Chapter 25 Endovascular Treatment of Renovascular Disease
Natural History
Atherosclerosis affecting the renal arteries is a progressive disease that often results from encroachment of aortic plaque into or across the renal artery orifice. Because of this characteristic pattern, most clinically relevant atherosclerotic renal artery lesions are ostial in location, occurring within the most proximal 5 mm of the vessel. Once RAS becomes hemodynamically significant (>60%), progression to renal artery occlusion is a real possibility. Using sequential angiograms, Tollefson and Ernst1 found that RAS progressed at approximately 5% per year, irrespective of the level of stenosis, and that occlusion was associated with greater degrees of stenosis. Caps and associates2 showed that 49% of patients with RAS greater than 60% measured by duplex ultrasonography showed disease progression over a 3-year period.2 Zierler and colleagues3 reported that in renal arteries with stenosis greater than 60%, progression to occlusion was 5% at 1 year and 11% at 2 years. Renal artery occlusion is associated with loss of nephrons and atrophy of the kidney parenchyma, with a mean decrease in kidney length of 1.8 cm in Zierler’s report. Renal atrophy can also occur in association with stenotic but nonoccluded renal arteries.
Imaging Studies
Renal duplex ultrasonography has emerged as an excellent test to screen patients for RAS. Although it requires significant technical expertise, it is a safe and relatively inexpensive modality that can provide information concerning kidney size, cortical thickness, renal artery hemodynamics, and velocity profiles, as well as nonvascular anatomic renal abnormalities such as cysts. Several authors have documented the accuracy of using renal artery peak systolic velocities to detect RAS. A peak systolic velocity greater than 180 cm/s, along with a renal-to-aortic velocity ratio of 3.5 or greater, is used in many vascular laboratories to identify significant RAS. In the author’s experience, a peak systolic velocity greater than 220 cm/s identified RAS with 91% sensitivity and 85% specificity.4 Importantly, the negative predictive value of 95% of this threshold value in our laboratories essentially eliminated the possibility of RAS, making duplex ultrasonography a highly useful screening test. Limitations of ultrasound imaging of the renal arteries include large body habitus and overlying bowel gas obscuring identification of the renal arteries.
Renal duplex ultrasonography to measure renal arterial resistance may also offer a method to predict outcomes following therapy for RAS.5,6 In a study by Radermacher and colleagues,5 duplex ultrasonography was used to measure the renal resistance index in 138 patients who had unilateral or bilateral renal artery stenosis of more than 50% and underwent renal angioplasty or surgery. The procedure was technically successful in 95% of patients. Creatinine clearance and 24-hour ambulatory blood pressure were measured before renal artery stenosis was corrected and at intervals of 3, 6, and 12 months and yearly thereafter. Mean follow-up was 32 months. Patients with an elevated renal resistance index (27% of the cohort) failed to realize an improvement in blood pressure, whereas those with a normal index experienced a statistically significant improvement in mean arterial pressure at 3-year follow-up. Creatinine clearance declined in 80% of patients with an elevated renal resistance index despite technically successful revascularization. Forty-six percent of patients with an elevated renal resistance index became dependent on dialysis during follow-up, and 29% died. Patients with a normal resistance index experienced a significant increase in creatinine clearance, followed for 60 months.
Magnetic resonance angiography (MRA) using gadolinium is also a useful tool for evaluating renal artery anatomy. It has a sensitivity of 90% to 100% and a specificity of 76% to 94% when compared with conventional arteriography.7,8 As a screening modality, MRA is more expensive and less patient friendly than duplex scanning, but it has become a valuable diagnostic tool and is often the first-line screening test in institutions without reliable duplex scanning results.
Using any of these modalities, the diagnosis of RAS can be reliably excluded, but conventional angiography is usually required for confirmation of positive results and to guide endovascular interventions. In the presence of renal insufficiency, carbon dioxide or gadolinium have been used instead of iodinated contrast agents.9–11 Although carbon dioxide and gadolinium have limitations, and the image quality is inferior to that obtained using conventional iodinated contrast agents, renal artery imaging is possible and reproducible using either of these alternatives. These agents are particularly valuable for patients with ischemic nephropathy, in whom even small amounts of iodinated contrast may be injurious. Recent concern regarding the use of gadolinium in patients with renal dysfunction and its association with nephrogenic systemic fibrosis has limited its use.12
Endovascular Management
Groin access via the femoral artery is used most commonly, although a brachial artery approach should be considered for renal arteries with significant caudal angulation or in patients with significant aortoiliac occlusive disease (Figure 25-1). For unilateral RAS, it is preferable to access the contralateral femoral artery, which can provide more secure access to the target vessel. Following percutaneous arterial access and placement of a 5-French sheath, a multi–side-hole catheter is placed in the abdominal aorta and positioned at the level of the L1 to L2 vertebral interspace for flush aortography. Complete visualization of the renal artery origins usually requires anteroposterior and oblique views. Catheterization of one or both renal arteries may be required for definitive angiographic detail. Translesion pressure measurements may be performed for lesions of questionable hemodynamic significance. Documentation of significant RAS is followed by upsizing to a 6- or 7-French sheath. Several sheaths are available with preformed curves at their tips that are designed for placement into the aortic branches. Alternatively, the operator may choose to place a shaped guiding catheter through the femoral sheath and into the renal artery. The shaped sheath or guiding catheter provides support against the opposite aortic wall that helps to stabilize access to the renal artery.
Placement of the sheath or guiding catheter at the renal orifice allows small amounts of contrast to be injected to guide the precise placement of angioplasty balloons and stents (Figure 25-2). In addition, the sheath or guiding catheter provides external support for the subsequent passage of guidewires, balloons, and stent delivery systems. This arrangement also allows complete imaging without the loss of the guidewire crossing the lesion.
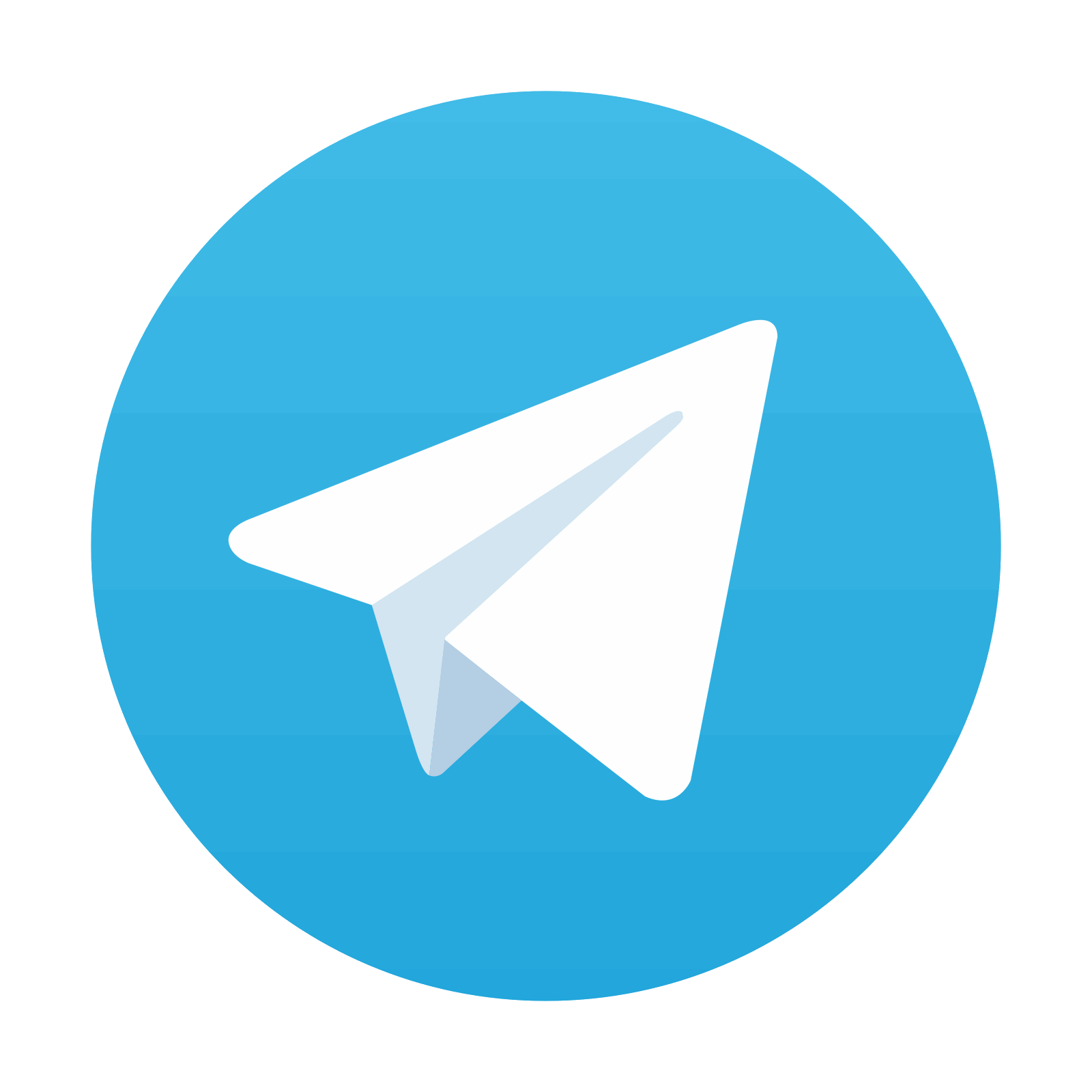