Background
Therapeutic hypothermia is used after cardiac arrest. The aim of this study was to investigate the effects of therapeutic hypothermia on left ventricular (LV) function assessed by ultrasonic imaging.
Methods
In 10 pigs, LV volumes, ejection fractions, and longitudinal strain were measured using two-dimensional echocardiography. Midwall fractional shortening and end-systolic wall stress were calculated. Wall thickness was continuously measured using an epicardial ultrasonic transducer placed on the LV anterior wall. Wall thickening velocity (S′) and pressure–wall thickness loops were used to assess systolic function. Diastolic function was assessed by echocardiographic transmitral flow and mitral annular velocity (e′) measurements, calculation of the LV relaxation constant, and determination of LV stiffness and restoring forces using the end-diastolic pressure–wall thickness relation during volume unloading. Early wall thinning velocity (e′ wt ) and early diastolic wall thinning were calculated. Measurements were done at 38°C and 33°C, at spontaneous heart rate and at atrial pacing at 100 beats/min.
Results
End-diastolic volume, stroke volume, midwall fractional shortening, and longitudinal strain remained unchanged during hypothermia, but end-systolic wall stress, S′, and pressure–wall thickness loop area decreased. A shift from early to late diastolic LV filling occurred during hypothermia, with concurrent decreases in e′, e′ wt , and early wall thinning fraction. Relaxation was prolonged, LV stiffness was increased, and restoring force was decreased during hypothermia. Hypothermia induced a decrease in relative diastolic duration at spontaneous heart rate, which was further reduced during pacing. During paced heart rate at 33°C, stroke volume, ejection fraction, and strain were reduced.
Conclusions
Hypothermia induced systolic and diastolic dysfunction, with reduced tolerance to increased heart rate. These findings may have implications for patient management during hypothermia.
Moderate hypothermia is increasingly used as adjunctive treatment to improve outcomes in comatose survivors of out-of-hospital cardiac arrest. Many patients receiving this treatment are hemodynamically unstable and require inotropic or mechanical circulatory support. The instability in this postresuscitation phase may arise from both regional myocardial infarction preceding the cardiac arrest and global ischemia caused by the arrest and subsequent resuscitation.
However, the hypothermia itself is also known to affect cardiovascular function, but the findings regarding left ventricular (LV) systolic function during hypothermia are ambiguous. Studies have demonstrated an increase in systolic function assessed by dP/dt max , end-systolic pressure-dimension relationship (E max ), or regional work represented by pressure-dimension loop area, whereas other studies have failed to demonstrate any change or even have demonstrated reduced systolic function variables during hypothermia. In contrast, assessment of diastolic function has more uniformly demonstrated a decrease in diastolic LV function by prolonged relaxation and a decrease in peak dP/dt min .
These studies of cardiac function during hypothermia have been performed using pressure-dimension measurements using either conductance catheters or sonomicrometric crystals. However, there is a conspicuous scarcity of studies using clinical imaging techniques to assess effects from hypothermia. Two-dimensional (2D) echocardiography is, in addition to its applications in routine hemodynamic monitoring, the principal modality for bedside assessment of cardiac function in the postresuscitation setting. The aim of this study was therefore, in an open-chest porcine model, to describe changes in myocardial performance during exposure to moderate hypothermia, using 2D ultrasound imaging techniques. To obtain continuous data during serial cardiac cycles, we used a recently validated epicardial ultrasonic system allowing M-mode recordings of high temporal and spatial resolution.
We hypothesized that therapeutic hypothermia would reduce systolic and diastolic function as detected using both conventional echocardiographic and novel ultrasonic techniques.
Methods
Animal Model
The main interventions in this experimental study were temperature change and heart rate (HR) manipulations. Measurements were made at baseline temperature (adjusted to 38°C) and during hypothermia (33°C). Because hypothermia reduces HR, measurements were made at spontaneous HR and at a fixed HR induced by atrial pacing at 100 beats/min, the latter to exclude effects from differences in HR. In each intervention, transient constriction of the inferior caval vein was performed for acute volume unloading. Ten animals were included in the study. In six animals, the recordings were performed at 38°C, followed by cooling to 33°C and repeat recordings. In four animals, cooling was instituted during instrumentation, and hypothermic recordings were made before rewarming and new recordings were made at 38°C.
Preparation
The animals (Norwegian land race swine; mean weight, 52.6 ± 6.4 kg) were anesthetized and tracheotomized as previously described. Muscle-relaxing agents were not used. In case of shivering during cooling, the level of anesthesia was increased by an extra bolus of intravenous morphine. Ventilation with air/oxygen (fraction of inspired oxygen, 0.4) using tidal volumes of 10 to 15 mL/kg was adjusted during hypothermia to maintain a stable partial pressure of carbon dioxide. Left atrial pressure and LV pressure (LVP) were measured using micromanometers (MPC-500; Millar Instruments, Houston, TX). For cooling and rewarming of the animals, a water-circulated catheter (Cool Line; Zoll, Chelmsford, MA) was placed in the inferior vena cava from the left femoral vein and connected to a designated thermal regulation system (Coolgard 3000; Zoll). Central temperature was measured in the pulmonary artery (Swan-Ganz CCO; Edwards Lifesciences, Irvine, CA). After sternotomy, epicardial ultrasonic transducers (Imasonic SA, Besançon, France) were sutured to the anterior LV wall for continuous monitoring of wall thickness through the cardiac cycle as a measure of myocardial function. Two pacemaker leads were attached to the right atrial appendage. A snare was placed around the inferior caval vein for acute volume unloading. All animals were handled according to international regulations. The study was approved by the National Animal Research Authority of Norway.
Measurements and Calculations
Hemodynamics
Peak systolic LVP and end-diastolic LVP (EDP) were recorded. Peak dP/dt max and dP/dt min were recorded. Cardiac output was measured by pulmonary artery catheter thermodilution, by three injections of 10 mL of ice-cold saline. The duration of systole was calculated from the onset of the steep upstroke of dP/dt to dP/dt min . Diastolic duration was calculated from dP/dt min to the onset of systole ( Figure 1 ). The LV relaxation time constant (τ) was calculated during isovolumic relaxation time according to Weiss et al. Time for complete relaxation was defined as 3.5τ from end-systole and was calculated as a fraction of diastolic duration (3.5τ/diastolic duration).

Ultrasonic Imaging
A Vivid 7 scanner with a 2.5/2.75-MHz probe (GE Vingmed Ultrasound AS, Horten, Norway) was used directly on the heart with a gel standoff for 2D echocardiographic and Doppler recordings. LV outflow tract Doppler recording was used for stroke volume calculation. End-systolic and end-diastolic volumes were calculated using Simpson’s biplane method. Ejection fraction was calculated as (end-diastolic volume − end-systolic volume)/end-diastolic volume. Longitudinal strain in the septal and lateral wall middle segments was calculated using speckle-tracking on 2D grayscale images (frame rate, 63 ± 11 frames/sec). Midwall fractional shortening (FS) was calculated according to de Simone et al. Transmitral flow was measured from Doppler recordings and the velocity-time integral (VTI) was calculated. The transmitral E and A waves fused during pacing at 33°C, so the fraction of VTI occurring before the P wave on the electrocardiogram was used as a measure of early LV filling. Early diastolic LV lengthening velocity (e′) was measured from Doppler tissue imaging recordings of mitral annular velocity from the longitudinal four-chamber projection. The E/e′ ratio was calculated.
For continuous recordings, wall thickness was measured through the cardiac cycle by M-mode recordings from the epicardial ultrasonic transducers ( Figure 1 ). End-systolic and end-diastolic wall dimensions were recorded. The velocity of wall thickening was measured as previously described. Peak systolic wall thickening velocity (S′) and early (e′) and late (a′) diastolic wall thinning velocities were recorded. During paced HRs at 33°C, the e′ and a′ waves fused in all experiments, precluding analyses of these variables. As an alternative measure of early diastolic function, we calculated early diastolic wall thinning fraction, defined as the diastolic wall thinning that occurred before the succeeding P wave on the electrocardiogram as a fraction of total diastolic wall thinning ( Figure 1 ). The major part of the diastolic filling normally occurs early, so the early diastolic wall thinning fraction would expectedly be high in a normal baseline situation. Early wall thinning fraction could be analyzed through all interventions.
End-systolic wall stress was calculated as (LVP × [LV inner diameter]/2)/ 2 h (1 + h /[LV inner diameter]), where h is the averaged wall thickness value of the anterior and posterior wall thicknesses. Wall thickness through the cardiac cycle was plotted toward LVP, resulting in pressure–wall thickness (P-WTh) loops used for further analysis. LV stiffness was assessed using end-diastolic PWTh relations during caval occlusion ( Figure 2 ), and the coefficient of the corresponding fit line ( K LV ) was calculated as LV stiffness. Restoring forces express the recoil of the systolic compression of the myocardium and have been demonstrated to be an independent contributor to early diastolic LV lengthening velocity. We calculated the restoring forces by similar principles as used by Opdahl et al. , although we used the maximum wall thickness instead of maximum segment shortening as the expression of systolic compression. The P-WTh relations were plotted during caval constriction to obtain the wall thickness at zero EDP. The unstressed wall thickness was subtracted from the end-systolic wall thickness in a normal cycle ( Figure 2 ), the difference representing the restoring forces.

Statistical Analysis
Data are reported as mean ± SD. The data from 38°C and 33°C at spontaneous and paced HRs were compared using paired t tests. To study the effects of the interventions, the data were analyzed using a linear mixed model to handle the dependencies introduced by repeated measurements within in each subject. The data were analyzed using temperature level and HR (spontaneous or with atrial pacing) as determinants in a model in which variable = β + β 1 (temperature) + β 2 (HR) + β 3 (temperature × HR). Time of recording was included as a covariate to account for the order of measurements and assess the effect of time. Determinants for early diastolic filling (relaxation rate, restoring force, and K LV ) were assessed in a model in which early diastolic wall thinning = β + β 1 τ + β 2 (maximum wall thickness − unstressed wall thickness) + β 3 K LV . Model selection was made choosing the covariance structure with the lowest Akaike information criterion. Predicted values and residuals were inspected for goodness of model fit. Differences were considered significant at P ≤ .05.
Results
Recordings of good quality were obtained from all experiments, except from caval constriction recordings in two animals. Two animals had spontaneous HRs of 100 beats/min at 38°C and hence were omitted from comparison between spontaneous and paced HRs at 38°C. The preparation of the animals lasted 228 ± 30 min, including a stabilizing period of 30 to 45 min before measurements. The cooling lasted 95 ± 31 min, while warming in the “cold first” animals lasted 218 ± 39 min. The average time for each experiment was 546 ± 22 min. The effects from temperature and HR changes were not dependent on the time of recording or the order of measurements in this model. Representative epicardial recordings from the two temperature levels during spontaneous and paced HRs are shown in Figure 3 .
LV Function
During hypothermia, HR decreased, with concomitant reductions in peak LVP and the amplitudes of dP/dt max and dP/dt min ( Table 1 ). Atrial pacing did not change LVP dP/dt max or dP/dt min during normothermia or hypothermia. Cardiac output was reduced during hypothermia and increased by pacing at 33°C.
Variable | Spontaneous HR | Atrial pacing (100 beats/min) | ||||
---|---|---|---|---|---|---|
38°C | 33°C | P | 38°C | 33°C | P | |
HR (beats/min) | 89 ± 10 | 77 ± 7 | .04 | 100 ± 0 | 100 ± 0 | 1.00 |
Cardiac output (L/min) | 4.9 ± 0.9 | 3.7 ± 0.8 | .00 | 5.2 ± 0.7 | 4.1 ± 0.7 † | .001 |
Systolic duration (msec) | 346 ± 27 | 470 ± 41 | <.001 | 347 ± 70 | 430 ± 59 ∗ | <.001 |
Diastolic duration (msec) | 343 ± 80 | 334 ± 87 | .60 | 276 ± 16 ∗ | 193 ± 21 † | <.001 |
τ (msec) | 32 ± 4 | 60 ± 10 | <.001 | 31 ± 5 | 54 ± 8 | <.001 |
3.5τ/diastolic duration | 0.34 ± 0.09 | 0.69 ± 0.32 | .002 | 0.41 ± 0.07 ∗ | 1.0 ± 0.17 † | .001 |
LVP (mm Hg) | 84 ± 7 | 69 ± 11 | <.001 | 85 ± 7 | 69 ± 9 | <.001 |
EDP (mm Hg) | 10 ± 3 | 12 ± 2 | .02 | 10 ± 2 | 13 ± 3 | .05 |
LV dP/dt max (mm Hg/sec) | 1,256 ± 285 | 1,010 ± 247 | <.001 | 1,284 ± 232 | 1,060 ± 307 | .005 |
LV dP/dt min (mm Hg/sec) | −1,216 ± 220 | −647 ± 266 | .004 | −1,252 ± 215 | −690 ± 185 | <.001 |
∗ P < .05 for comparison within temperature level.
Systole
End-diastolic volume, stroke volume, and ejection fraction remained unchanged compared with normothermia. Similarly, longitudinal speckle-tracking strain remained unchanged during hypothermia, but time to peak strain increased ( Figure 4 ). Systolic wall thickening velocity and P-WTh loop area, however, decreased during hypothermia ( Figure 5 ). There occurred no significant changes in end-diastolic and end-systolic wall dimensions during hypothermia at spontaneous HR ( Table 2 ), but end-systolic wall stress was reduced because of the lower LVP.
Variable | Spontaneous HR | Atrial pacing (100 beats/min) | ||||
---|---|---|---|---|---|---|
38°C | 33°C | P | 38°C | 33°C | P | |
2D echocardiography | ||||||
End-diastolic volume (mL) | 81 ± 6 | 73 ± 10 | .14 | 77 ± 7 | 64 ± 9 ∗ | .013 |
Stroke volume (mL) | 55 ± 10 | 53 ± 8 | .69 | 55 ± 7 | 46 ± 3 † | .002 |
Midwall FS (%) | 16 ± 4 | 17 ± 4 | .29 | 17 ± 5 | 13 ± 4 † | .02 |
Longitudinal strain (%) | −18.6 ± 2.0 | −16.9 ± 2.9 | .18 | −17.1 ± 1.4 | −14.1 ± 2,0 ∗ | .06 |
End-systolic stress (kdyne/cm 2 ) | 33.6 ± 4.9 | 27.9 ± 7.7 | .01 | 35.1 ± 6.9 | 28.4 ± 8.6 | .02 |
E (cm/sec) | 0.7 ± 0.1 | 0.5 ± 0.1 | .01 | |||
A (cm/sec) | 0.5 ± 0.1 | 0.6 ± 0.1 | .03 | |||
Doppler tissue imaging e′ (cm/s) | 8.1 ± 2.2 | 5.8 ± 1.0 | .004 | |||
E/e′ ratio | 8.6 ± 2.4 | 9.8 ± 2.4 | .056 | |||
M-mode | ||||||
End-diastolic wall thickness (mm) | 11.2 ± 2.0 | 11.7 ± 1.9 | .29 | 11.4 ± 0.9 | 12.3 ± 0.9 ∗ | .01 |
End-systolic wall thickness (mm) | 14.8 ± 2.4 | 15.6 ± 1.5 | .81 | 15.0 ± 0.9 | 15.6 ± 0.6 | .15 |
S′ wt (cm/sec) | 1.1 ± 0.3 | 0.8 ± 0.2 | <.001 | 1.1 ± 0.3 | 0.7 ± 0.2 | <.001 |
e′ wt (cm/sec) | −2.5 ± 0.9 | −1.6 ± 0.7 | .002 | |||
a′ wt (cm/sec) | −1.2 ± 0.4 | −1.4 ± 0.3 | .39 | |||
Early wall thinning fraction | 0.81 ± 0.05 | 0.12 ± 0.11 | <.001 | 0.71 ± 0.04 † | 0.18 ± 0.09 ∗ | <.001 |
Restoring force (mm) | 0.1 ± 1.2 | −1.1 ± 1.4 | <.001 | −0.7 ± 0.8 | −2.1 ± 1.9 ∗ | .07 |
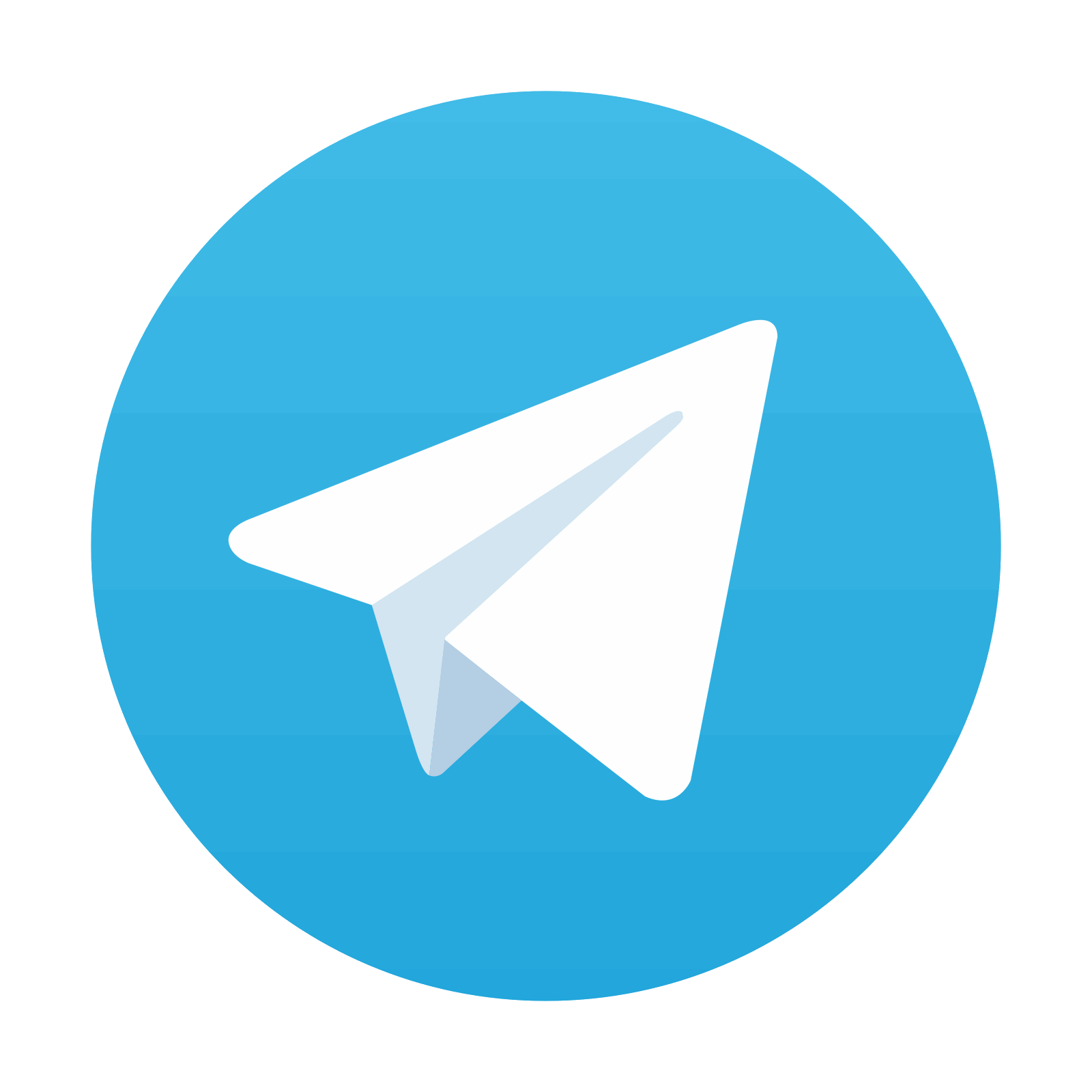
Stay updated, free articles. Join our Telegram channel

Full access? Get Clinical Tree
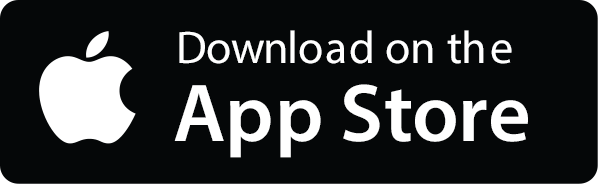
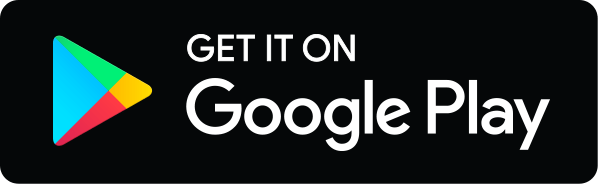
