Chapter 2 Embryology of the Vascular System
Early History
An organism of a cubic millimeter or so in volume (depending on the surface area and other factors related to the effectiveness of diffusion) may thrive without a vascular system. The human embryo enjoys the elaboration of a vascular system from its earliest stages, almost as if it can anticipate that its bulk will soon require a highly sophisticated transport system. As the embryonic disk becomes recognizable, blood islands rapidly accumulate around the periphery of the disk. These isolated “puddles” begin to coalesce and communicate with one another until the embryo resembles a bloody sponge. Most prominent is the precephalic region, where the seemingly random coalescence of blood islands forms a network in the region soon to be identified as the cardiogenic plate (Figure 2-1A).
Heart
The now-fused heart tube (see Figure 2-1B) begins to invaginate the presumptive pericardial cavity, acquiring its visceral and parietal layers of pericardium while still a single-chambered heart configured as a simple, relatively straight tube. As the somites begin to appear in the neck and trunk region, the heart tube begins to fold on itself, first bulging ventrally, further invaginating the pericardial sac. The heart that is now swinging ventrocaudally comes to lie in front of the head and will continue its descent down the front of the neck and into the anterior chest. The ventrally directed bulge created by the U-shaped fold of the heart characterizes the loop stage.1 The ventral limb of the U is the arterial outflow path, and the dorsal limb of the U will become the venous inflow tract (see Figure 2-1C to E). By the 10-somite stage, approximately 3 weeks’ ovulation age, the heart has begun to fold in a coronal plane as well, directing the ventricular region to the left and forming a recognizable outflow tract, now termed the bulbus cordis, whose distal part is called the truncus arteriosus (see Figure 2-1C). At this stage, the heart is still a single-chambered structure innocent of valves but completely enclosed in a pericardial sac and demonstrably beating, albeit irregularly. There is no single primordium, no segment of the primitive heart tube, that can be identified as leading to a specific cardiac cavity in the early postloop stage. Instead, there are microscopically and experimentally identifiable zones, each of which gives rise to a specific anatomic region of a definitive cardiac cavity. These primordia are most accurately termed primitive cardiac regions; therefore referring to segments of the heart tube as forerunners of the chambers of the fully formed heart is misleading.1 The folds in the heart tube and the peristaltic nature of myocardial contraction lead to a predetermined direction of flow out through the bulbus cordis, the folds acting as inefficient valves to direct the flow. Such early vitality is not surprising, because the cardiovascular system is the earliest to attain form and function among the organ systems of the body. The heart is disproportionately large for the size of the embryo at this stage, and this disproportion remains until birth, with only a modest decline in heart-to-body ratio toward birth. Obviously, this occurs because the heart must support not only the growing tissue of the organism but also the embryo’s share of the enormous placental circulation.
Arteries
During the early folding of the heart, and with identification of a bulbus cordis and truncus arteriosus as an outflow tract, the aortic arches are beginning to form. The truncus arteriosus is continuous with a ventral aorta. This large, single-channeled artery is connected to a pair of dorsal aortas through a series of branchial (pharyngeal) arch arteries. The developing pharynx passes through a period in its development when it is said to mimic the development of the gill apparatus of fish. Outpouchings of the pharyngeal wall grow as pockets toward the surface, where they are met or at least approached by corresponding infoldings of the ectodermal surface. Normally these outpouchings and infoldings neither meet nor coalesce to form gill slits or fistulas. The supporting tissue on both sides of the pouches is endowed with a cartilaginous supporting bar, a nerve, and a blood vessel, respectively known as the branchial arch (pharyngeal) cartilage, branchial arch nerve, and branchial arch artery. The first such cartilaginous bar is Meckel’s cartilage, in front of the first pharyngeal pouch; the second, Reichert’s cartilage, lies between the first and second pouches. The pharynx is supported by six arch complexes, surrounding and intervening between the pharyngeal pouches. The arteries of these arches are the connectives from the ventral aorta to the dorsal aortas, and they appear in sequence from cranial to caudal. Rarely are more than three such arch arteries identifiable at one time; in this case, as elsewhere in the embryo, the cranial development leads or precedes that occurring more caudally. As the fourth arch artery appears, the first is being transformed into its successor structures and ceases to be identifiable as an arch artery. In humans, there are five such arch arteries, numbered 1, 2, 3, 4, and 6, in recognition of the dropping out in phylogeny of the fifth arch artery, which has no significant role in human development (the fifth pharyngeal pouch fuses with the fourth at its opening into the pharynx; its rudimentary arch between the fourth and fifth pouches contributes to the formation of the larynx). In contrast to the constancy of innervation of the derivatives of the pharyngeal arches, the vascular supply to the arches is subject to later, often extensive modification. The motor nerve to an arch persists throughout phylogeny and throughout ontogenetic development in supplying the derivatives of that arch (first arch, mandibular nerve; second arch, facial nerve; third arch, glossopharyngeal nerve; fourth through sixth arches, recurrent and superior laryngeal nerves and vagal pharyngeal nerve). The geometric representation of the arch artery pattern and the fate of those arteries are summarized in Figure 2-2. The paired dorsal aortas sweep posteriorly and fuse in the midline to form a single dorsal aorta (see Figure 2-2, inset) posterior to entry points of the arch arteries.
The lungs begin their development as a ventrally directed outgrowth from the pharynx, and the single tube that will become the trachea descends into the presumptive chest cavity, where it branches into a pair of lung buds. These buds receive a small blood supply from branches of the sixth aortic arch arteries (see Figure 2-2A). Clearly the sixth arch arteries will have a role in the development of the pulmonary arterial tree. The developmental problem posed here is that the sixth arch arteries are initially part of the systemic circulation, simply representing the most caudal of the branchial arch arteries springing from the truncus arteriosus and uniting with the dorsal aortas. In the division of the heart tube into right and left hearts, some provision must be made for joining the right ventricular outflow tract to the sixth arch arteries and joining the remainder of the great branchial arch system and aortas with the left ventricle. The rationale for fusion of the primitive heart tubes into a single channel and subsequent division is now clarified by this need to divide the bulbus cordis and truncus arteriosus into a pulmonary artery and an aortic artery. The manner of that division solves the problem of connecting the right ventricle and the developing pulmonary artery to the lungs and connecting the remainder of the arch arteries to the systemic circulation and the left ventricle. The interested reader is encouraged to examine the article by Congdon2 for further clarification of this point.
The heart is divided into four chambers that compose two separate hearts, with provision for a parallel mode of function before birth and a tandem mode after birth. The umbilical veins (after the sixth week, a single left umbilical vein) return blood to the fetal heart by their union with the inferior vena cava. This return route sees the umbilical vein enter the liver, where a shunt, the ductus venosus, bypasses the complex hepatic circulation and shunts the blood directly into the inferior vena cava. Thus, the right atrium receives a supply of freshly oxygenated blood, in contrast to the adult condition. Before separation of the right and left atria, that placental return is into the single atrial chamber, which is diagrammatically depicted in Figure 2-3A. The single chamber undergoes a constriction in the plane of the atrioventricular orifices and the atrioventricular sulcus on the exterior of the heart. From the margins of this constriction, endocardial cushions grow inward to begin the formation of the tricuspid and mitral valves. The single atrium begins its separation into halves by downgrowth from the dorsocranial wall of a filmy crescentic curtain—the septum primum (see Figure 2-3B). The leading invaginated edge of the crescent grows down toward the floor of the single atrium; that floor forms by virtue of the growth of the atrioventricular valve primordia. Figure 2-3B shows the septum primum from the right side as it progresses toward complete closure of the single atrial chamber in its midline. In addition, just before the foramen primum closes, a group of perforations forms in the dorsocranial part of the partition (see Figure 2-3B) and then coalesces into a foramen secundum (see Figure 2-3C). This process is necessary because throughout this developmental sequence, the heart is pumping blood to and returning it from the placenta, and the returning blood must be shunted from the right side of the heart into the left atrium in large volume to sustain the systemic circulation. Therefore at no point in fetal life may the right and left atria be functionally separate. During the time that the placental circulation is intact, the pressure in the right atrium exceeds that in the left atrium, and a right-to-left shunt will be operative. As a result, the foramen secundum opens just in time to continue that shunt as the foramen primum closes. Next, on the right side of the septum primum, a much more robust and rigid septum secundum begins its downgrowth, following the same pattern as that of the septum primum (see Figure 2-3C); a crescent-shaped leading edge grows down from above toward the endocardial cushions that will finally separate the atria from the ventricles. This downgrowth of the septum secundum comes to overlie the orifice of the foramen secundum. Fortunately, the septum secundum is sturdy and relatively unyielding, whereas the septum primum is thin and curtainlike. As long as the free lower edge of the septum secundum fails to reach the floor of the atrium, thus forming the foramen ovale, the elevated pressure in the right atrium pushes blood through the ovale, deflecting the septum primum and allowing blood to pass through the foramen secundum into the left atrium and permitting continuation of the obligatory right-to-left shunt. Inasmuch as the downgrowth of the septum secundum is arrested, leaving a fixed foramen ovale, such a shunt operates throughout the intrauterine life of the organism. The orifice of the foramen ovale is just above and medial to the orifice of the inferior vena cava (see Figure 2-3D), so that inferior caval (i.e., placental) blood is preferentially directed into that foramen, and then into the left atrium, with remarkably little mixing of this oxygenated blood with the oxygen-poor blood returning via the superior vena cava.
< div class='tao-gold-member'>
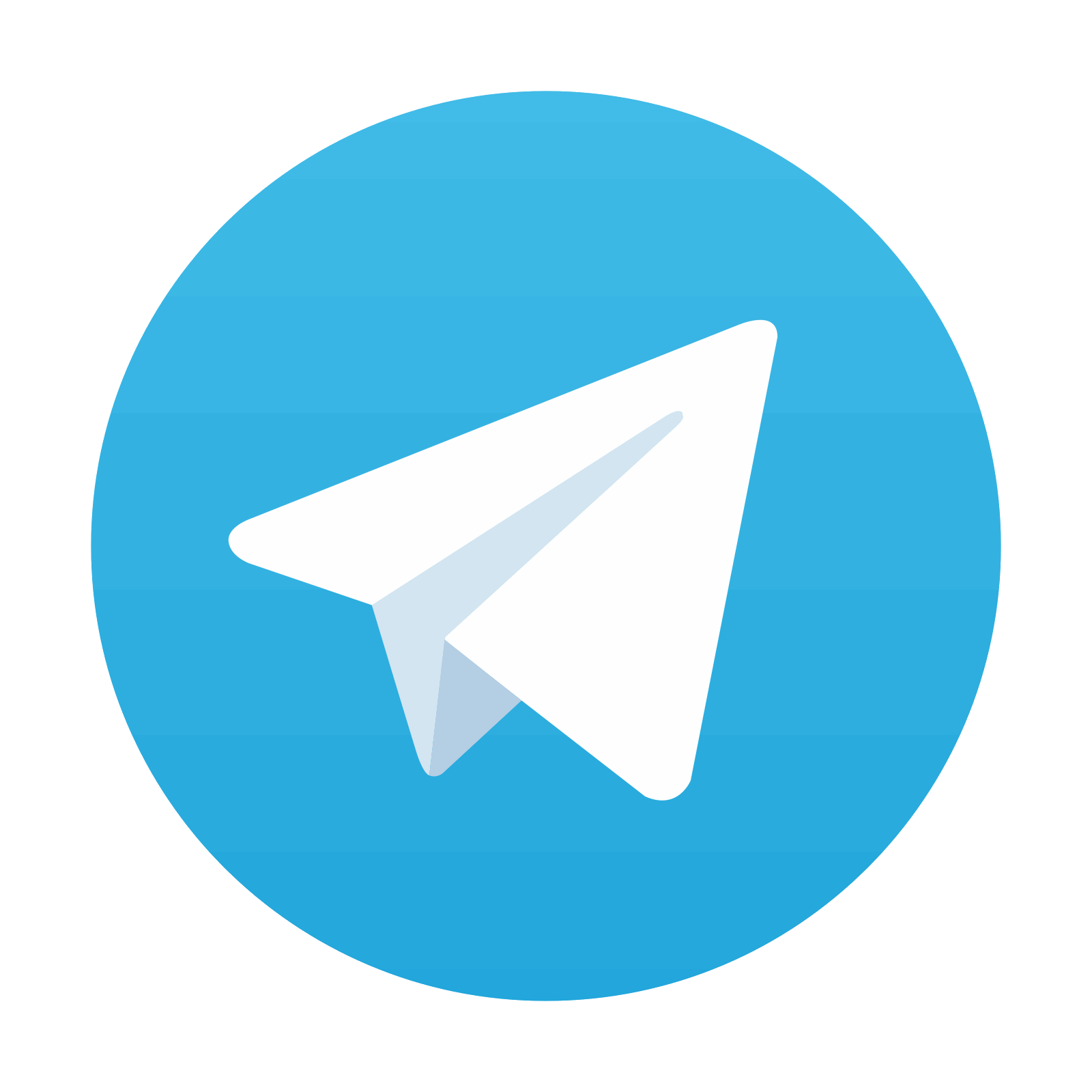
Stay updated, free articles. Join our Telegram channel

Full access? Get Clinical Tree
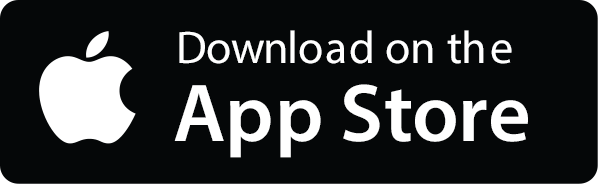
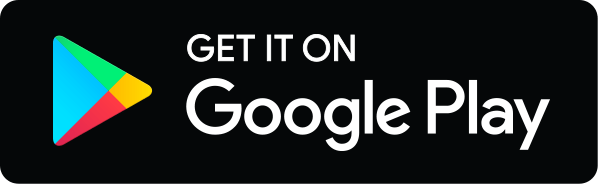