Electrophysiologic Investigation: Technical Aspects
Personnel
The most important aspects for the performance of safe and valuable electrophysiologic studies are the presence and participation of dedicated personnel. The minimum personnel requirements for such studies include at least one physician, one or two nurses (two nurses for complex ablations requiring conscious sedation), a technician with radiation expertise, an anesthesiologist on standby, and an engineer on the premises to repair equipment. With the widespread use of catheter ablation, appropriate facilities and technical support are even more critical.1,2 The most important person involved in such studies is the physician responsible for the performance and interpretation of these studies. This person should have been fully trained in clinical cardiac electrophysiology in an approved electrophysiology training program. The guidelines for training in clinical cardiac electrophysiology have undergone remarkable changes as interventional electrophysiology has assumed a more important role. The current training guidelines for competency in cardiac electrophysiology have been developed by the American College of Cardiology and the American Heart Association, and the American College of Physicians-American Society of Internal Medicine in collaboration with the Heart Rhythm Society (formerly, the North American Society for Pacing and Electrophysiology).3,4 Based on these recommendations, criteria for certification in the subspecialty of clinical cardiac electrophysiology have been established by the American Board of Internal Medicine. Certifying exams are now given twice a year. Recertification is required every 10 years. The clinical electrophysiologist should have electrophysiology in general and arrhythmias in particular as his or her primary commitment. As such, they should have spent a minimum of 1 year, preferably 2 years, of training in an active electrophysiology laboratory and have met criteria for certification. The widespread practice of device implantation by electrophysiologists will certainly make a combined pacing and electrophysiology program mandatory for implanters. This should be a 2-year program. Recently, with the development of resynchronization therapy for heart failure, there has been an interest in developing a program to train heart failure physicians to implant devices in their patients. At the least this should be a program of 1 year, and in my opinion, should include training in basic electrophysiology. Such programs are currently available in a few centers. Sufficient training is necessary for credentialing, which will be extremely important for practice and reimbursement in the future.
One or, preferably, two nurses and a technician are the bare minimum support for simple EP studies and devices. Complex ablations (AF, VT, etc.) should be supported by two nurses and a technician. This is critical for safety, particularly with use of conscious sedation or anesthesia in patients in whom there is risk of life-threatening complications. These nurse–technicians must be familiar with all the equipment used in the laboratory and must be well trained and experienced in the area of cardiopulmonary resuscitation. We use two or three dedicated nurses and a technician in each of our electrophysiology laboratories. Their responsibilities range from monitoring hemodynamics and rhythms, using the defibrillator/cardioverter when necessary, and delivering antiarrhythmic medications and conscious sedation (nurses), to collecting and measuring data online during the study. They are also trained to treat any complications that could possibly arise during the study. An important but often unstressed role is the relationship of the nurse and the patient. The nurse is the main liaison between the patient and physician during the study—both verbally, communicating symptoms, and physically, obtaining physiologic data about the patient’s clinical status. The nurse–technician may also play an invaluable role in carrying out laboratory-based research. It is essential that the electrophysiologist and nurse–technician function as a team, with full knowledge of the purpose and potential complications of each study being ensured at the outset of the study. A radiation technologist should also be available to assure proper equipment function and monitor radiation dose received by patients and laboratory personnel.
An anesthesiologist and probably a cardiac surgeon should be available on call in the event that life-threatening arrhythmias or complications requiring intubation, ventilation, thoracotomy, and potential surgery should arise. This is important in patients undergoing stimulation and mapping studies for malignant ventricular arrhythmias and, in particular, catheter ablation techniques (see Chapter 14). In addition, an anesthesiologist or nurse-anesthetist usually provides anesthesia support for ICD implantation and/or testing. We use anesthesia for all our atrial fibrillation ablations, and for ablative procedures in patients with fragile hemodynamics to
enable us to maintain smooth hemodynamic control during the procedure. Anesthesia is also extremely useful in elderly patients because of the frequent paradoxical response to standard sedation. Although conscious sedation is usually given by laboratory staff, in the substantial minority of laboratories, anesthesia (e.g., propofol) is given by the laboratory staff (nurse or physician) and not by an anesthesiologist.
enable us to maintain smooth hemodynamic control during the procedure. Anesthesia is also extremely useful in elderly patients because of the frequent paradoxical response to standard sedation. Although conscious sedation is usually given by laboratory staff, in the substantial minority of laboratories, anesthesia (e.g., propofol) is given by the laboratory staff (nurse or physician) and not by an anesthesiologist.
A biomedical engineer and/or technician should be available to the laboratory to maintain equipment so that it is properly functioning and electrically safe. It cannot be stated too strongly that electrophysiologic studies must be done by personnel who are properly trained in and who are dedicated to the diagnosis and management of arrhythmias. This opinion is shared by the appropriate associations of internal medicine and cardiology.1,2,3,4 Finally a radiation technologist should be available to assure that excessive radiation is not delivered to the patient or electrophysiology team.
Equipment
The appropriate selection of tools is of major importance to the clinical electrophysiologist. Although expensive and elaborate equipment cannot substitute for an experienced and careful operator, the use of inadequate equipment may prevent the maximal amount of data from being collected, and it may be hazardous to the patient. To some degree, the type of data collected determines what equipment is required. If the only data to be collected involve atrioventricular (A-V) conduction intervals (an extremely rare situation), this can be determined with a single catheter and a simple ECG-type amplifier and recorder, which are available in most cardiology units. However, a complete evaluation of most supraventricular arrhythmias, which may require activation mapping, necessarily involves the use of multiple catheters and several recording channels as well as a programmable stimulator. Thus, an appropriately equipped laboratory should provide all the equipment necessary for the most detailed study. In the most optimal of situations, a room should be dedicated for electrophysiologic studies. This is not always possible, and in many institutions, the electrophysiologic studies are carried out in the cardiac hemodynamic–angiographic catheterization laboratory. A volume of more than 100 cases per year probably requires a dedicated laboratory. The room should have air-filtering equivalent to a surgical operating room, if it is used for ICD and pacemaker implantation. This is the current practice in more than 90% of centers and is likely to be the universal practice in the future. It is important that the electrophysiology laboratory have appropriate radiographic equipment. The laboratory must have an image intensifier that is equipped for at least fluoroscopy, and, in certain instances, is capable of cine-fluoroscopy if the laboratory is also used for coronary angiography. To reduce radiation exposure, pulsed fluoroscopy or other radiation reduction adaptations are required. This has become critical in the ablation era, when radiation exposure can be prolonged and risk of malignancy increased. Currently the best systems are pulsed and digitally based, which reduces the radiation risk and allow for easy storage of acquired data. The equipment must be capable of obtaining views in multiple planes. Newer systems which markedly reduce radiation exposure enable the electrophysiologist to move catheters at a distance or in the absence of the fluoroscopic system. Examples of such systems are the Stereotaxis magnetic guided catheter positioning system and Hanson robotic system. The Stereotaxis system is available at this time; it is expensive and requires special catheters, which add to the expense. The Hanson system is currently not approved in the US, but is likely to be within the year. It is less expensive but all catheters can be used. These systems are of most value for complex ablations (e.g., atrial fibrillation or untolerated ventricular tachycardia), but seem excessive for most procedures. Other navigation systems are also being developed with the goal of reproducible three-dimensional navigation and reduction of fluoroscopy time and exposure. Currently, state-of-the-art equipment for the gamut of electrophysiologic studies includes permanent radiographic equipment of the C-arm, U-arm, or biplane varieties. It is critical that dosimetry to the patient is monitored. Guidelines for total dosage delivered during a single procedure are needed to prevent radiation-induced injury should be mandated.
Electrode Catheters
A variety of catheters is currently available with at least two ring electrodes that can be used for bipolar stimulation and/or recording. The catheter construction may be of the woven Dacron variety or of the newer extruded synthetic materials such as polyurethane. As a general all-purpose catheter, we prefer the woven Dacron catheters (Bard Electrophysiology, Billerica, MA) because of their greater durability and physical properties. These catheters come with a variable number of electrodes, electrode spacing, and curves to provide a range of options for different purposes (Fig. 1-1). Although they have superior torque characteristics, their greatest advantage is that they are stiff enough to maintain a shape and yet they soften at body temperature so that they are not too stiff for forming loops and bends in the vascular system to adapt a variety of uses. The catheters made of synthetic materials cannot be manipulated and change shapes within the body, so they are less desirable. Many companies make catheters for specific uses such as coronary sinus cannulation, His bundle recording, etc., but in most cases I believe this is both costly and unnecessary. The advantages of the synthetic catheters are that they are cheaper and can be made smaller (2 to 3 French) than the woven Dacron types. Currently, most electrode catheters are size 3 to size 8 French. The smaller sizes are used in children. In adult patients, sizes 5 to 7 French catheters are routinely used. Other diagnostic catheters have a deflectable tip (Fig. 1-2). These are useful to reach and record from specific sites (e.g., coronary sinus, crista terminalis, tricuspid valve). In most instances the standard woven Dacron catheters suffice, and they are significantly cheaper. Although special catheters
are useful for specific indications described below, standard catheters can be used for most standard pacing and stimulation protocols. We save our hospital thousands of dollars by using standard woven Dacron catheters for all but the ablation catheter. Skilled catheterizers rarely require steerable catheters for positioning, the cost of which is often >$500 in excess of standard catheters.
are useful for specific indications described below, standard catheters can be used for most standard pacing and stimulation protocols. We save our hospital thousands of dollars by using standard woven Dacron catheters for all but the ablation catheter. Skilled catheterizers rarely require steerable catheters for positioning, the cost of which is often >$500 in excess of standard catheters.
![]() FIGURE 1-1 Electrode catheters routinely used. Woven Dacron catheters with varying number of electrodes and interelectrode distances. |
![]() FIGURE 1-2 Electrode catheter with deflectable tips. Different types of catheters with deflectable tips. These are primarily made of extruded plastic. |
Electrode catheters have been designed for special uses. Catheters with an end hole and a lumen for pressure measurements may be useful in: (a) electrophysiologic hemodynamic diagnostic studies for Ebstein’s anomaly; (b) validation of a His bundle potential by recording that potential and the right atrial pressure simultaneously (see Chapter 2); (c) the occasional instance when it may be desirable to pass the catheter over a long guidewire or transseptal needle; and (d) electrophysiologic studies that are part of a more general diagnostic study and/or for which blood sampling from a specific site (e.g., the coronary sinus) or angiography in addition to pacing is desirable. Special catheters have also been designed to record a sinus node electrogram, although we believe that such electrograms can be obtained using standard catheters (see Chapter 3). Other catheters have been specially designed to facilitate recording of the His bundle potential using the antecubital approach, which occasionally may be useful when the standard femoral route is contraindicated. This catheter has a deflectable tip that permits it to be formed into a pronounced J-shape once it has been passed into the right atrium.
In the last decade the evolution of ablation techniques for a variety of arrhythmias necessitated the development of catheters that enhance the ability to map as well as to safely deliver radiofrequency energy. Mapping catheters fall into two general categories: (a) deflectable catheters to facilitate positioning for mapping and delivering ablative energy and (b) catheters with multiple poles (8 to 64) that allow for simultaneous acquisition of multiple activation points. The former category includes a variety of ablation catheters as well as catheters to record and pace from specific regions (e.g., coronary sinus, tricuspid annulus, slow pathway [see Chapter 8], crista terminalis [see Chapter 9]). Some ablation catheters have a cooled tip, one through which saline is infused to allow for enhanced tissue heating without superficial charring (Biosense Webster and St Jude) or internal cooling (Chili; Boston Scientific) (Fig. 1-3). Ablation catheters deliver RF energy through tips that are typically 3.5 to 5 mm in length but may be as long as 10 mm. Catheters that are capable of producing linear radiofrequency lesions are being developed to treat atrial fibrillation by compartmentalizing the atria, but currently the ability of these catheters to produce transmural linear lesions that have clinical benefit and are safe is not proven. Catheters that deliver microwave, laser, cryothermal, or pulsed-ultrasound energy to destroy tissue are currently under active investigation. The cryothermal catheters have recently been approved by the FDA for A-V nodal modification for A-V nodal tachycardia (see Chapter 14) but are also being evaluated for other uses, that is, atrial fibrillation. For this latter use a cryoballoon catheter has been developed (Arctic; Medtronic [Fig. 1-4]). In the second category are included standard catheters with up to 24 poles that can be deflected to map large and/or specific areas of the atrium (e.g., coronary sinus, tricuspid annulus, etc.) (Fig. 1-5). Of particular note are catheters shaped in the
form of a “halo” to record from around the tricuspid ring (Fig. 1-6), or a lasso catheter on a deflectable shaft to record from 10 to 20 electrodes in the pulmonary vein/ostia, (Biosense Webster and St Jude) (Fig. 1-7) and basket catheters (Fig. 1-8), which have up to 64 poles or prongs that spring open and which are used to acquire simultaneous data
from within a given cardiac chamber. A PentaRay catheter (Fig. 1-9) is available from Biosense Webster which has five flexible splines with 4 electrodes on each spline allowing on to acquire 20 sites of activation. The 2-mm interelectrode distance allows for high-density mapping. The floppiness of
the splines sometimes makes for variable contact in misinterpretation of data. More recently Rhythmia Medical (Boston Scientific) has developed a 64 pole roving catheter (Fig. 1-10). This mapping (minibasket) catheter has an 8 F bidirectional deflectable shaft and a basket electrode array (usual mapping diameter 18 mm) with eight splines, each spline containing eight small (0.4 mm2), low-impedance electrodes (total 64 electrodes). The interelectrode spacing along the spline is 2.5 mm (center-to-center). Mapping can be performed with the basket in variable degrees of deployment (diameter ranging 3 to 22 mm). The location of each of the 64 electrodes is identified by a combination of a magnetic sensor in the distal region of the catheter and impedance sensing on each of the 64 basket electrodes. The location of each basket electrode is obtained whether the basket is fully or only partially deployed. Heparinized saline (1 U/mL) is infused through the central lumen of the catheter shaft at 1 mL/min, emerging at the proximal end of the basket to prevent thrombus. This
catheter has a magnetic sensor and with its respiratory gating in the accompanying mapping system provides the most accurate high-density mapping currently available. The magnetic locating system is similar to Carto, Biosense Webster (see below).
form of a “halo” to record from around the tricuspid ring (Fig. 1-6), or a lasso catheter on a deflectable shaft to record from 10 to 20 electrodes in the pulmonary vein/ostia, (Biosense Webster and St Jude) (Fig. 1-7) and basket catheters (Fig. 1-8), which have up to 64 poles or prongs that spring open and which are used to acquire simultaneous data
from within a given cardiac chamber. A PentaRay catheter (Fig. 1-9) is available from Biosense Webster which has five flexible splines with 4 electrodes on each spline allowing on to acquire 20 sites of activation. The 2-mm interelectrode distance allows for high-density mapping. The floppiness of
the splines sometimes makes for variable contact in misinterpretation of data. More recently Rhythmia Medical (Boston Scientific) has developed a 64 pole roving catheter (Fig. 1-10). This mapping (minibasket) catheter has an 8 F bidirectional deflectable shaft and a basket electrode array (usual mapping diameter 18 mm) with eight splines, each spline containing eight small (0.4 mm2), low-impedance electrodes (total 64 electrodes). The interelectrode spacing along the spline is 2.5 mm (center-to-center). Mapping can be performed with the basket in variable degrees of deployment (diameter ranging 3 to 22 mm). The location of each of the 64 electrodes is identified by a combination of a magnetic sensor in the distal region of the catheter and impedance sensing on each of the 64 basket electrodes. The location of each basket electrode is obtained whether the basket is fully or only partially deployed. Heparinized saline (1 U/mL) is infused through the central lumen of the catheter shaft at 1 mL/min, emerging at the proximal end of the basket to prevent thrombus. This
catheter has a magnetic sensor and with its respiratory gating in the accompanying mapping system provides the most accurate high-density mapping currently available. The magnetic locating system is similar to Carto, Biosense Webster (see below).
![]() FIGURE 1-9 PentaRay mapping catheter. This flexible, 20 pole catheter on 5 splines allows for high-density activation mapping. |
Another catheter that has the characteristics and appearance of a standard ablation catheter that has a magnetic sensor within the shaft near the tip is made by Biosense, Webster (see Fig. 1-3). Together with a reference sensor, it can be used to precisely map the position of the catheter in three dimensions. This Biosense electrical and anatomic mapping system is composed of the reference and catheter sensor, an external, ultra-low magnetic field emitter, and the processing unit.5 The amplitude, frequency, and phase of the sensed magnetic fields contain information required to solve the algebraic equations yielding the precise location in three dimensions (x, y, and z axes) and orientation (roll, yaw, pitch) of the catheter tip sensor. A unipolar or bipolar electrogram can be recorded simultaneously with the position in space. An electrical anatomic map can, therefore, be generated. This provides precise (∼1 mm) accuracy and allows one to move the catheter back to any desirable position, a particularly important feature in mapping. In addition, the catheter may be moved in the absence of fluoroscopy, thereby saving unnecessary radiation exposure. The catheter, because of its ability to map the virtual anatomy, can display the cardiac dimensions, volume, and ejection fraction. New enhancements include respiratory gating, assessment of catheter stability prior to ablation, and measurement of contact force to optimize the ablation lesion. This is similar to the Rhythmia Medical mapping system which records from 64 poles in unipolar and bipolar modes yielding the highest accuracy, but which requires a separate ablation catheter.
Another new mapping methodology, with its own catheter, is Ensite noncontact endocardial mapping system. An intracavitary multielectrode probe (Fig. 1-11) is introduced retrogradely, transseptally, or pervenously into the desired chamber and endocardial electrograms are reconstructed using inverse solution methods.6 Endocardial potentials and activations sequences are reconstructed from intracavitary probe signals by a mathematical process called the “inverse solution.” Beat-to-beat activation sequences of the entire chamber are generated. It represents both the inverse solution for 64 poles from which several thousand signals are interpolated in space. Whether this technique offers enough spatial resolution to be used to guide precise ablation in large, and/or diseased hearts, requires validation.
The number and spacing of ring electrodes on standard, contact mapping catheters may vary. Specially designed catheters with many electrodes (up to 24), an unusual sequence of electrodes, or unusual positioning of bipolar pairs may be useful for specific indications. For routine pacing or recording, a single pair of electrodes is sufficient; simultaneous recording and stimulation require two pairs; and studies requiring detailed evaluation of activation patterns or pacing from multiple sites may require several additional pairs. It is important to realize that while multiple poles can gather simultaneous and accurate data, only the distal pole of an intracavitarily placed electrode will have consistent contact with the wall; thus, electrograms from the proximal electrodes may yield unreliable data. In general, a quadripolar catheter suffices for recording and stimulation of standard sites in the right atrium, right ventricle, and for recording a His bundle electrogram. We routinely use the Bard Electrophysiology multipurpose quadripolar catheter with a 5-mm interelectrode distance for recording and stimulation of the atrium and ventricle as well as for recording His bundle.
![]() FIGURE 1-11 The EnSite noncontact mapping probe. Mathematically derived electrograms from more than 3,000 sites can be generated from this olive-like probe (see Chapter 14). |
The interelectrode distances may range from 1 to 10 mm or more. In studies requiring precise timing of local electrical activity, tighter interelectrode distances are theoretically advantageous. We have evaluated activation times comparing 5- and 10-mm interelectrode distance on the same catheter and have found they do not differ significantly. It is unclear how much different the electrogram timing is using 1- to 2-mm apart electrodes. In my experience, the “local activation time” is similar in normal tissue. The width and amplitude of the electrogram and sometimes additional components of a multicomponent electrogram are more frequently seen with interelectrode distances of ≥5 mm and may be absent when very narrow interelectrode distances are used. This is not surprising since most of the local information recorded is from
the tip electrode. If careful attention is paid to principles of measurement, an accurate assessment of local activation time on a bipolar recording can be obtained with electrodes that are 5 or 10 mm apart. As stated above, we routinely use catheters with a 2-mm or 5-mm interelectrode distance for most general purposes. Very narrow interelectrode distances (less than or equal to 1 mm) may, however, be useful in understanding multicomponent electrograms. In similar fashion, orthogonal electrodes may provide particularly advantageous information regarding the presence of bypass tract potentials. In certain circumstances, unipolar, unfiltered, or filtered recordings are used since they provide the most accurate information regarding local activation time as well as directional information (Fig. 1-12). The peak negative deflection of the unfiltered bipolar signal corresponds to the intrinsicoid deflection of the unfiltered unipolar signal (Fig. 1-13). The filtered unipolar signal times with the most rapid dV/dT of the unfiltered signal and is the best approximate of local activation. Using simultaneous unipolar and bipolar recordings allows one to determine which component of a multicomponent signal is local and what the sequence of activation is (Fig. 1-14).7 To facilitate recording unipolar potentials without electrical interference, catheters have been developed with a fourth or fifth pole, 20 to 50 cm from the tip. This very proximal pole can be used as an indifferent electrode, and unipolar unfiltered recordings can be obtained without electrical interference. We have found this method to be more consistently free of artifact than unipolar signals generated using a Wilson central terminal. If handled with care, electrode catheters, specifically the woven Dacron types without a lumen, may be resterilized and reused almost indefinitely. However, there is much disagreement about the policy of reuse of catheters. Whereas, many of the early electrophysiologists have used the woven Dacron catheters multiple times without infection, there has been some concern in some laboratories about resterilization. While sterilization using ethylene oxide may leave deposits, particularly in extruded catheters, other forms of sterilization are safe. Contrarily, all catheters with lumens must be discarded after a single use. If catheters are reused prior to sterilization, they should be checked to assess electrical continuity. This can be done with a simple application of an ohmmeter to the distal ring and the corresponding proximal connectors. Currently the FDA has proposed strict guidelines for the resterilization of catheters. As a consequence, most institutions now send out their catheters to companies with approved resterilization facilities or, more commonly, have gone to single use.
the tip electrode. If careful attention is paid to principles of measurement, an accurate assessment of local activation time on a bipolar recording can be obtained with electrodes that are 5 or 10 mm apart. As stated above, we routinely use catheters with a 2-mm or 5-mm interelectrode distance for most general purposes. Very narrow interelectrode distances (less than or equal to 1 mm) may, however, be useful in understanding multicomponent electrograms. In similar fashion, orthogonal electrodes may provide particularly advantageous information regarding the presence of bypass tract potentials. In certain circumstances, unipolar, unfiltered, or filtered recordings are used since they provide the most accurate information regarding local activation time as well as directional information (Fig. 1-12). The peak negative deflection of the unfiltered bipolar signal corresponds to the intrinsicoid deflection of the unfiltered unipolar signal (Fig. 1-13). The filtered unipolar signal times with the most rapid dV/dT of the unfiltered signal and is the best approximate of local activation. Using simultaneous unipolar and bipolar recordings allows one to determine which component of a multicomponent signal is local and what the sequence of activation is (Fig. 1-14).7 To facilitate recording unipolar potentials without electrical interference, catheters have been developed with a fourth or fifth pole, 20 to 50 cm from the tip. This very proximal pole can be used as an indifferent electrode, and unipolar unfiltered recordings can be obtained without electrical interference. We have found this method to be more consistently free of artifact than unipolar signals generated using a Wilson central terminal. If handled with care, electrode catheters, specifically the woven Dacron types without a lumen, may be resterilized and reused almost indefinitely. However, there is much disagreement about the policy of reuse of catheters. Whereas, many of the early electrophysiologists have used the woven Dacron catheters multiple times without infection, there has been some concern in some laboratories about resterilization. While sterilization using ethylene oxide may leave deposits, particularly in extruded catheters, other forms of sterilization are safe. Contrarily, all catheters with lumens must be discarded after a single use. If catheters are reused prior to sterilization, they should be checked to assess electrical continuity. This can be done with a simple application of an ohmmeter to the distal ring and the corresponding proximal connectors. Currently the FDA has proposed strict guidelines for the resterilization of catheters. As a consequence, most institutions now send out their catheters to companies with approved resterilization facilities or, more commonly, have gone to single use.
![]() FIGURE 1-12 Unipolar recordings. The unipolar recording identifies local activity by the most rapid negative (intrinsicoid) deflection. |
Laboratory Organization
As stated previously, a dedicated electrophysiologic laboratory and equipment dedicated to that laboratory are preferred. Use of stimulation and recording equipment in such a laboratory is schematically depicted in Figure 1-15. The equipment may be permanently installed in an area set apart for electrophysiologic work, or it may be part of a general catheterization laboratory such that it is installed in a standard rack mount that includes the hemodynamic monitoring amplifiers. In most laboratories, a stimulator and a computer system that modifies all input signals and stores them on optical disk are used. Some centers still use older systems, such as the E for M electronics DR 8, 12, or 16, in which signals are conditioned and visualized on an oscilloscope and printed out on a strip chart recorder. Such data may also be separately saved on tape for subsequent review. These systems, some of which may be more than 20 years old, are no longer commercially available, but work well. The recent development of computerized recording systems with optical disks has obviated the need for a tape recorder or VCR for clinical studies and has made storage of data much easier. However, current proprietary software limits the ability to analyze data acquired on computers with different software. Conversely, research data stored on a VCR tape recorder can be more widely used. In the near future, several manufacturers of recording equipment will allow storage of individual cases on CDs. Currently this is possible on the Bard system. This may allow analysis “off-site,”
although tools for measurements are not readily available on standard laptops. There are several computerized recording systems available; the most commonly used are Marquette (formerly Prucka) and Bard (see below). The quality of the signal in each of the systems varies because of the way they are processed and filtered. I believe the Bard system currently provides purer signals the Marquette but it is less user-friendly. Further refinement should improve both systems. Filtering the signal can remove or add components to the signal which are not the pure signal. Even a notch filter (60 Hz) can introduce artifactual complexity to the signal (Fig. 1-16).7
although tools for measurements are not readily available on standard laptops. There are several computerized recording systems available; the most commonly used are Marquette (formerly Prucka) and Bard (see below). The quality of the signal in each of the systems varies because of the way they are processed and filtered. I believe the Bard system currently provides purer signals the Marquette but it is less user-friendly. Further refinement should improve both systems. Filtering the signal can remove or add components to the signal which are not the pure signal. Even a notch filter (60 Hz) can introduce artifactual complexity to the signal (Fig. 1-16).7
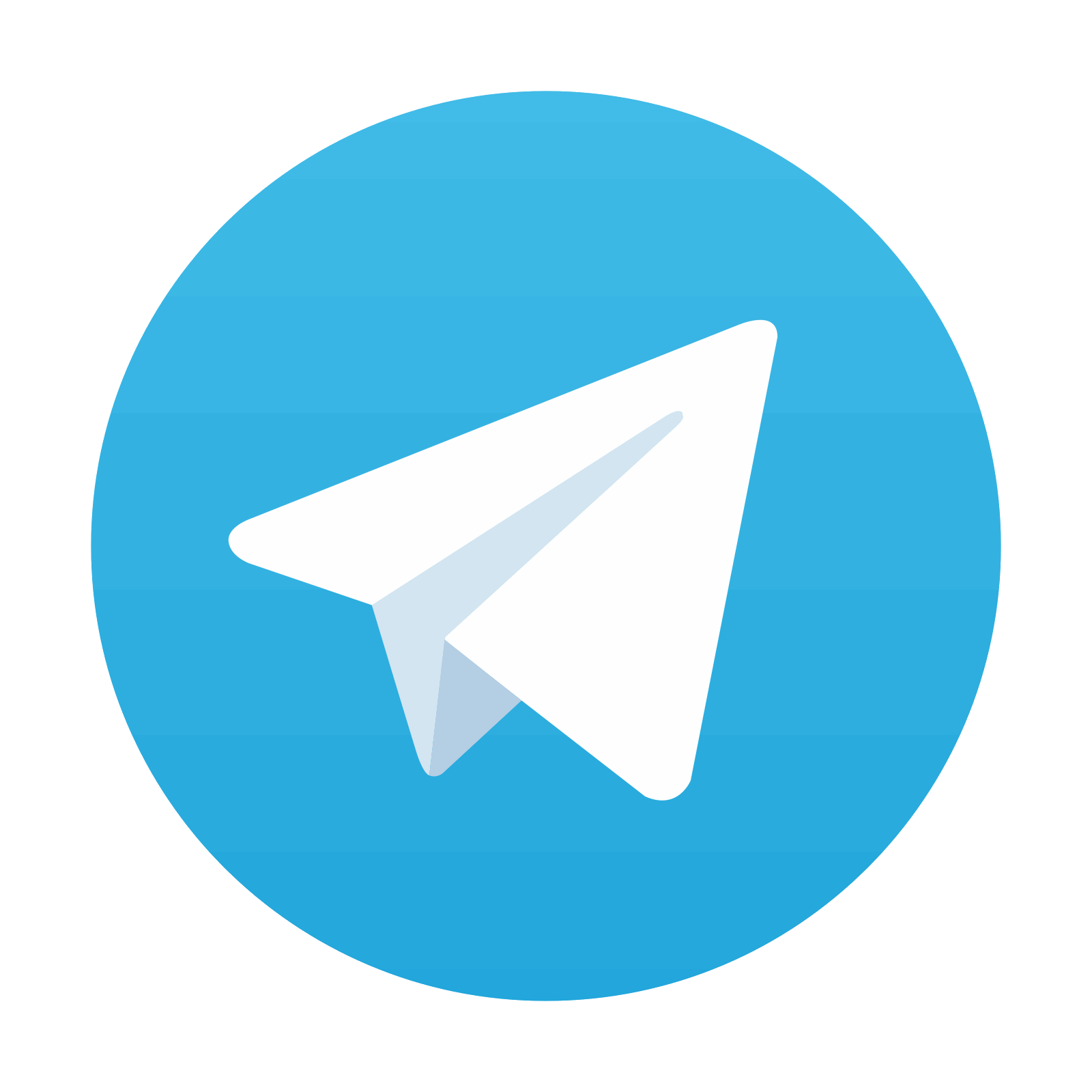
Stay updated, free articles. Join our Telegram channel

Full access? Get Clinical Tree
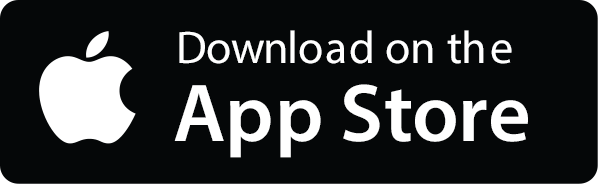
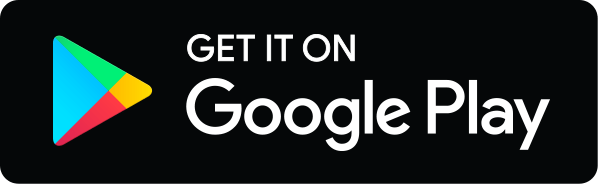