Endovascular Revascularization for Infrainguinal Arterial Occlusive Disease
Daniel G. Clair
Amir Kaviani
Peripheral arterial occlusive disease of the lower extremity is a common problem facing vascular surgeons and one that is increasing in prevalence with our aging population. Epidemiologic studies have demonstrated that up to 5% of men and 2.5% of women over the age of 60 are afflicted with symptomatic disease. Moreover, the condition is being diagnosed more frequently in frail, elderly patients with multiple comorbidities. These patients may not be candidates for traditional, open surgical revascularization, given its associated morbidity. A discussion about the endovascular options for treating lower-extremity occlusive disease in this setting is not only appropriate but also necessary.
Until recently, vascular surgeons have viewed endovascular therapy for lower-extremity arterial occlusive disease as a treatment to be used only in patients with focal disease limited to the suprageniculate arteries. These views were based on early reports of lower-extremity percutaneous transluminal angioplasty (PTA), published more than two decades ago, demonstrating results that were equivalent to open surgical revascularization for short, focal lesions and inferior for more extensive disease, particularly in the vessels below the knee. Notably, a small minority of the patients in the early PTA trials (15%) had critical limb ischemia at the time of treatment that required direct, inline flow to achieve limb salvage and, thus, were likely poor candidates for the less invasive option. Finally, most vascular surgeons have had limited hands-on experience with the percutaneous approaches until more recently. This lack of experience undoubtedly contributed to the limited initiative in pursuing these options for patients with infrainguinal occlusive disease. As surgeons have become more skilled in using these therapies, they have become aware of their distinct advantages and have recognized that they can achieve limb salvage while avoiding open operation in appropriately selected patients.
This chapter focuses on the endovascular treatment options for managing infrainguinal arterial occlusive disease. Specifically, the indications and operative techniques for transluminal as well as subintimal angioplasty with or without stent placement will be discussed. In addition, we will discuss the role of atherectomy. It is the author’s experience that the blood flow to the lower extremity can be improved in up to 90% of appropriately selected patients using these approaches.
Pathogenesis
Progressive atherosclerosis is the most common underlying etiology of lower-extremity arterial occlusive disease. Although the exact mechanisms responsible for the atherosclerotic changes remain to be fully elucidated, it is clear that cholesterol plays a central role in plaque accumulation and disease progression. Elevated serum cholesterol levels initiate a process of endothelial cell activation. The cholesterol particles, retained in the vessel wall at areas of hemodynamic stress, are modified by the endothelial cells and become oxidized/activated. These modified lipids then cause the endothelial cells themselves to be activated, thereby initiating the adhesion of platelets and macrophages to these areas of “injury.” The adherent platelets and macrophages further activate the endothelial cells in the area, while the macrophages enter the vessel wall and actively scavenge the activated cholesterol particles. These cells, commonly referred to as “foam cells,” contain highly active lipid particles with oxidative potential. These activated cells within the vessel wall lead to further activation of the endothelium and adhesion of additional platelets and other inflammatory cells. The inflammatory process soon becomes self-sustaining with the adhesion/ activation of a range of inflammatory cells. These cells lead to tissue destruction and release of active oxygen and nitrogen species that cause breakdown of the intercellular matrix of the vessel wall. Destruction of the architecture of the normal vessel wall leads to vessel remodeling with luminal loss and instability. The affected vessels can have a significant reduction of the lumen from the deposition of more lipid within the wall or from hemorrhage within injured vessel walls (i.e., intraplaque hemorrhage). Additionally, these areas can rupture, leading to distal embolization and the exposure of the extremely thrombogenic material and further activation of the process. While cholesterol alone was initially thought to be the etiology of atherosclerosis, it has become increasingly clear that the inflammation within the vessel wall plays a major role in plaque formation.
Clinical Presentation
Patients with lower-extremity arterial occlusive disease often present with symptoms related to a hemodynamically significant stenosis within the superficial femoral artery. The vessel is affected most often where it emerges from the adductor foramen or the region referred to as Hunter’s canal. The
mechanisms responsible for the development of atherosclerosis in this specific location remain unclear but may be related to the surrounding tendinous structures. Additionally, occlusive lesions both in the lower extremity and throughout the body frequently develop at the site of arterial bifurcations (e.g., common carotid bifurcation, common femoral artery bifurcation), presumably due to changes in shear stress within the vessel wall.
mechanisms responsible for the development of atherosclerosis in this specific location remain unclear but may be related to the surrounding tendinous structures. Additionally, occlusive lesions both in the lower extremity and throughout the body frequently develop at the site of arterial bifurcations (e.g., common carotid bifurcation, common femoral artery bifurcation), presumably due to changes in shear stress within the vessel wall.
The risk factors for lower-extremity atherosclerosis are the same as those for the other vascular beds. These include family history, hypertension, hyperlipidemia, diabetes mellitus, smoking, and obesity. Controlling the modifiable risk factors can alter the progression of the disease but does not completely eliminate the associated risk. Before treating hemodynamically significant lesions, the physician must address the patient’s risk factors in an attempt to reduce the risk of disease progression and recurrence.
Patients with lower-extremity arterial occlusive disease will present with a spectrum of symptoms ranging from mild claudication to extensive tissue loss so severe that it may preclude limb salvage. Patients with claudication will typically describe activity-induced muscle pain, cramping, or fatigue. Depending upon the location of the stenosis, these symptoms can occur in the buttock, hip, calf, or foot. For those with disease below the inguinal ligament, the symptoms usually occur in the calf or foot. The extent of exercise necessary to induce these symptoms varies and depends upon the speed at which the individual is walking, the angle of ascent, and the patient’s general cardiovascular health. In some patients with severe claudication, the symptoms may develop at <100 feet. These patients are often unable to do any activity outside their own home without the onset of lower-extremity pain. The symptoms may be masked by the presence of a peripheral neuropathy in diabetics. The neuropathy makes it difficult for the patients to distinguish the usual symptoms of claudication, and the vascular insufficiency may contribute to further progression of the neuropathic changes. In addition, diabetics have impaired wound healing and are predisposed to developing soft tissue infections in the foot; both concerns merit an aggressive approach to revascularization.
Patients with more advanced disease may present with nonhealing ulceration or progressive tissue loss. These patients truly have limb-threatening ischemia and often require amputation without revascularization. In addition, a subset of these patients will present with nonhealing wounds after minor surgical procedures on their feet (e.g., ingrown toenail excision). These patients also require revascularization in order to avoid further tissue loss and amputation.
Indications
The indications for treatment have remained fairly stable and are dictated by the ischemic symptoms at presentation. Patients with limb-threatening ischemia have a significant risk of amputation without treatment and merit revascularization unless contraindicated. The indications for patients with claudication are less clear, but revascularization appears justified in patients with shortdistance claudication (<100 ft) or those with lifestyle/economically limiting symptoms.
The healthcare provider must weigh the relative risks and benefits of intervention for each patient with vascular disease similar to the decision algorithm for all medical treatments. As the risk of the procedure decreases, assuming similar benefits, the operative indications may change, because the risk:benefit ratio has been altered. Indeed, this appears to be the situation for the endovascular procedures in patients with limb-threatening ischemia and claudication. In the hands of an experienced endovascular surgeon, the risk and disability from percutaneous revascularization has significantly decreased, thereby justifying a more aggressive approach. This is particularly relevant for patients with poor overall health in whom the potential complications associated with open revascularization would be prohibitive.
The potential to decrease peri-operative morbidity, even in healthier patients, has encouraged the authors to explore all endovascular options. In our current approach, the diagnostic arteriograms are reviewed to determine whether percutaneous revascularization is an option, and revascularization is attempted at the same setting if feasible. Important factors in the decision-making process include the extent of the occlusive disease and the status of the outflow vessels. In cases where only one arterial segment (i.e., femoral, popliteal, tibial) is involved and the outflow is nondiseased, percutaneous therapy is a reasonable initial option. Predictably, the likelihood of success diminishes with the number of involved segments and is quite small when all three segments are involved. It is important to recognize that the complication rate associated with endovascular revascularization varies with the anatomic segment. For example, the risk of perforation and dissection is significantly higher in the popliteal segment when compared to that of the common femoral and superficial femoral arteries. These factors should be taken into consideration especially when planning revascularization in multiple segments.
It is important to stress that the revascularization plan should be tailored to the clinical scenario at hand, and the endovascular surgeon should be able to use different treatment modalities. With the advent of newer endovascular tools (e.g., atherectomy devices, cryoplasty balloon), the indications and potential application of endoluminal therapies will likely further expand. The following sections provide a straightforward approach to interventions in the infrainguinal arterial tree. The specific plan must take into account the clinical situation as well as the endovascular surgeon’s experience and level of comfort with the proposed procedure.
Endovascular Technique
There are a number of basic techniques for percutaneous revascularization of the lower extremity. The most commonly used modalities include PTA, subintimal angioplasty, intravascular stenting, and atherectomy. In most situations, one of these modalities or a combination thereof can be used to achieve a successful result. These techniques will be addressed individually with the understanding that they may be used simultaneously as necessary to achieve the desired result. Indeed, it is important to be facile with these different techniques, because they may be required as a remedial or “bailout” procedure if the initial angioplasty is unsuccessful or complications arise.
Percutaneous Transluminal Angioplasty
The angioplasty technique for the lower-extremity vessels is essentially the same as that used in the other anatomic locations. Indeed, the principles of PTA have not changed significantly over the past few years, although changes in the technology (i.e., lower-profile balloons, higher-pressure balloons) have expanded the lesions amenable to treatment. Access to the lesions can be obtained in either an antegrade or retrograde femoral approach. Although the retrograde approach is more familiar to most surgeons, the antegrade approach offers several advantages and should be considered. The catheter and wire
control with the antegrade approach are predictably much better given the relatively short distance from the puncture site to the target lesion. The requisite wires/catheters/ balloons are likewise much shorter and the antegrade approach obviates any concerns about tortuosity in the aortoiliac vessels. The antegrade approach can be somewhat difficult given the limited working room for obtaining access to the superficial femoral artery, and it is contraindicated in both obese patients and those with very proximal superficial femoral artery lesions. It is imperative to confirm that the necessary equipment is available before starting any endovascular procedure. This is particularly a concern when treating the infrageniculate vessels using the retrograde approach, given the associated working length.
control with the antegrade approach are predictably much better given the relatively short distance from the puncture site to the target lesion. The requisite wires/catheters/ balloons are likewise much shorter and the antegrade approach obviates any concerns about tortuosity in the aortoiliac vessels. The antegrade approach can be somewhat difficult given the limited working room for obtaining access to the superficial femoral artery, and it is contraindicated in both obese patients and those with very proximal superficial femoral artery lesions. It is imperative to confirm that the necessary equipment is available before starting any endovascular procedure. This is particularly a concern when treating the infrageniculate vessels using the retrograde approach, given the associated working length.
Percutaneous interventions using the retrograde approach are begun by gaining access to the contralateral femoral artery (Figs. 52-1A, 52-1C, 52-1E, 52-1F, 52-1G). We prefer to use a 21-gauge needle and a 0.018-inch wire (micropuncture kit, Cook, Inc. Bloomington, Ind.) and have found this combination to be very safe in terms of access complications. The 21-gauge needle and 0.018-inch wire are exchanged for a 3 French sheath, and the sheath is confirmed to be intraluminal by injecting contrast. A starting 0.035-inch guidewire (e.g., Bentson, Cook Inc., Bloomington, Ind.) is then advanced under fluoroscopic guidance and the 3 French sheath is exchanged for a 5 French sheath. An aortogram in the anteroposterior projection is then obtained after positioning a 4 French reversed curve flush catheter (e.g., Contra, Boston Scientific, Natick, Mass.) between the L1-2 vertebral bodies. A pelvic arteriogram is then obtained in the anteroposterior projection by withdrawing the catheter back until it is positioned immediately proximal to the aortic bifurcation. Additional oblique images can be obtained as necessary to exclude any significant stenoses within the aortoiliac segment. The hemodynamic significance of any questionable lesions can be interrogated by assessing the pressure gradient across the lesion both at rest and after administration of an intra-arterial vasodilator.
Access to the contralateral femoral artery is then obtained using the curved flush catheter and the guidewire. This can be performed by advancing the wire to the end hole of the catheter, thereby splaying out its curve. The catheter and wire combination in this splayed-out configuration can then be withdrawn and used to engage the aortic bifurcation. The guidewire can then be directed and advanced through the external iliac artery into the superficial or profunda femoris artery. Anchoring the guidewire in these vessels allows the catheter to be advanced over the aortic bifurcation and seated in the common femoral artery above its bifurcation. The image intensifier is then angled laterally toward the affected extremity (20° ipsilateral oblique) to optimize visualization of the common femoral and the proximal superficial and profunda femoris vessels, and an arteriogram is obtained. The remaining portion of the lower extremity is then imaged in the anteroposterior projection and helps to serve as a baseline of comparison for the subsequent interventions.
If a hemodynamically significant lesion amenable to endovascular treatment is identified, the 5 French sheath should then be exchanged for a long sheath (e.g., 55 cm Raabe, Boston Scientific, Natick, Mass.). The choice of sheath diameter is dictated by the planned endovascular procedure (i.e., angioplasty alone or angioplasty/ stent) with the usual choice of sizes being 6 French or 7 French. It is frequently necessary to replace the selective wire with a stiffer 0.035-inch exchange wire (e.g., Rosen, Boston Scientific, Natick, Mass.) to facilitate passing the long sheath over the aortic bifurcation. Ideally, the sheath should be advanced to within 10 cm of the target lesion, although this is not always possible given the distance from the contralateral femoral artery. The sheath affords many advantages, including the ability to perform multiple arteriograms without removing the guidewire, improved catheter mechanics, and an easy-access roadway. The specific lesion is crossed using a selective, hydrophilic 0.035-inch wire (e.g., Glidewire, Terumo Corp., Japan), and this can be facilitated using a 4 French angled, hydrophilic catheter (e.g., Glidecatheter, Terumo Corp., Japan) if necessary. The guidewire should be advanced a comfortable distance beyond the lesion to avoid dislodgement during the subsequent steps of the procedure. It is imperative to confirm the position of the wire throughout the procedure to avoid injuring the distal vasculature. Standard 0.035-inch wire systems are used for the superficial femoral and popliteal arteries, while 0.014-inch wire systems are optimal below the knee because they facilitate using lower-profile balloons. However, these lower-profile systems lack some of the pushability and trackability associated with their larger counterparts. Patients are aggressively heparinized (100 units/kg initial dose with subsequent doses as needed to maintain the activated clotting time >250 seconds) after the target lesion is successfully crossed.
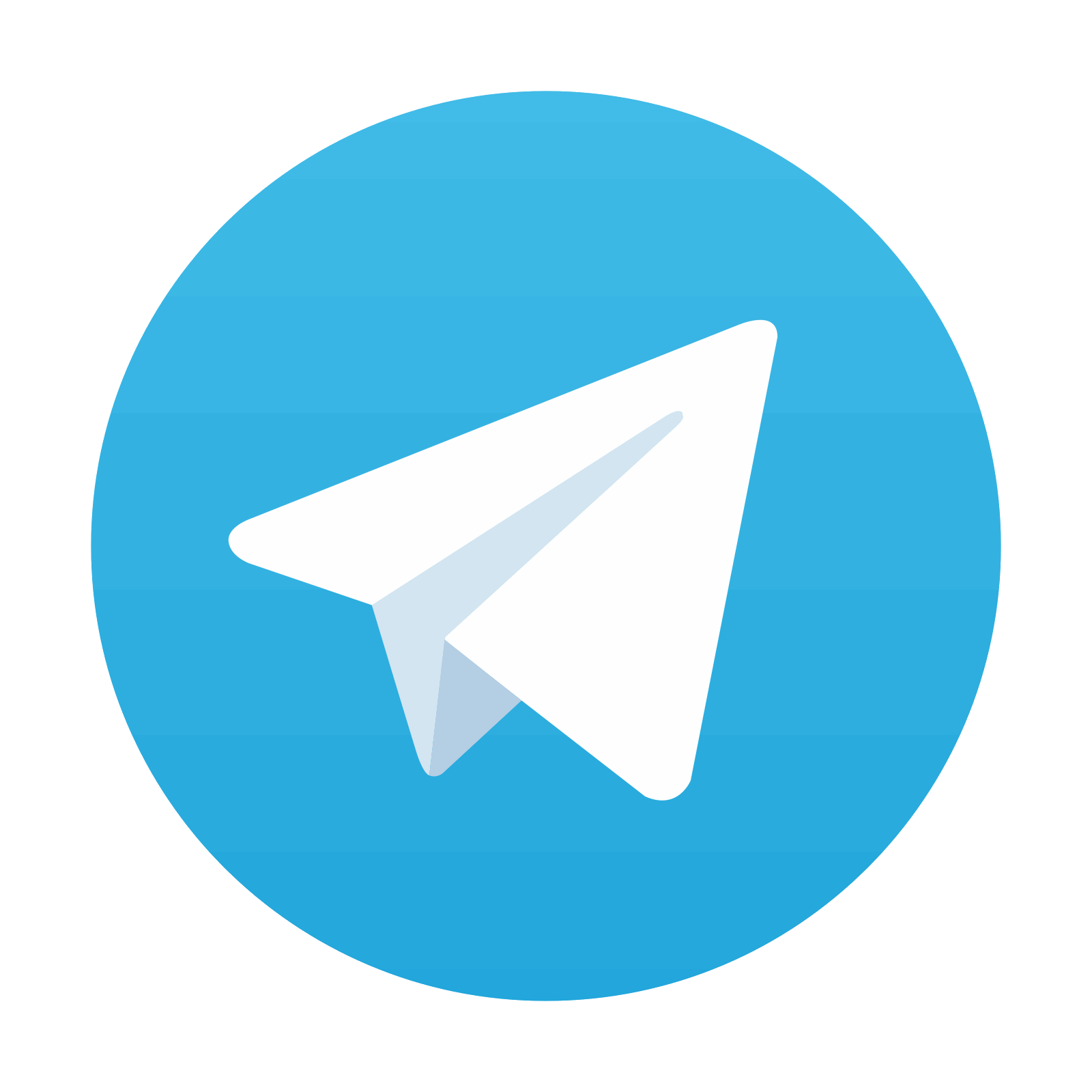
Stay updated, free articles. Join our Telegram channel

Full access? Get Clinical Tree
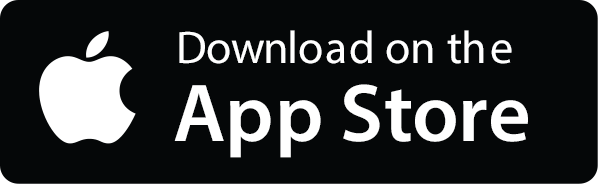
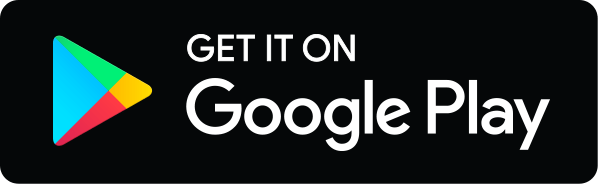