INTRODUCTION
For over 30 years, in the diagnosis and management of patients with known or suspected heart disease, nuclear cardiology procedures have made extensive use of radioactive tracers that can be injected as a bolus and tracked during first pass through the vascular system, attached to red blood cells and in equilibrium within the vascular space or as myocardial perfusion tracers that define the endocardial borders of the left ventricle. First pass radio-nuclide angiography (FPRNA) and equilibrium radionuclide angiocardiography (ERNA) have been the most commonly used techniques capable of assessing global and regional systolic and diastolic function at rest and following supine or upright exercise. They were used extensively in the 1970s and 1980s. The advantages of these modalities over other cardiac tests available at that time included counts based on true three-dimensional measurements (independent of geometric assumptions), high accuracy, reproducibility, and absolute quantitation. However, such accurate measurements of diastolic function were difficult to perform using the standard methods of acquisition, and processing in clinical practice and efforts to achieve greater accuracy and reproducibility were time consuming, computer intensive, and not practical in most clinical settings. Echocardiography provides similar information on diastolic function and is portable and highly flexible, which offers practical advantages. In addition, echocardiography provides assessment of valves, wall thickness, left ventricular (LV) mass, chamber pressures, flow dynamics, and the integrity of the pericardium from the same study, which makes it much more valuable for patient evaluation and management. For these reasons, echocardiography has been the most practical method for the assessment of diastolic function. Due to practical and economic factors, FPRNA and ERNA techniques are rarely performed in general clinical practice, and even less for evaluation of diastolic function.
However, many of the concepts and methods developed for diastolic function analysis for FPRNA and ERNA in the 70s and 80s may be applicable today to the information available from the 8 million radionuclide myocardial perfusion imaging studies performed annually in the United States. Early radionuclide perfusion imaging did not allow the assessment of systolic or diastolic ventricular function. In the mid-1990s, several methods for assessment of systolic ventricular function using 8 time frames in the heart cycle were developed, and recently this technique has been modified to allow greater temporal sampling through 16 time frames, which can be used for the evaluation of diastolic function. This may provide incremental information to the assessment of perfusion and systolic function. In some circumstances, diastolic dysfunction may identify preclinical abnormalities in the absence of alterations of systolic function.
Given the current limited utilization of FPRNA and ERNA for assessment of diastolic function, this review will focus on the concepts and methods that have been found to be useful in the past and will then look at the opportunities for obtaining diastolic information from radionuclide myocardial perfusion studies that are currently being performed in such large numbers.
PATHOPHYSIOLOGY
Basic Principles of Radionuclide Assessment of Diastolic Function
All radionuclide approaches for assessing systolic and diastolic LV function require the generation of a curve plotting the changes in radioactivity, which is proportional to changes in LV volume over time, as shown in Figure 9-1 . From this time-activity curve (TAC), the first derivative, which measures the change in volume over time, is derived and used to calculate the most rapid changes in ejection and filling and the time when the maximal rate occurs. The typical ranges of normal values for these diastolic parameters in humans are shown in Box 9-1 .

- 1.
Peak filling rate (PFR) (>2.5 end diastolic volumes/second)
- 2.
Time to peak filling rate (TPFR) (<180 msec)
- 3.
Filling fraction (% of stroke volume at one third, one half, or two thirds of diastole)
- 4.
E/A ratio, 1:40
The left side of the curve prior to end systole (ES) in Figure 9-1 provides information on the systolic function of the ventricle as expressed by the rate of ejection, measured as end diastolic volumes per second (EDV/sec), and the time at which this peak ejection occurs, expressed as time to peak filling rate (TPFR). For ventricles with muscle damage or infiltration, pericardial abnormalities, or ischemia, the rate of emptying is decreased and there is prolongation of the time when the peak emptying rate is achieved. Diastole is represented to the right of end systole in Figure 9-1 and is a much more complicated process, which consists of four distinct phases:
- 1.
Isovolumic relaxation
- 2.
Peak filling rate (PFR)
- 3.
Diastasis
- 4.
Atrial systole
Isovolumic relaxation starts at end systole, is an energy-dependent process, has a short duration (usually <50 msec), and ends when pressures in the left ventricle fall below the left atrial (LA) pressure, the mitral valve opens, and rapid filling begins. PFR represents the most rapid ventricular filling and is normalized to EDV/sec, which is normally greater than 2.5. Generally, it has been shown to be rapid in young and healthy hearts but declines in the elderly, even in the absence of pathology. TPFR is an important measure of diastolic function and is less than 180 milliseconds in normal ventricles. There have also been attempts to look at the percent of stroke volume filling that occurs during the rapid filling phase as an indicator of disease. Generally, more than 69% of the stroke volume should fill during the rapid filling portion.
Following rapid filling, there is a separate phase, diastasis, with minimal changes in volume, which is followed by atrial systole. At slower heart rates, a distinct atrial component can be identified and the peak measured to calculate an E/A ratio similar to that obtained by echocardiography. For nuclear techniques, a ratio of less than 1:4 is normal but increases with age. At faster heart rates, the duration of diastasis decreases and the rapid ventricular filling and atrial systolic portions of the TAC merge, making it difficult to calculate ratios and assess the relative contribution of the two phases.
This chapter will focus on disorders of diastolic function resulting from abnormalities of the myocardium and ischemia and not address abnormalities resulting from valvular or pericardial disease. Caution must be used in applying these normal value ranges to a particular patient, as the method of acquisition and processing, as well as patient-specific parameters, will result in great variability in the values independent of any changes caused by cardiac pathology. As shown above, factors such as age, heart rate, systolic function (ejection fraction [EF]), end diastolic volume, adrenergic state, and medications will alter these parameters in the absence of pathology. Given the influence of these multiple factors on the derived diastolic values, it is easy to see that single or even multiple variables may not accurately identify the presence or absence of pathology in a given individual.
Figure 9-2 is a schematic representation of a normal TAC in the presence of diastolic dysfunction, shown as the dashed line. With decreased relaxation, the rate of filling, expressed as EDV/sec, decreases and the TPFR is delayed and shifted to the left. Under such circumstances, the contribution of atrial systole may be increased and the E/A ratio shifted to a greater reliance on atrial filling. In patients with very stiff ventricles, such as in hypertrophic cardiomyopathy (HCM) or diminished systolic ventricular function, the loss of the atrial contribution to filling caused by rhythms other than sinus can rapidly lead to pulmonary edema.

Equilibrium Radionuclide Angiocardiography Methods in Diastolic Assessment
Data Acquisition
Important variables for performing diastolic function analysis using ERNA are listed in Box 9-2 . Technetium (Tc)-99m pertechnetate is the radioisotope of choice and is attached to the patient’s own red blood cells using one of three possible labeling methods, which vary in the time to perform labeling, expense, and labeling efficiency. Both planar and single photon emission computed tomography (SPECT) methods of acquisition are available, but nearly all studies are performed using planar techniques, with the patient positioned in the left anterior oblique (LAO) view, which allows the best separation of the right and left ventricles for independent and accurate volume measurements in each chamber. An example of a typical LAO view is shown in Figure 9-3 . The labeled red blood cells circulate in the vascular space, and the patient’s electrocardiographic (ECG) signal is used to set the timing for acquisition of each heart beat at individual time points, which may vary from 10 to 150 msec, depending on heart rate and the preset parameters. Information for each time point for each beat (usually >400 beats are acquired) is summed so that the final TAC is an average rather than information from a single beat or a small number of beats, as is provided by other modalities. For this reason, this technique may be more representative of overall function than are other methods, due to the large number of beats averaged.
- 1.
20–30 millicuries Tc-99m labeled red blood cells
- 2.
Labeling methods
- a.
In vivo : fastest and least expensive but lowest binding efficiency
- b.
Modified in vivo / in vitro : compromise with good labeling efficiency
- c.
In vitro : longest time and expense but best labeling efficiency
- a.
- 3.
Planar or single photon emission computed tomography (SPECT)
- 4.
Positioning: Best septal separation, left anterior oblique (LAO)
- 5.
Arrhythmia rejection: ±10% of mean R-R interval and drop postpremature beat
- 6.
Temporal resolution: <50 milliseconds or 16–32 frames/cycle
- 7.
Acquisition
- a.
Frame mode: simplest and least data intensive
- b.
List mode: optimal but adds considerable processing time and data storage
- c.
Forward-backward with buffered beat: compromise
- a.

Unlike systolic function analysis, where changes in heart rate and arrhythmias have relatively little influence on the time to end systole and EF, diastolic parameters are markedly affected by heart rate variability during acquisition and processing. To avoid volume and filling inconsistencies due to heart rate variability and arrhythmias from such a large number of beats, the R-R interval is sampled prior to acquisition and the parameters set up to reject individual beats that vary by ±10% of the mean R-R. Since the beat following a rejected beat has a longer filling period and will add variability to the measurements due to differences in end diastolic volume and EF, it is also rejected.
There are three computer methods used to acquire ERNA studies: frame, list, and buffered beat acquisition. Frame mode is the simplest method. It samples the R-R interval prior to acquisition, determines the number of time frames for each beat, and puts data into the appropriate time frame in real time for each beat using the set parameters until a new ECG gating signal identifies the beginning of a new contraction. Once a beat has been added to the particular time bin, it cannot be removed. If there is an early beat, a new ECG trigger resets the acquisition so that the time bins toward the end of the heart cycle will have fewer beats contributing counts. The TAC generated from this method of acquisition suffers from count dropoff in the terminal frame(s) due to heart rate variability; and although accurate for EF measurements, these curves cannot be analyzed for diastolic parameters using harmonic analysis. Although this is not the best method, it is universally used because of its simplicity and the small storage size of the retained data.
List mode acquisition is the best method. It retains the X,Y spatial distribution of the radiotracer for every millisecond of acquisition, along with the ECG trigger signal so that the data set can be reformatted into any timing interval and use the appropriate arrhythmia rejection during postprocessing. It provides maximal flexibility relative to heart rate variability, but unfortunately at the expense of prolonged processing time and massive data storage. This technique was used exclusively for all studies performed by the group at the U.S. National Institutes of Health.
A hybrid method, the buffered beat approach, is an attempt to provide realistic flexibility for heart rate variability while keeping data size to a manageable limit. It uses a temporary memory buffer to examine each beat and the ECG gating signal with regard to the set baseline parameters and makes an instant decision to keep or reject the beat. An additional feature of this method is a forward-backward curve generation technique to avoid discontinuities or count dropoff in the last several frames, which precludes harmonic analysis of the TAC.
Data Analysis
For analysis of diastolic indices, there needs to be adequate temporal sampling of LV volume to capture the fine detail required to detect minor or subtle alterations in diastolic filling. The actual sampling interval will vary with heart rate. To achieve a temporal resolution under 50 msec usually requires 16–32 frames per heart cycle with frame mode or buffered beat. At slow heart rates, more frames are required than at faster heart rates. The processed images are filtered to reduce statistical noise, the edges of the left ventricle are defined using manual or automated edge detection software, and background subtraction is performed. The radioactive counts within these boundaries represent the total volume in the ventricle and are used to produce a TAC. A TAC from a frame mode of 16 time intervals acquired from a clinical study is shown in Figure 9-4 . All the fine detail shown schematically in Figure 9-1 is retained in this curve, which does not have any dropoff in counts in the terminal frames that may be seen in the presence of even minor sinus arrhythmias but is much more pronounced in the presence of atrial or ventricular arrhythmias. Figure 9-5 is from a study with 32 frame intervals. In comparison with the 16-frame study shown in Figure 9-4 , the higher temporal resolution results in even more detail during the diastolic filling period.

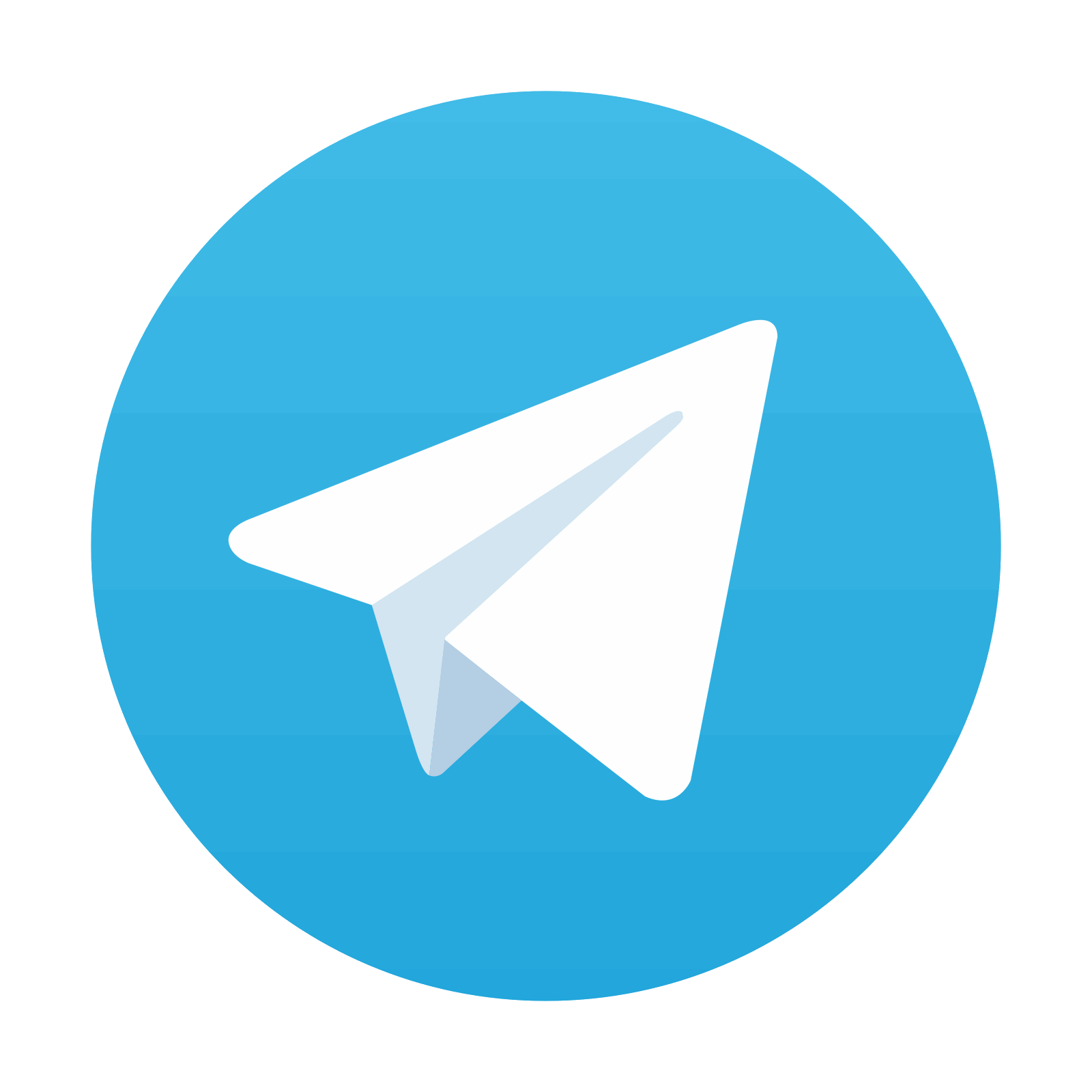
Stay updated, free articles. Join our Telegram channel

Full access? Get Clinical Tree
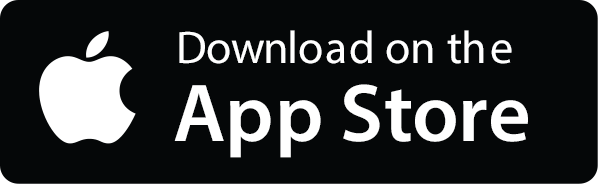
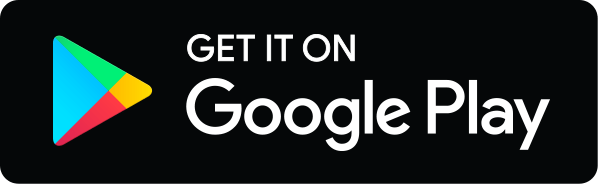
