Abstract
Exercise in children is not merely play; rather, it is a critical environmental and physiological perturbation that has a profound effect on growth and development in health and disease. In this chapter, we review fundamental knowledge focused on how the growing child adapts to the physiological stress of exercise with a particular focus on respiratory disease. Cardiopulmonary exercise testing (CPET) remains the most widely used approach for precise measurement of respiratory and cardiovascular fitness in children and adults. Essential diagnostic uses of CPET in asthma, cystic fibrosis, and lung disease of prematurity are reviewed. Children at high altitude, particularly those with chronic lung disease, are at risk for exercise impairment, and the mechanisms responsible for these challenges are identified. We present new information regarding an increasingly diagnosed syndrome that limits exercise performance in adolescents, exercise-induced laryngeal obstruction. Finally, we set the stage for the next phase of discovery in exercise research in children, namely linking exercise to genomic, epigenetic, proteomic, and metabolomic technologies that will, hopefully, advance our understanding of exercise as a biomarker and therapeutic adjunct in child health.
Keywords
cardiopulmonary exercise testing, peak oxygen uptake, habitual physical activity, high altitude, exercise challenge test, exercise-induced bronchoconstriction, exercise-induced laryngeal obstruction, omics
The Biological Importance of Physical Activity in the Growing Child
Exercise in children and adolescents is not merely play, but is an essential component of growth and development. Children are among the most spontaneously physically active human beings. It is not surprising that habitual physical activity (HPA) is a major determinant of health across the lifespan and health-related quality of life in both healthy children and in children with chronic diseases. Despite this essential biologic role for HPA, children have not been spared the relentless reduction in levels of physical activity (PA) that is creating a crisis in health care in our nation and throughout the world. Recognition of the enormous morbidity and cost of physical inactivity-related diseases, such as atherosclerosis, type 2 diabetes, and osteoporosis, along with the deleterious consequences of physical inactivity and deconditioning in pediatric respiratory diseases like asthma and cystic fibrosis (CF), has spurred new policy initiatives targeting preventive medicine early in life. The concept of pediatric origins of adult health and disease is gaining scientific merit (e.g., chronic obstructive pulmonary disease [COPD]), highlighting the need to transform existing notions of how to evaluate health in a growing child. A physically inactive child may have no symptoms of disease, but evidence of deterioration in vascular health may already be present. As we move into the era of population health management and precision medicine, the notion of what it means to be a healthy child must change and include robust metrics of physical fitness.
As noted, the deleterious health effects of physical inactivity and poor fitness are exacerbated in children with chronic disease and/or disabilities or with environmental-lifestyle conditions like obesity. Children with previously fatal diseases or conditions (e.g., CF, prematurity, acute lymphocytic leukemia) are living longer due to remarkable advances in research and care, but are often unable to achieve levels of PA and fitness associated with health benefits in otherwise healthy children. Not surprisingly, the healthspan (the period of life free from serious chronic diseases and disability ) of children with chronic diseases is threatened not only by the underlying disease but by the compounding effects of insufficient PA and sedentary behavior. Increasing PA and fitness is feasible, but has proven quite challenging to implement in a systematic manner. Once a pattern of physical inactivity and a sedentary lifestyle is established, a vicious cycle ensues ( Fig. 12.1 ), in which constraints on PA harm immediate health and contribute to lifelong health impairment ranging from cardiovascular and metabolic disease to osteoporosis. Exactly what constitutes ideal physical fitness in a child with a chronic condition remains unknown. Finding beneficial levels of PA in children with chronic disease or disability is challenging because the optimal range of exercise is much narrower than in a healthy child ( Fig. 12.2 ).


Change in policy and practice, and the resources necessary to achieve such change, must increasingly be fueled by translational scientific evidence. Despite the broad recognition that many children in the United States (and throughout the world) no longer engage in healthy levels of PA, defining what the level of optimal PA should be remains quite vague. For example, in a recent study of 182, 9–11-year-olds, Füssenich and coworkers noted, “there were no differences between CCVR [composite cardiovascular risk score] of children who undertook 60 min MVPA [moderate to vigorous physical activity] per day in accordance with WHO recommendations, and those who did not. This implies that current recommendations may be an underestimation of the PA [physical activity] necessary to reduce clustered CVD risk. A gender difference between the CVD risk in active and inactive children raises the possibility that gender specific guidelines may be needed, although much work is needed to determine if these differences are a result of gender specific responses to PA or sex differences in PA level…. Taken together these findings suggest that in order to reduce CVD risk, the current guidelines should be updated….”
From the Playground to the Bench to the Physician’s Office
Integrating Laboratory Cardiopulmonary Exercise Testing and Field Assessments of Physical Fitness
Although it might seem intuitive that physically active children and adolescents score well in cardiopulmonary exercise testing (CPET), quantifying the mechanistic relationship between these two components of physical fitness and activity in children and adolescents has proven to be challenging. Only weak correlations between HPA and CPET are consistently found ; HPA and CPET are not interchangeable physical fitness–associated biomarkers. Traditional CPET focuses on measuring maximal efforts, but as we and others have shown, high-intensity PA occurs relatively infrequently in real lives of children and adolescents ; thus, even high levels of HPA may not be evident in traditional CPET. Until recently, there has been little standardization of the methodologies used to assess HPA in children, which include a myriad of tools and technologies such as questionnaires, activity logs, HR monitoring, and wearable accelerometers. Most practicing primary care child health care providers perceive the overall levels of and participation in daily HPA as a more important indicator of child health than the results of CPET per se (if only because formal education in exercise physiology and CPET is lacking in medical school or most residency programs).
Schools and communities are currently investing substantial resources in physical fitness assessments in children; such tests are mandated in 46 of 50 states in the fifth, seventh, and ninth grades. In California, testing is done “to help students in starting life-long habits of regular physical activity.” The school-based tests typically used are the 20 m shuttle run or the mile run. The raw data from these tests are entered into standardized equations to estimate maximal oxygen uptake (V̇O 2max ), but none of the data become part of the child’s medical record.
What Can We Learn From Cardiopulmonary Exercise Testing ( Fig. 12.3 )
Consider, for example, the important acts of fleeing from a predator or, in more modern terms, running to avoid an oncoming car. When sudden and large increases in metabolic demand are imposed by PA, the whole organism can successfully function only by means of an integrated response among several organ systems. At the very onset of exercise, before there has been sufficient time for an in increase in environmental oxygen uptake, the healthy human must have sufficient stores of oxygen, high-energy phosphates, nonaerobic metabolic capability, and supplies of substrate to perform significant amounts of PA. As exercise proceeds, cardiac output increases and blood flow is diverted to the working muscles without compromising the critical flow of oxygen and glucose to the brain.

Ventilation and pulmonary blood flow must increase to precisely match the energy demand of the working muscles so that homeostasis for PaCO 2 and pH are maintained. There must be sufficient increase in substrate availability (glucose, fat, protein) but without depleting the peripheral blood glucose stores. And finally, the heat produced during exercise must be dissipated so that homeostasis for body temperature is maintained. In summary, as diagrammed in the late 1960s by Wasserman and coworkers, events at the cell are closely linked to events at the heart and lungs ( Fig. 12.4 ).

Physical fitness and exercise biomarkers are promising “disease-agnostic” tools for a remarkably wide range of health conditions, ranging from asthma in children to COPD in the elderly. Exercise testing provides insight into integrated physiological responses that are hidden when the subject is at rest. Despite this promise, exercise-related outcome variables are used far more commonly in the research laboratory than in clinical trials or clinical practice. Poor fitness and low PA are major causes of morbidity and mortality from obesity, cardiovascular disease, type 2 diabetes, chronic respiratory diseases, and some malignancies, but the use of exercise biomarkers in clinical trials is still largely untapped. Consequently, the potential tangible clinical benefits of exercise as a diagnostic and therapeutic tool have failed to fully materialize.
Children are naturally physically active, and therefore quantifying physical fitness through formal exercise testing is increasingly useful as a biomarker in a variety of pediatric conditions such as congenital heart disease, sickle cell anemia, athletic training, and cerebral palsy. Physiological parameters obtained at maximal or peak exercise (most notably, maximal oxygen uptake-V̇O 2max or peak V̇O 2 ) remain the most widely used type of exercise laboratory test in pediatric clinical trials and research. As outlined below, true plateaus or reductions in oxygen uptake despite increasing work rate (WR), the hallmark of V̇O 2max , occur only in a minority of children. CPET is minimally invasive, rendering it suitable for studies in children. CPET is typically performed with cycle ergometers or treadmills, in which the work input increases progressively until the child reaches the limit of his or her tolerance.
Maximal exercise tests are, by definition, highly dependent on the willingness of each child to continue exercise at relatively high WRs when dyspnea, muscle fatigue, and other stress sensations are commonly experienced. Not surprisingly, the “cheerleading” abilities of the laboratory personnel contribute to the achievement of a true maximal response in CPET. Despite its proven clinical and research utility, V̇O 2max , as noted, only occurs in a minority of exercise tests even in otherwise healthy children and maybe less so in children with chronic disease or disability. For example, in a recent large study of children and adolescents (mean age 12.3 years old) who had undergone the Fontan correction for congenital heart disease during childhood, only 166 of 411 patients (40%) achieved an acceptable V̇O 2max using current criteria. This is one reason why many clinicians and researchers choose to use the “peak” V̇O 2 rather than V̇O 2max .
Most modern commercially available CPET devices measure gas exchange variables (V̇O 2 , carbon dioxide output-V̇CO 2 , ventilation-V̇ E ) and heart rate (HR) quite frequently (e.g., breath-by-breath or with mixing chamber gas collection devices in regular intervals multiple times per minute), and these data are collected well before maximal exercise is achieved. Thus, when a child does not reach an exercise level in CPET that can be classified as maximal, the whole test may be deemed a failure despite the wealth of data successfully collected. Such data could shed light on fitness and other specific indicators of cardiac, pulmonary, or metabolic disease even in the absence of a true V̇O 2max .
Physiologic Response to Progressive Exercise Testing
A ramp protocol is often used in CPET in adults and children, and is so named because exercise is performed on a cycle ergometer and WR increases linearly in a ramp-type pattern until the participant reaches the limit of his or her tolerance ( Fig. 12.5 ). Gas exchange is typically measured with the use of mouthpieces or facemasks and the many technological advances made over the past several decades in assessing oxygen uptake and carbon dioxide output permit breath-by-breath measurement. HR is measured using standardized monitoring. Armstrong and Fawkner summarized noninvasive methods that are currently used to assess exercise biomarkers in children. It has been particularly useful in developing relatively simple equations to describe the gas exchange and HR responses to the progressive WR exercise protocols that typify CPET. Whipp and coworkers elegantly outlined the theoretical and experimental framework that predicted and demonstrated the largely linear response of V̇O 2 to the steady increase in WR during ramp exercise protocols. Their model assumed a system with first-order linear dynamics, an assumption that is accurate for low-intensity exercise. A number of studies have attempted to determine the value of τ (the time constant for the first-order linear equation) for gas exchange and HR variables in children. Factors such as maturational status and the presence of disease and obesity appear to play a role. After the initial minutes of the ramp test, V̇O 2 approaches a linear increase as the ramp progresses. We do recognize that system dynamics become more complex when exercise is performed at high intensities.

The anaerobic threshold (AT, also referred to as the “lactate threshold”) indicates the point at which excess lactic acid production leads to increases in circulating lactate concentrations and acidosis. Although the mechanism of the AT is not completely clear, one possibility is that at some point during exercise, oxygen supply to the working muscles is inadequate and anaerobic metabolism is necessary to supply the energy requirements of physical work. The AT can be measured directly from blood samples taken during exercise. The onset of anaerobic metabolism is affected by such factors as anemia and the presence of peripheral vascular disease. The ramp protocol and breath-by-breath analysis of gas exchange allow a noninvasive measurement of the ventilatory threshold (a synonym for AT when gas exchange data is used) and V̇O 2max in a single exercise test. Measuring the AT in children is a potential biomarker of both respiratory and cardiovascular disease, first, because the test is noninvasive, and second, because the protocol is both brief and stimulating to the young child whose sense of competition is high but whose attention span is low. Additional work is needed to examine the maturation of the kinetics of CPET variables during growth and maturation.
The dynamic relationships among gas exchange and HR variables during progressive CPET are promising tools for evaluating fitness in healthy children or in children with chronic disease and disability. The relationship between V̇ E and V̇CO 2 is given by a modification of the alveolar gas equation:
V ˙ E = [ 863 × PaCO 2 − 1 × ( 1 − V D / V T ) − 1 ] × V ˙ CO 2
Because ventilation and CO 2 production during exercise are determined in large measure by muscle mass (highly related to body mass), ΔV̇ E /ΔV̇CO 2 should be a body-size–independent CPET variable. However, others and we have noted a weak but significant correlation between ΔV̇ E /ΔV̇CO 2 and both total body mass (TBM) and lean body mass (LBM). As has been described in greater detail earlier, analysis of the alveolar gas equation indicates that ΔV̇ E /ΔV̇CO 2 is determined by ventilatory factors such as physiological deadspace, CO 2 storage capacity, and the PaCO 2 set point (the concentration of CO 2 that is homeostatically maintained despite changes in CO 2 production). Maturation of each of these factors is reflected by the higher values of the slope in younger children compared with adolescents.
ΔV̇ E /ΔV̇CO 2 is abnormal in children with chronic lung diseases like CF, in which ventilatory deadspace is known to be increased, and was recently found to be an independent predictor of mortality in adult patients with chronic obstructive pulmonary disease undergoing surgery for nonsmall-cell lung cancer. Thus, analysis of ΔV̇ E /ΔV̇CO 2 in children with chronic lung diseases may provide specific information about lung function not readily determined from maximal exercise values alone.
The Challenge of Scaling Cardiopulmonary Exercise Testing to Body Mass in Children and Adolescents and the Special Case of Obesity
Physical fitness and PA are essential components in both the diagnosis and treatment of the overweight and obese child and adolescent. Despite this, evidence-based guidelines do not yet exist on how best to assess and track physical fitness in this population. Recently, Hansen and coworkers reviewed a number of studies focused on assessing fitness from CPET in obese adolescents. They were unable to find consensus among these studies, and reached the following conclusion: “Whether cardiopulmonary anomalies during maximal exercise testing would occur in obese adolescents remains uncertain. Studies are therefore warranted to examine the cardiopulmonary response during maximal exercise testing in obese adolescents.”
Measuring fitness in children (whether normal weight, overweight, or obese) is complicated because muscle and fat mass and hormonal regulation of metabolism and growth change rapidly in children and adolescents. Consequently, any physiological variable derived from CPET must be scaled to some index of body size and maturational status. In the obese child, useful scaling of CPET variables is further confounded because body fat (virtually metabolically inactive during exercise) may obscure the effect of the metabolically active muscle tissue when CPET is normalized to body mass.
As noted, maximal exercise tests are highly dependent on the willingness of each child to continue exercise at relatively high WRs. In some cases, investigators purposefully do not exhort obese children during progressive exercise testing. For example, Salvadego and coworkers studied exercise in a group of obese, otherwise healthy adolescents and stopped exercise when the participant achieved a HR of 180 bpm. The authors noted, “A true maximal test was not performed to avoid the cardiovascular risks associated with maximal exercise in obese subjects.” Further, several studies suggest that obese children and adolescents perceive high-intensity exercise differently than normal-weight controls. It is not surprising that the plateau in oxygen uptake, the classical physiologic proof that V̇O 2max had been reached, is found in relatively small proportions of normal-weight or obese children and adolescents.
The data from our earlier study of CPET slopes in children and adolescents with body mass index (BMI) below the 85th percentile showed that many of these variables, like peak or maximal V̇O 2 , were highly correlated with muscle mass. We recently found that both CPET submaximal values and peak V̇O 2 were lower in high-BMI adolescents (BMI >95th percentile). We observed this deficiency even after referencing CPET values to LBM. The mechanisms for reduced both peak and submaximal CPET results are not entirely clear. Obese children may not push themselves as hard as normal-weight children in the high-intensity range of exercise that typifies peak or maximal V̇O 2 . Shim and coworkers in obese children with asthma and Marinov and coworkers in otherwise healthy obese children, for example, noted greater sense of breathlessness at high WRs than in normal-BMI children. Salvadego and coworkers showed in a recent elegant study that obese adolescents improved their rate of perceived exertion during high-intensity exercise by reducing the work of breathing (using Heliox).
Development of Physiological Responses to Exercise in Children and Adolescents and Predicting Cardiopulmonary Health Across the Lifespan
With respect to exercise and PA, children are not simply miniature adults. When normalized to body size, strength is lower in children, as is the magnitude of the physiologic response to chronic exercise training (both resistance and aerobic). Children use relatively more oxygen than adults for high-intensity exercise. Gas exchange and HR response kinetics are also different in children ( Fig. 12.6 ), as are metabolic responses such as lactate concentrations, high-energy intramuscular phosphate dynamics (using 31 P-MR spectroscopy ), and CO 2 storage capacity. Recent work on the genomic responses to exercise in children shows differences in the pattern of leukocyte gene expression between early- and late-pubertal girls. The mechanisms that link the distinct processes of growth and adaptation to exercise are largely unknown.

A number of pioneering, thoughtfully designed, long-term studies now confirm that cardiovascular disease risk factors begin in youth, track into symptomatic atherosclerosis in adulthood, and are, fortunately, modifiable (e.g., the Muscatine Study, the Young Finns Study, the Bogalusa Heart Study, the CARDIA study, and Pathobiological Determinants of Atherosclerosis in Youth [PDAY] ). Efforts to develop childhood- and youth-based preventive interventions focused on diet and PA, in combination or separately, have not met expectations. Similar work is needed to determine the long-term effects of pediatric lung disease and the role that exercise and fitness may have in the trajectory of respiratory health across the lifespan.
Searching for Normal Values in Pediatric Cardiopulmonary Exercise Testing
Resources are needed and in many cases have been mobilized to support the development of drugs and devices focused specifically on children. Clinical investigators are increasingly aware of regulatory incentives to perform clinical trials on drugs used specifically for childhood diseases, rather than relying on adult studies. Moreover, clinical researchers understand that effective clinical trials focused on childhood disease must be accompanied by improved laboratory reference standards developed for children.
In a comprehensive review, Shaw and coworkers outlined the challenges facing the development of laboratory reference values in pediatrics. Although focused primarily on blood biomarkers, the following summary provides a relevant framework for establishing normative values in pediatric CPET:
Reliable and accurate reference intervals for laboratory analyses are integral for correct interpretation of clinical laboratory test results and therefore for appropriate clinical decision making. Ideally, reference intervals should be established based on a healthy population and stratified for key covariates including age, gender, and ethnicity. However, establishing reference intervals can be challenging as it requires the collection of large numbers of samples from healthy individuals. This challenge is further augmented in pediatrics, where dynamic changes due to child growth and development markedly affect circulating levels of disease biomarkers.
Mounting literature suggests that fitness (assessed as peak V̇O 2 ) can help predict morbidity and mortality in children. Therefore the development of robust reference values for CPET will serve as a powerful tool in the clinician’s ability to (1) identify children in whom the “exercise prescription” might be most beneficial, and (2) assess the success of exercise interventions across the child health spectrum. Of equal importance is the larger public health question of the degree to which fitness and PA levels are decreasing in children and adolescents in the United States and throughout the world and the extent to which these serious threats to health across the lifespan are related to environmental, socioeconomic, and genetic/epigenetic factors. Absent the development of robust CPET normal values, we cannot answer these critically important questions. However, reference values that may be helpful for CPET in children are available.
Asthma and Exercise
The Role of Exercise and Physical Activity in Asthma
Despite much recent progress in understanding asthma pathophysiology and the development of new therapies, the health care use associated with asthma and the disruptions it causes to family and community life have not decreased substantially. From the 1990s to the 2000s, asthma prevalence was reported to plateau or even decrease in high-income countries while, in many cases, asthma symptoms became more common. At the same time, many low- to middle-income countries with large populations showed increases in prevalence, suggesting that the overall world burden is increasing and nonexpensive strategies are needed to cope with the challenge. Similar to patterns observed in asthma prevalence, children experience higher rates of incident asthma than adults and younger children have higher rates as compared with older children.
The link between PA and asthma is strong, but remains enigmatic. PA is a “double-edged sword” for the child with asthma. On the one hand, PA and exercise is a common trigger of bronchoconstriction, occurring in as many as 90% of asthmatic children and 45% of children with allergic rhinitis. Exercise-induced bronchospasm inhibits the ability of affected children to participate normally and perform optimally in physical activities, and it is recognized as an indicator of risk for urgent medical visits. On the other hand, exercise and fitness training seem to benefit asthma control and severity in many children with asthma. As succinctly stated by Lucas and Platts-Mills, “It is our belief that an exercise prescription should be part of the treatment for all cases of asthma. The real question is whether prolonged physical activity and, in particular, outdoor play of children play a role in prophylaxis against persistent wheezing. If so, the decrease in physical activity might have played a major role in recent increases in asthma prevalence and severity. The move from traditional lifestyle to urban living can be seen as the progressive loss of a lung-specific protective effect against asthma.”
Critical exercise-asthma treatment issues remain enigmatic and poorly studied, ranging from rare but tragic instances of death due to exercise-induced bronchoconstriction (EIB) in asthmatic youth to the lack of clinically validated paradigms of “return to play” following an exercise-associated asthma attack. Despite the accepted clinical goal of ensuring that children with asthma fully participate in all types of exercise, physical fitness and participation in PA have been shown to be impaired in children with asthma. Participation in school PE among children with asthma is reported to be reduced by as much as 40%, and only 1% of adolescents with asthma and 6% of their teachers gave the correct answer on how to prevent exercise-induced asthma, emphasizing the challenge. Children with asthma were less fit (10% lower V̇O 2max ) than their peers. Moreover, Conn and coworkers discovered excessive use of electronic media in children with asthma, particularly in those with activity limitation. Studies using accelerometers (a device worn on the body that can measure movement) show equivocal results, and Lovstrom and colleagues found that asthmatic patients (10–34 years old) were more frequently physically active and for longer durations than controls. These latter reports can add to the changing prevalence in asthma in recent years. For the inactive asthmatic children, whether this is a result from the perception of disability, parental restrictions, or from poorly managed exercise-associated symptoms is not known. Nevertheless, whatever the causes, reduced participation in PA is an ominous finding in a child with asthma.
There are emerging data, which suggest that exercise is beneficial for asthma in terms of disease control and pathogenesis. A growing number of animal studies (such as those by Pastva and coworkers and Hewitt and coworkers ) examined how brief exercise and exercise-training modulated subsequent lung inflammatory responses to ovalbumin (OVA) challenge in OVA-sensitized rats. An intriguing finding from Kodesh and coworkers showed that rats sensitized to OVA were subsequently more susceptible to bronchoconstriction following exercise, a nonspecific challenge. These studies demonstrated a generally moderating effect of exercise on subsequent lung inflammatory responses to acute allergen and exercise challenges, specifically by decreasing nuclear factor κB (NF-κΒ) nuclear translocation and IκΒ-α phosphorylation, thereby diminishing key proinflammatory (and possible neuroadrenergic) control pathways. Aerobic training reduced leukocyte activation, reversed airway remodeling, and airway inflammation. Specifically it reversed OVA-induced eosinophil and macrophage airway migration and decreased expression of Th2 cytokines (IL-4, IL-5, IL-13), eotaxin, RANTES, chemokines (CCL5, CCL10), adhesion molecules (VCAM-1, ICAM-1), reactive oxygen and nitrogen species (GP91phox and 3-nitrotyrosine), inducible nitric oxide synthase (iNOS), and NF-κB. It also increased the expression of the antiinflammatory cytokine (IL-10). Repeated bouts of moderate-intensity aerobic exercise improve airway hyperresponsiveness (AHR) in OVA-treated mice via a mechanism that involves β 2 -adrenergic receptors.
In children, studies of the benefits of PA and exercise training have yielded similar results. Lung function in children who are more physically active tend to be higher. Aerobic training programs (8–16 weeks) were shown to improve disease control (Asthma Control Questionnaire) and severity (daily doses of inhaled steroids), exercise capacity (V̇O 2max ), reduce pulmonary inflammation (exhaled NO), decrease bronchial hyperresponsiveness (exercise challenge test/methacholine challenge test), and medication use. Lung function (FEV 1 , FVC) does not change after aerobic training sessions.
Most training sessions include aerobic exercise, and the effect of resistance training on the asthmatic child is yet to be evaluated. Bonsignore and coworkers concluded that exercise training in combination with antiinflammatory therapy might synergize to attenuate airway response to methacholine challenge in asthmatic children. Furthermore, physical inactivity and increased sedentary time were found to predict childhood asthma directly and indirectly (obesity induced). Uncontrolled asthma is associated with reduced fitness and daytime spent in intensive activity. Poorly controlled asthma in children contributes to lung disease in adulthood ; thus efforts to improve fitness and asthma control in children and adolescents, a “critical period” of growth and development, are bound to have effects on health that last a lifetime. As noted by Ploeger and coworkers, “To optimize exercise prescriptions and recommendations for patients with a chronic inflammatory disease, more research is needed to define the nature of physical activity that confers health benefits without exacerbating underlying inflammatory stress associated with disease pathology.”
Exercise-Induced Bronchoconstriction
Exercise-related respiratory symptoms are underdiagnosed and undertreated in many cases and may reflect an isolated clinical condition or uncontrolled underlying asthma. EIB is the acute transient airway narrowing during or after exercise. EIB is defined by the American Thoracic Society (ATS) as percent fall in FEV 1 from the preexercise level of >10%–15%, with responses graded as mild, moderate, or severe (10%–25%, 25%–50%, and >50%, respectively). This grading was based on measured values before the widespread use of inhaled steroids; and currently when a child performs an exercise challenge test, FEV 1 fall of ≥30% can be graded as “severe.” The upper limit of postexercise fall in FEV 1 (mean ± 2 SD) in normal children was found to be 6%–8% and higher values for percent fall in FEV 1 (13%–15%) have been recommended for diagnosing EIB. Protocols developed by a pioneer in the field, Simon Godfrey, suggest a threshold of FEV 1 of ≥13%. This threshold was recommended also for preschool children. Other laboratories use a threshold of FEV 1 fall of >15% from baseline when testing children to increase sensitivity of the test. Fonseca-Guedes found that FEF25%–75% (postexercise fall >26%) may add to the sensitivity of the test, mainly in children with mild asthma. Symptoms of EIB typically begin after 5–10 minutes of vigorous exercise, peak a few minutes after stopping physical exertion, and usually last for 30–90 minutes. Symptoms may include shortness of breath, dyspnea, chest tightness, coughing, wheezing, decreased performance, increased fatigue, chest pain, and chest tightness. In children, the exertional symptoms can be less profound and may include a combination of symptoms.
Obese asthmatic children have a greater exercise-induced fall in FEV 1 and a slower recovery from EIB than nonobese asthmatic children, which can limit their participation in physical and sporting activities with peers. Dietary-induced weight loss in overweight and obese asthmatic children leads to significant reduction in severity of EIB and improvement of the quality of life.
Exercise Challenge Test
An exercise challenge test is usually performed on a treadmill or a cycle ergometer. Most children are more used to walking or running than to cycling, and exercise complaints often appear during running (such as when playing tag, dodge ball, or soccer) ( Fig. 12.7 ). Therefore running is the preferred exercise in children, and can be standardized using a treadmill. ATS and ERS guidelines for EIB testing involves a rapid increase in exercise intensity over approximately 2–4 minutes to achieve a high level of ventilation while breathing dry air (<10 mg H 2 O/L). It should be noted that in adults, breathing dry air enhances EIB and is associated with circulating biomarkers of epithelial damage. The test is performed with a nose clip in place while running or cycling at a load sufficient to raise the HR to ~90% of predicted maximum (predicted maximum HR = 220-age in years) or ventilation to reach ≥17.5–21 times FEV 1 (the two most important determinants of EIB are the sustained high level ventilation reached and the water content of the air inspired). Once this level of exercise is attained, the subject should continue exercise at that high level for an additional 4–6 minutes for a total time of 6–8 minutes. These targets are achieved more rapidly with running than cycling. Pulmonary function tests should be measured 5 minutes before the exercise and repeated 1, 3, 5, 10, 15, 20, 30 minutes postexercise; the percent fall in FEV 1 compared to baseline is recorded. Nebulized albuterol (salbutamol) or an albuterol inhaler + spacer should be prepared before the exercise test as some children may respond with a severe asthmatic attack. In our lab 200–400 µg albuterol (2–4 puffs of 100 µg) or 0.5 mL of Salbutamol nebulized solution is given to any child immediately after the last pulmonary function test (PFT) done to treat and/or evaluate responsiveness.

It is important to note that the recovery from EIB differs in children compared to adults and in younger compared with older children. For example, Vilozni and coworkers elegantly showed that in very young children (3–6 years old) PFTs should be performed several times during the first 5 minutes after the run due to a very short-lived nadir 2–3 minutes postexercise and that FEV 0.5 describes the bronchoconstriction event better than the traditional FEV 1 ( Fig. 12.8 ). Hofstra and coworkers demonstrated that 7–10-year-olds with EIB improved FEV 1 by a mean of 1.60%/min following the challenge, but improvement in 11–12 year-olds was significantly prolonged (0.54%/min). In a group of adolescents, 50% with a positive EIB test showed a maximum postexercise decline at 15 or 30 minutes after exercise cessation and previous reports support this finding. These observations should be taken into account when measuring PFTs after the exercise challenge and when symptoms or complaints indicate a high suspicion of asthma, it is reasonable to measure lung function very early (1–2 minutes postexercise) or late (30 minutes postexercise). Our standard test for demonstrating EIB is either a 6–8-minute run on a treadmill at a speed of 3–5 mph with a slope of 10% or, alternatively, cycle ergometer exercise calculated to achieve about 70% of the child’s predicted V̇O 2max . This will result in an oxygen consumption of about 60%–80% of V̇O 2max and a HR of 170–180 beats/min.

Pretest
Children should avoid PA for at least 4 hours before exercise testing because otherwise they may show an attenuated response due to refractoriness following vigorous repeated exercise. The mechanisms underlying the refractory period are not fully understood but may be due to depletion of catecholamines, prostaglandin release, degranulation of mast cell mediators, and cooling of the airways. Medications that can influence the pulmonary response to exercise should be stopped prior to the test: 6 and 12 hours for short- and long-acting beta-adrenergic drugs, respectively; 8 hours for anticholinergic drugs; and 24 hours for cromolyn sodium, leukotriene receptor antagonist (montelukast) and nonsteroidal antiinflammatory medications. Recent exposure to inhaled allergens may alter the severity of the response to exercise challenge. Caffeine-containing drinks or food should also be avoided before the test. Inhaled corticosteroids (ICS) are often discontinued 24–48 hours prior to testing, but it is not yet entirely clear whether or not ICS inhibit EIB in the testing context. Baseline pulmonary function (FEV 1 ) should be at least 65% of the predicted value. Abrupt cessation of the test or an audible complaint on the part of the patient is suggestive of an alternate diagnosis of deconditioning, upper airway dysfunction, or cardiopulmonary disorders. Some studies have shown a higher incidence of EIB in girls while others did not show a sex difference.
The diagnosis of asthma in preschool children may be difficult, as these children typically cannot perform reliable spirometric measurement of lung function. Phenomena of wheezing and prolonged expiration appearing and disappearing within 5 minutes after exercise have been described in a group of 3–6-year-old asthmatic children and may have an important clinical application in defining EIB in early childhood, in the absence of spirometry. There are promising new approaches including digitized assessment of recorded breath sounds that may prove useful in years to come in assessing EIB in younger children, a difficult age group to study.
Exercise and Other Tests for Bronchial Reactivity
Bronchial hyperreactivity to methacholine (MCH) or histamine is a characteristic feature of asthma but is also present in patients with other types of chronic obstructive lung diseases. Positive MCH results showed significantly higher sensitivity and higher positive predictive value in the diagnosis of asthma in children with postexercise symptoms than did exercise challenge tests. Free-running and sport-specific exercise challenges have been suggested to increase the specificity and sensitivity of exercise challenge test (ECT) in children and young athletes. For example, bronchoconstriction associated with a field test (e.g., free run, 6-minute run on a 100 m flat grassed track with nose clip on and continuous heart monitoring) increased the specificity of ECT in children (8–11 years old) for diagnosing asthma, recent wheezing, or atopy to 95%. The sensitivity and specificity were comparable to histamine challenge test. Although field tests may increase ECT sensitivity and specificity, such challenges performed outside the laboratory are difficult to control in terms of exercise intensity and standardizing environmental conditions.
In asthmatic children the severity of EIB may be influenced by the severity of asthma and by preexposure to allergens. Moreover, the severity, duration, and type of exercise may influence the severity of EIB. Running, as compared to swimming under the same inspired air conditions and work intensity, will result in much more EIB.
The Mechanism of Exercise-Induced Bronchoconstriction Remains Enigmatic
In the widely accepted osmolar hypothesis, dehydration of the airway during hyperventilation of inspired air during exercise leads to a hyperosmolar environment. This environment promotes movement of the water from the airway epithelium, leading to degranulation of mast cells. Mast cell degranulation precipitates the release of mediators such as transglutaminase, which preferentially activates the leukotriene pathway, histamine, and prostaglandin. Release of cellular recruitment factors promotes an influx of inflammatory cells, particularly eosinophils and T cells. The combined impact of mediator releases and influx of inflammatory cells causes bronchoconstriction and inflammation.
There have been extensive studies of the effect of climate on EIB. Breathing warm and humid air during exercise attenuates EIB, but not inevitably, while breathing dry and cold air increases its severity. Facial cooling combined with either cold or warm air inhalation causes the greatest EIB, as compared with the isolated challenge with cold air inhalation, a possible vagal effect. Respiratory heat loss (RHL) from the airway mucosa during exercise was suggested by Deal and his colleagues as the trigger for bronchoconstriction, and they showed that exercise and hyperventilation with similar RHL will result in similar bronchoconstriction. Anderson and coworkers showed that epithelial injury in the airways arising from breathing poorly conditioned air at high flows for long periods of time or high volumes with airway hyperemia can obstruct the airway (the “vascular theory”).
RHL cannot entirely account for the triggering stimulus for EIB. First, both patients with known asthma and nonaffected controls develop the same degree of RHL during exercise, yet only the patients tend to have EIB. This suggests additional mechanisms that render asthmatics more susceptible (a “second hit” phenomenon). Moreover, as noted, some asthmatic patients will develop EIB while breathing warm humid air at body temperature and humidity, which will not result in RHL. Also, Noviski and his colleagues exercised a group of asthmatic children at two levels of exercise while the respiratory heat and water loss was kept constant by altering the inspired air conditions. They found that the harder exercise with a mean V̇O 2 of approximately 1.6 times greater than V̇O 2 of the less strenuous exercise resulted in EIB which was greater by almost 1.7 times.
Exercise has been demonstrated to be a vigorous stimulant of the stress/inflammatory immune system involving increased levels of circulating IL-6, intracellular adhesion molecules, and increased circulating immune cells, many of which are involved in the pathophysiology of bronchoconstriction. Indeed, given the robust stress/inflammatory response in healthy children along with the increased number of inflammatory cells in the circulation, one might wonder why not all children wheeze when they exercise. Mediator release from circulating leukocytes and/or the airway mast cells was suggested as the intermediary pathway involved in EIB. Leukotrienes C 4 , D 4 , and E 4 were found in induced sputum and exhaled breath condensate of asthmatic patients with EIB associated with greater desquamation of epithelial cells into the airway lumen. The neutrophil is emerging as a key player early on triggering bronchospasm. Preexercise treatment with antagonists of neutrophil-related mediators reduced the severity of EIB and in some reports even prevented EIB refractoriness. There is increasing research focused on novel pharmacological agents that would specifically inhibit neutrophil migration and function that may eventually prove to be of benefit in asthma.
Air Quality, Exercise, and Asthma
As noted by McConnell and coworkers, “Incidence of new diagnoses of asthma is associated with heavy exercise in communities with high concentrations of ozone, thus, air pollution and outdoor exercise could contribute to the development of asthma in children.” Competition or training at areas close to busy roadways, or in indoor ice arenas or chlorinated swimming pools, harbors a risk for acute and chronic airway disorders from high pollutant exposure.
Exercise-Induced Bronchoconstriction in Nonasthmatics
In recent years, an increasing number of nonasthmatic children (e.g., no asthma diagnosis by a physician and/or negative methacholine/Histamine challenge test) are diagnosed with positive ECT with or without exercise complaints. Up to 50% of Olympic athletes, 29% of adolescent athletes, and 15% of the general pediatric population were reported to have this diagnosis. Most of the data were collected from control children participating in asthma and exercise studies in which they found to have positive ECT or from athletes with/without exercise complaints.
The mechanism suggested for the bronchial responsiveness in nonasthmatic children and athletes is not completely clear but seems to be strongly related to high ventilation rates in cold-dry air; time duration of the exercise challenge may be less important. Other possible mechanisms were suggested in specific circumstances (e.g., ice rink athlete exposure to high-emission pollutants from fossil-fueled ice resurfacing machines, swimmers exposure to chlorine). Airway inflammation was suggested to be involved but the reports are controversial. Some studies reported that nonasthmatic EIB positive athletes have neutrophil-dominant or mixed-type sputum compared to asthmatic EIB-positive patients who have eosinophil dominant inflammation, while other studies on airway inflammation in nonasthmatic EIB-positive athletes have found sputum eosinophilia in a higher percentage compared to asthmatics. Haby and coworkers reported that more than 50% of children with a positive free running exercise challenge had a negative histamine challenge test and concluded that mediators other than histamine may be more important in BHR to exercise or that an additional, nonmast cell mechanism may be involved in BHR to exercise.
In light of the high percentage of exercise complaints in athletes, it was suggested that there may be a different reference range for the drop in FEV 1 in elite athletes compared with the general population. One study compared sport/environment specific field-based exercise challenge (cold weather athletes) to a lab-based ECT and concluded that only a 7% drop in FEV 1 after treadmill exercise challenge in the laboratory is sufficient to make the diagnosis of EIB compared with a greater drop in FEV 1 (e.g., 15%) in a sports-specific setting.
Hallstrand and coworkers studied 256 adolescents participating in organized sports and diagnosed EIB in 9.4% of them. The screening history identified people with symptoms or a previous diagnosis suggestive of EIB in 39.5% of the participants, but only a third of these persons actually had EIB. Among adolescents with a negative review of symptoms of asthma or EIB, 7.8% had EIB. Among adolescents with no previous diagnosis of asthma, allergic rhinitis, or EIB, 7.2% had EIB diagnosed by exercise challenge. Children who screened negative on all questions about symptoms or history of asthma, EIB, and allergic rhinitis accounted for 45.8% of the adolescents with EIB. The authors concluded that “EIB occurs frequently in adolescent athletes, and screening by physical examination and medical history does not accurately detect it.” Other studies reported similar findings in athletes.
Exercise, Asthma, and Bronchoconstriction: Treatment Considerations
The approach to treatment of EIB mainly depends on a preliminary assessment of the isolated clinical condition or uncontrolled underlying asthma with more exercise focused recommendations for the nonasthmatic children and a broader approach including appropriate controller medications for chronic asthma. Therapy should result in optimal control of exercise-induced symptoms during HPA and exercise, and allow unrestricted participation in sports activity in children and athletes. Both nonpharmacological and pharmacological treatment should be discussed in the management plan ( Fig. 12.9 ).

Nonpharmacological Approaches
HPA and aerobic training should be encouraged for treatment and prophylaxis of EIB. Children tend to play in short exercise bouts (up to 1–2 minutes), and repeated exercise bouts may increase the threshold for triggering bronchospasm and decrease EIB response. Swimming is less likely to trigger EIB than running. Recommendations should include 1 hour of moderate-vigorous exercise per day and to be as active as possible the rest of the time. Preexercise warm-up is recommended for all patients to attenuate the fall in FEV 1 postexercise. Similar results were found whether high-intensity interval or variable intensity warm-up exercise was performed. Screen time was found to correlate with activity limitation in children with asthma. Daily screen time should be limited to no more than 2 hours/day and children should be as active as possible during that period.
There is evidence to support a beneficial role for diet in mitigating EIB as well. The most salient example of this is the rare but life-threatening phenomenon of exercise-induced anaphylaxis, which occurs when an individual with a food allergy (often unknown to the patient) engages in exercise several hours following a meal with the offending dietary element. It has been postulated that the mechanism for exercise-induced anaphylaxis in this instance results from the exercise-associated release (perhaps, by catecholamines) into the peripheral circulation of immune cells in the spleen and GALT (gut associated lymphoid tissue) that were locally activated by the ingestion of the food allergen prior to the exercise bout.
Increased intake of natural foods, particularly fresh fruit, vegetables, and oily fish, and decreased salt consumption are the most promising strategies to improve pulmonary function to below the clinical threshold for the diagnosis of EIB. High doses of caffeine (6–9 mg/kg) were shown to provide a significant protective effect against EIB (no significant difference from preexercise β 2 -agonist alone) in a group of adolescent-young adults. Reduction in susceptibility to EIB is yet another reason that overweight and obese children should be encouraged to modify dietary intake to gradually decrease body weight. Lower levels of vitamin D are associated with increased reactivity to exercise, and more studies are needed to evaluate the role of vitamin D in EIB management. Ascorbic acid (vitamin C) supplementation may provide a protective effect against exercise-induced airway narrowing. A 50% reduction in the postexercise FEV 1 drop and EIB-related symptoms were reported in small groups of adolescent–young adult asthmatic subjects ; other studies did not find strong correlation between vitamin C and self-reported wheezing. A Cochrane analysis concluded that evidence is not available to provide a robust assessment on the use of vitamin C in the management of asthma or EIB.
Wearing a mask or scarf in cold weather for those with cold weather–EIB offers considerable protection against bronchospasm induced by cold air. In young adults, combining a β 2 -agonist with a face mask prevented EIB and was superior to treatment with β 2 -agonist or a face mask alone. Breathing through the nose to humidify and warm the inhaled air is suggested to decrease the amount of thermal and moisture loss during exercise.
Pharmacological Approaches
Prophylactic intermittent treatment (10–15 minutes preexercise) with short-acting bronchodilators is the most commonly used treatment for EIB. β 2 -agonists, both short-acting β 2 -agonist (SABA) and long-acting β 2 -agonist (LABA), when administered preexercise in a single dose, are effective and safe in preventing the symptoms of EIB. Longer-term administration of inhaled β 2 -agonists induces tolerance and lacks sufficient safety data and is not recommended as a single therapy. A daily administration of a leukotriene receptor antagonist to ameliorate EIB is also recommended. A single-dose of montelukast was shown to attenuate EIB 1–24 hours after consumption, although it is less effective than β 2 -agonists (albuterol) for prevention of EIB in children with asthma. Tolerance to the protective effect of β 2 -agonist does not develop with regular use of montelukast and if breakthrough EIB occurs, a β 2 -agonist can be used effectively as rescue medication. Montelukast treatment added to aerobic training in asthmatic children may exert beneficial effects on BHR.
For daily or frequent EIB complaints, daily administration of an ICS is recommended. It may take 2–4 weeks after the initiation of therapy to see maximal improvement. Administration of ICS only before exercise (“as needed”) is not recommended. EIB is one of the earliest signs of chronic asthma and one of the last symptoms to disappear upon treatment with ICS ( Fig. 12.10 ). In a double-blind, randomized, placebo-controlled trial, intranasal corticosteroid (fluticasone furoate) reduced EIB in asthmatic children with allergic rhinitis. If EIB symptoms are not controlled with regular use of ICS, a LABA or montelukast is recommended. A multimodal treatment concept including physical training and medical treatment with an inhaled steroid for 7 days resulted in a decrease in airway hyperresponsiveness to exercise in children (13.5 ± 2.7 [SD] years). Adding montelukast to the treatment protocol resulted in a further decrease in airway hyperresponsiveness. Chronic treatment with a combination of inhaled corticosteroid and LABA (fluticasone propionate/salmeterol) was shown to provide superior protection compared with an inhaled corticosteroid alone (fluticasone propionate) in protecting against EIB in children with persistent asthma. To date, most of the treatment recommendations are the same between EIB positive asthmatic and EIB positive nonasthmatics. Specific irritants (e.g., cold weather, pollutants, chlorine) should be avoided if possible. It was reported that athletes with positive EIB may compete successfully at the international level despite their symptoms.

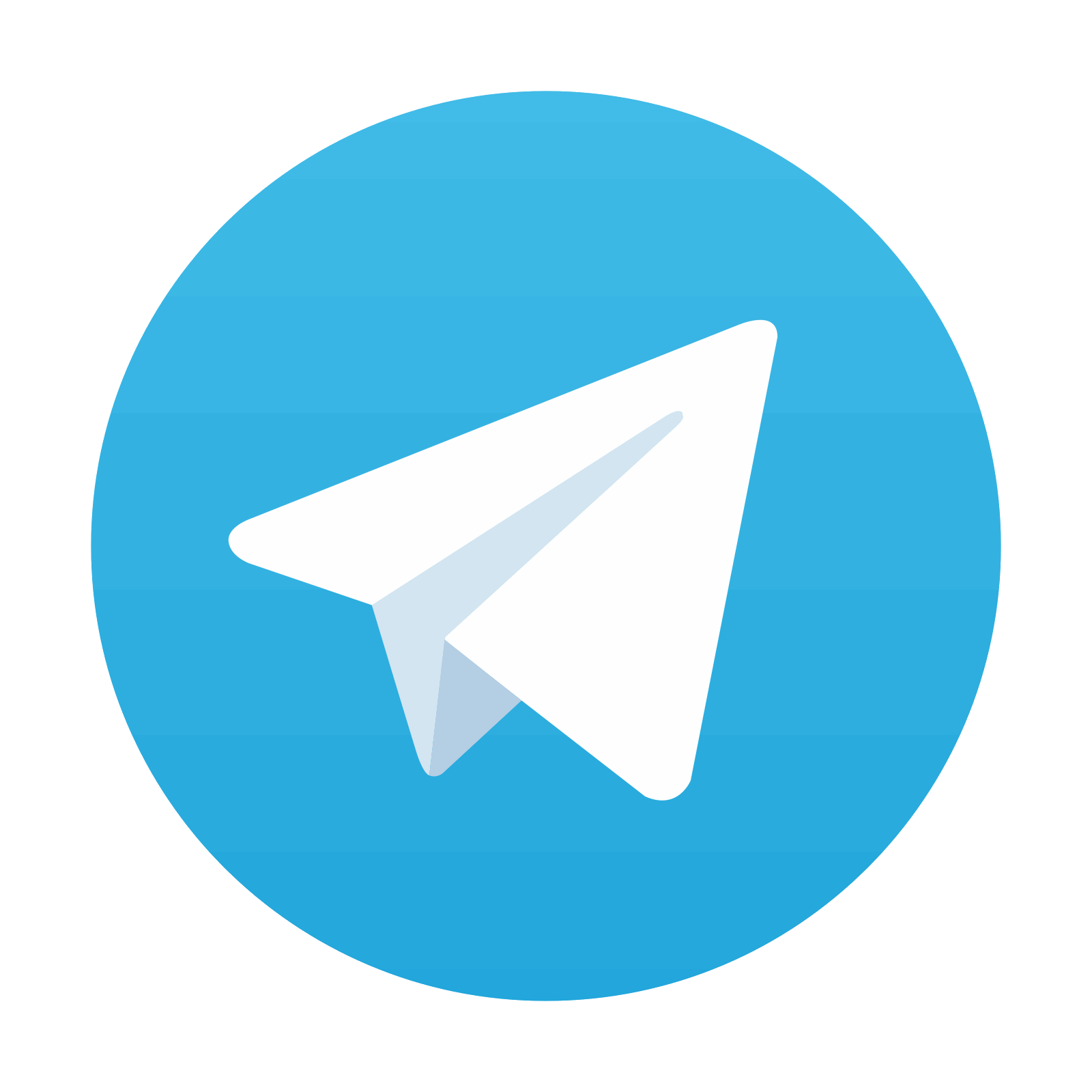
Stay updated, free articles. Join our Telegram channel

Full access? Get Clinical Tree
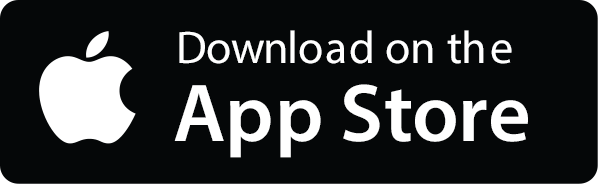
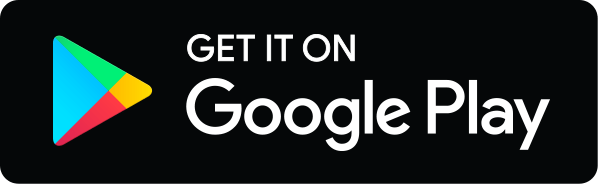
