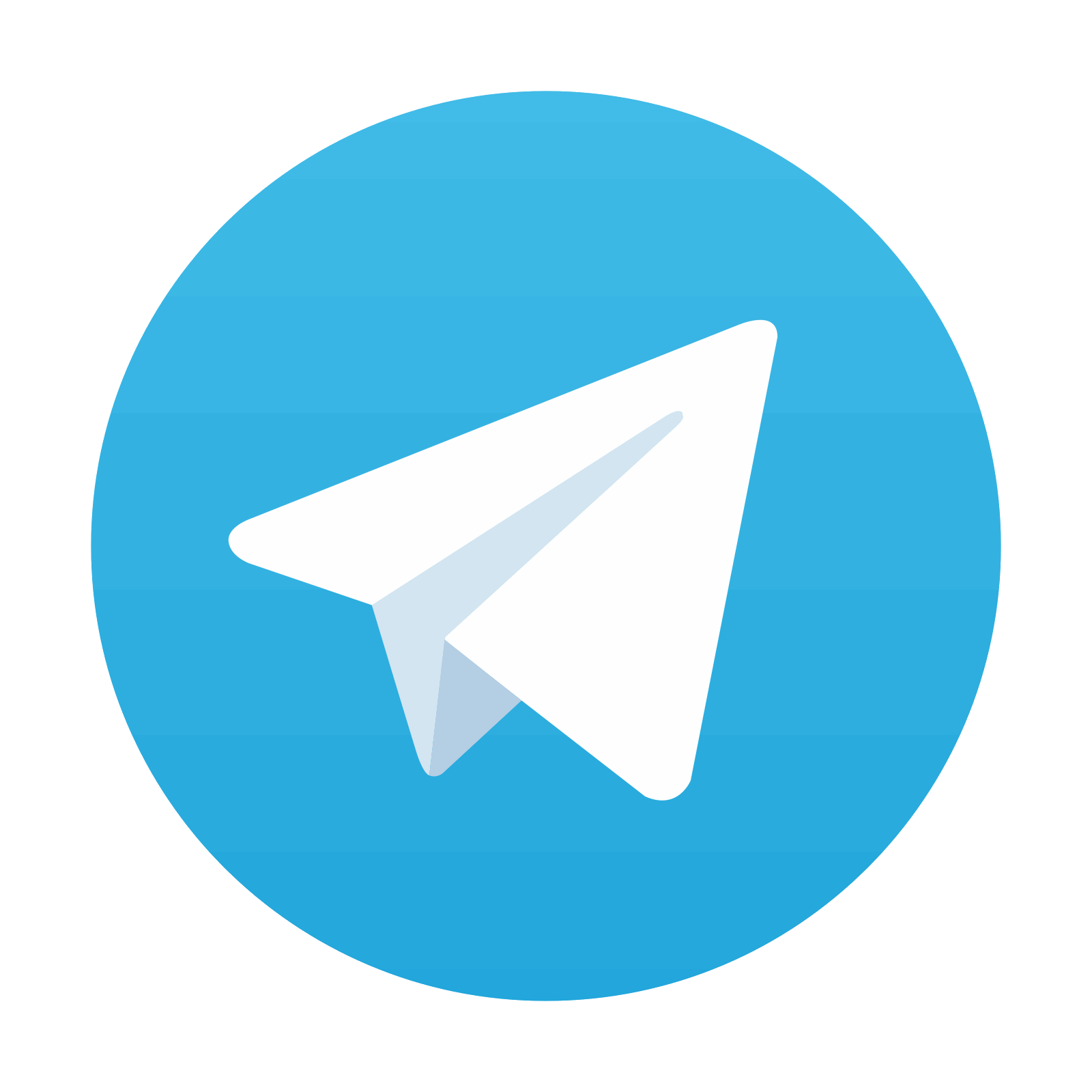
Stay updated, free articles. Join our Telegram channel

Full access? Get Clinical Tree
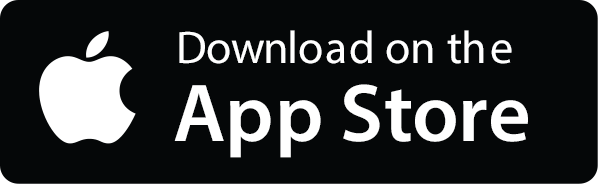
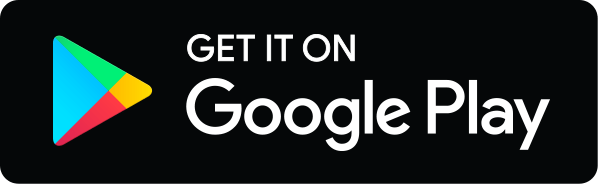
